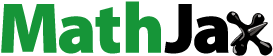
Abstract
In Vietnam, the fire protection for steel structures is relativity much; thus, the study of fire protection solutions for the load-bearing steel structures (beams, columns) is considered very important in the safety of the buildings. One possible solution is the use of gypsum plasterboard. Up to now, the engineers use the constant thermal conductivity to verify the fire resistance of steel structures protected by gypsum plasterboard. However, the thermal conductivity depends on many factors such as temperature, moisture, thickness, type of plasterboard, manufacturer, heating rate. This paper presents an experimental identification of the thermal conductivity of gypsum plasterboard fabricated under fire conditions.
PUBLIC INTEREST STATEMENT
This study investigates different types of fire protection for steel structures. It describes the experimental results obtained for the thermal conductivity of gypsum plasterboard used in the protection of steel columns in Vietnam. Thermal conductivity was determined through a one-sided heat transfer experiment, taking parameters such as moisture, thickness, type of plasterboard, and heating rate into account. It was found that thin sheets used to protect structures under fire action are more effective than thicker sheets. The thermal conductivity determined from the experiment will be used in the actual calculation of the fire resistance of steel columns protected by gypsum plasterboard.
Competing Interests
The authors declare no competing interest.
1. Introduction
Steel structures have been popularly used throughout the world and increasingly used in many projects in Vietnam. Besides the many advantages of steel structures, one of its inconvenient is the low fire resistance. Thus, the study of fire protection solutions for the load-bearing steel structures (beams, columns) is considered very important in the safety of the buildings. One possible solution is the use of gypsum plasterboard.
The studies on the behavior of steel columns protected by box-type coating gypsum plasterboard have also been mentioned in a number of studies in the country and the world. However, these research works have not yet mentioned the impact of the actual moisture in the gypsum on its insulation capacity when the fire occurs. The calculation of the protective capability of the fireproof gypsum plasterboard was based on the thermal conductivity of gypsum as a constant with a dehydrated gypsum plasterboard provided by the manufacturer, while at the moment of a fire occurring, there still exists an amount of water due to the humid air. Impacts of heat during the combustion process will make this water dehydrated, causing the thermal conductivity to change. Therefore, the timing of insulation for the protected structures when using the values of the changing thermal conductivity mentioning the effects of dehydration process will provide results close to reality, ensuring the safety for the structures.
This paper first presents the previous studies on the experimental identification of the thermal conductivity of gypsum plasterboard and then an experimental investigation on the parameter influencing the thermal conductivity of gypsum plasterboard heated on one side under fire conditions.
2. Previous studies
Plasterboard is made mainly of plaster, with the chemical formula is CaSO4. 2H2O. The composition of gypsum plasterboard consists of gypsum covered by two thin layers of cardboard paper, about 0.3 mm, on either side of the face. In some special cases, such as fire gypsum plasterboard, the gypsum core is reinforced with fiberglass, vermiculite, and clay. Fiberglass helps keep the mechanical stabilizer while vermiculite and clay are added to help reduce the shrinkage of the plasterboard when subjected to high temperatures (Thomas, Citation2002, Citation2010). The characteristics of gypsum plasterboard under the effect of high temperature are described below:
In the temperature range from 30°C to 200°C: When heating the whole face of the panel already hardened, over 70°C, the water separation occurred, which destroyed the crystal lattice structure. At the temperature of approximately 200°C, the chemical water in the gypsum is completely separated. At the temperature of 200°C, the weight loss of sample is the largest (approximately 21%) due to the complete dehydration of gypsum.
(1)
(1)
(2)
(2)
In the temperature range of 400–700°C: The panel has been completely dehydrated in the previous temperature range. So at this range of temperature, the weight of the sample does not change.
From 900°C: If the temperature continues to rise to nearly 900°C, the following chemical reaction occurs:
(3)
(3)
The thermal conductivity of the plasterboard is affected by several factors such as temperature, density, water quantity, porosity of the material, and the appearance of cracks in the plasterboard when exposed to fire. All measurements of thermal conductivity in the literature are performed in a stabilized condition that did not take into account the movement of moisture and the opening of cracks (Tran, Citation2012).
Mehaffey, Cuerrier, & Carisse (Citation1994) proposed the variation of the thermal conductivity in two regions: a linear decrease up to 200°C and a linear increase between 200°C and 1,000°C, while Sultan (Citation1996) indicated that the thermal conductivity of the plasterboard can be defined by four regions: a constant value up to 100°C, at 100°C the value decreases and remains constant up to 400°C, then gradually increases with two positive slopes between 400°C and 800°C and after 800°C.
Tests conