Abstract
Surface enhancement of engineering materials is an essential step in improving the service life, mechanical properties and corrosion resistance of any metal. Behavioural effects of SiO2 particulate on the electrodeposition of Zn-Nb2O5 coatings of mild steel were investigated. Bath composition was developed through zinc electrolyte solution to produce ZnNb2O5–SiO2 alloy. The adhesion profile, topography and microstructures of the coated samples were analysed using atomic force micrographs (AFM), and JEOL JSM 6390 scanning electron microscope (SEM) coupled with energy dispersive x-ray analyser (EDS micrographs). Emco-test microhardness tester and MTR 300 dry abrasion rig machines were used for the determination of hardness and wear resistance of the coated samples. Results of the research showed that finer microstructure of the Zn-Nb2O5–SiO2 composites obtained was due majorly to the addition of SiO2 particulate. Also, hardness behaviour and wear resistance of Zn-10Nb2O5 coating increased due to incremental addition of SiO2 particulate (up to 10 wt%).
PUBLIC INTEREST STATEMENT
Surface engineering that is tailored towards coating and modification of metal surfaces is becoming increasingly important because it allows both the physical and mechanical properties of the materials to remain unchanged while at the same time the corrosion resistance is improved upon. That is, surface enhancement of engineering materials is an essential step in improving the service life of any metal. Behavioural effects of SiO2 particulate on the electrodeposition of Zn-Nb2O5 coatings of mild steel were investigated. The microstructures of the coated samples were analysed using atomic force micrographs (AFM), scanning electron microscope (SEM). The determination of hardness and wear resistance of the coated samples was done. The finer microstructure of the Zn-Nb2O5–SiO2 composites obtained was as a result of the addition of SiO2 particulate to the composites. Both the hardness performance and wear resistance of Zn-10Nb2O5 coating increased due to incremental addition of SiO2 particulate.
1. Introduction
It is an established fact that surface engineering that is tailored towards the coating and modification of metal surfaces is becoming increasingly important because it allows both the physical and mechanical properties of the materials to remain unchanged while at the same time the corrosion resistance is improved (Basavanna & Arthoba, Citation2014; Popoola, Aigbodion, & Fayomi, Citation2016a). Zinc and its alloys, due to their better corrosion resistance property, are commonly used in steel coatings. Although excellent in this mode of protection, but these coatings are often less durable when subjected to environments of combined wear and corrosion due to their intrinsic relative softness and ductility (Popoola, Aigbodion, & Fayomi, Citation2016b; Popoola & Fayomi, Citation2012). One good way of combating the durability challenge of these coatings is through co-deposition of inert particles on the zinc and zinc-alloy matrix (Popoola et al., Citation2016b; Tuaweri, Adigio, & Jombo, Citation2013).
Many research works have been performed on the study of the behavioural pattern of the deposition process of Zn composite. It is found that the characteristics of the deposited coating depend on the applied voltage, current density, pH, bath composition, additives and temperature (Blejan & Muresan, Citation2012; Fayomi, Abdulwahab, & Popoola, Citation2013; Patel et al., Citation2013; Refaey & Tillmann, Citation2008; Tiwari, Sahu, Pramanick, & Raghuvir, Citation2011). The microstructure of the surface of the deposited Zn composite is another important characteristic which affects the corrosion resistance and other mechanical properties (Ayoola, Efeovbokhan, Bafuwa, & David, Citation2014; Fullston, Citation2004; Mohankumar, Praveen, Venkatesha, Vathsala, & Nayana, Citation2012; Olafadehan, Ayoola, Akintunde, & Adeniyi, Citation2015).
Co-deposition of zinc metal matrix with ZnO composite using electrolytic chloride based coating consisting of 20–40 g/L ZnO particle was reported by (Popoola & Fayomi, Citation2012). The composite coatings were characterized using high optic microscope (OPM). The corrosion resistance properties of Zn-ZnO composite coatings were measured using linear polarization in 3.5% NaCl solution. The results showed that addition of ZnO particles in the deposition bath obviously increased hardness values and corrosion resistance significantly.
According to (Ayoola, Fayomi, Popoola, & Oloruntoba, Citation2017), composite coating of Zinc-TiO2 on mild steel was achieved by preparing bath solution using varied concentration of TiO2. And the results of the corrosion behaviour by electrochemical studies using polarization and Impedance method revealed that TiO2 incorporated with the Zinc coatings produced better corrosion resistance towards aggressive media when compared to pure zinc coating. The embedded TiO2 nanoparticles changed the compactness, microstructure and orientation of the deposit.
Hence, inert materials are to be included in order to improve mechanical properties, corrosion resistance properties and life span of the zinc coating. That is, the inclusion of niobium oxide and silica in zinc coating, by co-deposition of these materials with zinc, needs to be investigated. This research work is to investigate the effect of varied concentration of silica dioxide on the electrodeposition of Zn coated mild steel, with focus on wear, hardness and corrosion resistance properties.
2. Materials and methods
2.1. Materials and reagents
The materials and reagents used include a flat plate mild steel (30 mm × 30 mm) sample, zinc-niobium oxide-silica composite electrodeposit produced from chloride bath (Tables and ).
Table 1. Chemical composition and concentration for bath solution
Table 2. Chemical composition of Zn-10Nb2O5-SiO2 matrix
2.2. Preparation of the coatings
The electrodeposition bath was prepared from analar grade chemicals and deionized water. Mild steel sample was dipped into the acid solution for 10 s, and then rinsed with the deionised water. The solution was continuously stirred with the aid of magnetic stirrer for the solution to plate well on the steel. 99% pure zinc (anode) and mild steel (cathode) were connected to DC rectifier, through copper wire. The electrodeposition of the samples was done for 15 min at different concentration of SiO2 additive.
2.3. Characterization of the electrodeposited samples
After electrodeposition, the adhesion profile, topography and microstructures of the samples were carried out using atomic force micrographs (AFM), and JEOL JSM 6390 scanning electron microscope (SEM) coupled with energy dispersive x-ray analyser (EDS micrographs). Also, the optical micrographs of the samples’ microstructure were done after heat treatment of the samples and after corrosion.
2.4. Hardness test
Emco-test microhardness tester machine was used to evaluate and display hardness value of each of the samples on the computer screen connected to the machine. Indentations of four (4) points for 20 s with a load of 100 g were made on the surface of each sample.
2.5. Wear test
Mass loss by samples due to wear was determined using MTR 300 dry abrasion rig machine, in a dry medium of silica sand at speed of 200 rev/min for 60 s. The difference in mass before and after the tests was noted after each dry sliding.
3. Results and discussion
Figures – show SEM/EDS analysis of Zn-10Nb2O5 composites. EDS result in Figure indicates that is no presence of Si, while the presence of Si peak in the Zn-10Nb2O5-SiO2 coatings can be verified in Figures and . Comparing Figures and , it was obvious that the microstructure of Zn-10Nb2O5-10SiO2 coating was more coagulated than that of Zn-10Nb2O5-5SiO2 sample. That is, a more adhesive crystal of Zn-10Nb2O5-10SiO2 made the coating to be tightly packed to mild steel. And this behaviour promotes improved corrosion resistance and mechanical properties of the material. That is, an increase SiO2 coating concentration favours anticorrosion behaviour of the coated mild steel (Ayoola, Anawe, Efeovbokhan, & Akpanobong, Citation2014; Ayoola et al., Citation2017; Badkar, Pandey, & Buvanashekaran, Citation2009).
Figures – reveal AFM topography of Zn-10Nb2O5, Zn-10Nb2O5-5SiO2 and Zn-10Nb2O5-10SiO2 matrix (respectively) in two (2D) and three (3D) dimensions. The plane surface observed in Figure indicates the absence of SiO2. The uniformity of the coating was better achieved in Figure than Figure . That is, the topography is more uniform in Figure as a result of the formation of fine structure and sub-grains.
To further evaluate the quality of composite coating obtained, optical microscope (X100 magnification) was used to study the microstructures of the samples after electrodeposition (Figure ), after heat treatment (Figure ) and after corrosion (Figure ). The results show that as the quantity of SiO2 added in the formulation increased, the micro grains become more finely and uniformly distributed on the surface. Considering Figure , it was observed that many pits were noticed in Figure (a), lesser pits were noticed in Figure (b), while the least number of pits were seen in Figure ). This implies that increase in SiO2 added during electrodeposition resulted in improved and refined grains distribution. This claim supports the results obtained from SEM/EDS and AFM analyses.
Figure 7. Optical micrographs obtained (a) Zn-10Nb2O5, (b) Zn-10Nb2O5-5SiO2, (c) Zn-10Nb2O5-10SiO2 after electrodeposition.
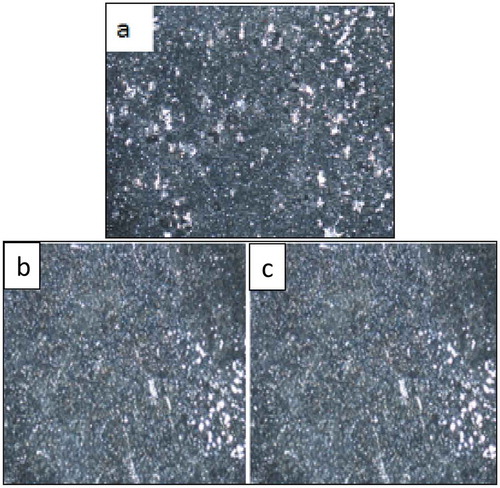
Figure 8. Optical micrographs obtained (a) Zn-10Nb2O5 (b) Zn-10Nb2O5-5SiO2 (c) Zn-10Nb2O5-10SiO2 after heat treatment.
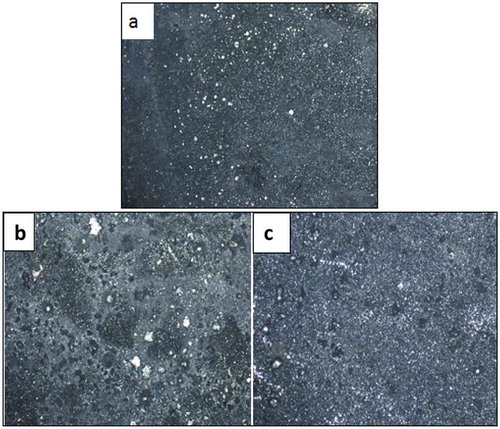
Figure 9. Optical micrographs obtained: (a) Zn-10Nb2O5, (b) Zn-10Nb2O5-5SiO2, (c) Zn-10Nb2O5-10SiO2 after corrosion.
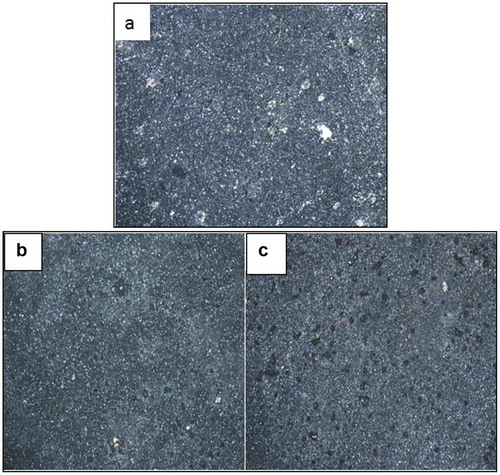
Similar trend was observed in the optical microscopic examination of the coated samples after heat treatment (Figure ) and after corrosion (Figure ). That is, increase in SiO2 added (up to 10 wt% of SiO2) during heat treatment, as well as after corrosion, resulted in improved and refined grains distribution. It is reasonable to state that the heat treatment promotes the firm adhesion of the coating material to the surface of the steel and that the addition of SiO2 nanoparticle up to 10 wt% improves the corrosion resistance property of the sample (Ayoola, Fayomi, Popoola, & Ige, Citation2017).
In Figure show the hardness values obtained from the coated samples, before and after heat treatment. The results show that the hardness of the samples increased as the concentration of SiO2 in the coating formulation increased. For instance, Zn-10Nb2O5 coated sample had 132 and 138 HV (before and after heat treatment respectively) while Zn-10Nb2O5-10SiO2 coated sample had 138 and 147 HV (before and after heat treatment respectively). This could be attributed to the higher hardness of SiO2 ceramic coating compared to mild steel. Similar result was reported by (Mohankumar et al., Citation2012; Popoola et al., Citation2016b).
Likewise the results show that heat treatment enhances the hardness property of the samples. This is due to the fact that the hardness values obtained after heat treatment were higher than the results obtained before heat treatment. According to (Rana, Goswami, Jha, Mishra, & Prasad, Citation2007), the addition of heat strengthens the intermolecular bonds within the grains and at the boundary of the ceramic particles with the substrate (Popoola et al., Citation2016b).
From Figure , coated samples exhibited great wear abrasion resistance. The wear rate of Zn-10Nb2O5 sample (without SiO2) was significantly high. From the results, one can also infer that the load-bearing potential of the samples increases as the concentration of SiO2 in the coating formulation increased. The wear rate of Zn-10Nb2O5-10SiO2-coated sample was 0.004 g/min, the wear rate of Zn-10Nb2O5-5SiO2-coated sample was 0.006 g/min while 0.009 g/min was the wear rate of Zn-10Nb2O5-coated sample. The results show that SiO2 nanoparticle possesses great inherent strength to withstand stress, particularly at a concentration of 10 g/L.
4. Conclusion
Based on the methods adopted, results obtained and the discussion of the results, the following facts are clearly made:
Zn-10Nb2O5-SiO2 coatings displayed better adhesion property compared to Zn-10Nb2O5 coating or mild steel (control).
Hardness property of Zn-10Nb2O5 coating increased due to incremental addition of SiO2 particulate (up to 10 wt%).
Wear abrasion resistance of Zn-10Nb2O5 coating increased due to incremental addition of SiO2 particulate (up to 10 wt%).
The finer microstructure formed due to the addition of SiO2 contributed greatly to improvement in the hardness behaviour and wear resistance.
Acknowledgements
This work received support from surface engineering research centre, TUT South Africa and advance surface and electrochemistry centre (ASEC) in the department of Mechanical Engineering, Covenant University, Ota, Nigeria. The open access financial contribution of Covenant University CUCRID is highly appreciated.
Additional information
Funding
Notes on contributors
A. A. Ayoola
A. A. Ayoola holds a PhD degree in Chemical Engineering. He is a senior lecturer in the Department of Chemical Engineering, Covenant University, Ota, Nigeria. His research interest includes surface engineering, corrosion technology and biofuel (renewable) energy.
O. S. I. Fayomi
O. S. I. Fayomi is a senior researcher in the Department of Mechanical Engineering at Covenant University and Research Fellow with Surface Engineering Research Centre (SERC) Tshwane University of Technology, Pretoria, South Africa. His research interests are centred on surface structural integrity, corrosion engineering, mechanical metallurgy, environmental science and engineering, and triboxidation processing.
A. P. I. Popoola
A.P.I Popoola is a professor of Metallurgical Engineering in the Department of Chemical, Metallurgical and Materials Engineering at Tshwane University of Technology, Pretoria, South Africa. She is the leader of Advanced Engineering Materials and Surface Technologies unit and her research includes surface science and corrosion engineering among others.
References
- Ayoola, A. A. , Anawe, P. A. L. , Efeovbokhan, V. E. , & Akpanobong, O. (2014). Investigating alternatives to diesel in oil based drilling fluid formulations used in the oil industry. Journal of Environmental Engineering and Science , 4(14), 70.
- Ayoola, A. A. , Efeovbokhan, V. C. , Bafuwa, O. T. , & David, O. T. (2014). A search for alternative solvent to hexane during neem oil extraction. International Journal Sc & Technical , 4, 66.
- Ayoola, A. A. , Fayomi, O. S. I. , Popoola, A. P. I. , & Ige, O. O. (2017). Study of particle incorporation and performance characteristic of aluminium silicate-zirconia embedded on zinc rich coatings for corrosion and wear performance. Asian Journal Chemical , 29(12), 2575. doi:10.14233/ajchem
- Ayoola, A. A. , Fayomi, O. S. I. , Popoola, A. P. I. , & Oloruntoba, T. (2017). Inhibitive characteristics of cetylpyridinium chloride and potassium chromate addition on type A513 mild steel in acid/chloride media. Cogent Engineering , 4, 1.
- Badkar, D. S. , Pandey, K. S. , & Buvanashekaran, G. (2009). Laser transformation hardening of unalloyed titanium using Nd: YAGlaser. International Journal Materials Sciences , 4(3), 239.
- Basavanna, S. , & Arthoba, N. Y. (2014). Electrochemical studies of Zn–Ni alloy coatings from acid chloride bath. Journal Applications Electrochemistry , 39(10), 1975. doi:10.1007/s10800-009-9907-1
- Blejan, D. , & Muresan, L. M. (2012). Corrosion behaviour of Zn–Ni–Al2O3 nanocomposite coatings obtained by electrodeposition from alkaline electrolytes. Materials Corrosion , 63, 9999.
- Fayomi, O. S. I. , Abdulwahab, M. , & Popoola, A. P. I. (2013). Properties evaluation of ternary surfactant-induced Zn-Ni-Al2O3 films on mild steel by electrolytic chemical deposition. Journal Ovonic Researcher , 9, 123.
- Fullston, D. (2004). Zinc coating thickness effects on hot dip galvanized steel corrosion rates at a severe marine site. Corrosion Materials , 29, 165.
- Mohankumar, C. , Praveen, K. , Venkatesha, V. , Vathsala, K. , & Nayana, O. (2012). Electrodeposition and corrosion behaviour of Zn–Ni and Zn–Ni–Fe2O3 coatings. Journal of Coatings Technology and Research , 9(1), 71. doi:10.1007/s11998-011-9322-5
- Olafadehan, O. A. , Ayoola, A. A. , Akintunde, O. O. , & Adeniyi, V. O. (2015). Mechanistic kinetic models for steam reforming of concentrated crude ethanol on Ni/Al2O3 catalyst. Journal Engineering Sc & Technical , 10, 633.
- Patel, N. S. , Jauhariand, S. , Mehta, G. N. , Al-Deyab, S. S. , Warad, I. , & Hammoouti, B. (2013). Mild steel corrosion inhibition by various plant extract in 0.5M sulphuric acid. International Journal Electrochem Sciences , 8, 2635.
- Popoola, A. P. I. , Aigbodion, V. S. , & Fayomi, O. S. I. (2016a). Anti–Corrosion coating of mild steel using ternary Zn–ZnO–Y2O3 electro–Deposition. Surface and Coatings Technology , 306, 448. doi:10.1016/j.surfcoat.2016.05.018
- Popoola, A. P. I. , Aigbodion, V. S. , & Fayomi, O. S. I. (2016b). Surface characterization, mechanical properties and corrosion behaviour of ternary based Zn–ZnO–SiO2 composite coating of mild steel. Journal of Alloys and Compounds , 654, 561. doi:10.1016/j.jallcom.2015.09.090
- Popoola, A. P. I. , & Fayomi, O. S. I. (2012). Performance evaluation of zinc deposited mild steel in chloride medium. International Journal Electrochem Sciences , 6, 3254.
- Rana, G. L. , Goswami, S. K. , Jha, P. K. , Mishra, P. K. , & Prasad, S. S. (2007). Experimental studies on the microstructure and hardness of laser-treated steel specimens. Optics & Laser Technology , 39(2), 385. doi:10.1016/j.optlastec.2005.07.001
- Refaey, A. E. , & Tillmann, W. (2008). Characterization of titanium/steel join brazed in vacuum. Journal Weld Researcher Council , 87, 113.
- Tiwari, S. K. , Sahu, R. K. , Pramanick, A. K. , & Raghuvir, S. (2011). Development of conversion coating on mild steel prior to sol gel nanostructured Al2O3 coating for enhancement of corrosion resistance. Surface and Coatings Technology , 205, 4960. doi:10.1016/j.surfcoat.2011.04.087
- Tuaweri, T. J. , Adigio, E. M. , & Jombo, P. P. (2013). A study of process parameters for zinc electrodeposition from a sulphate bath. International Journal of Engineering Science , 2, 17.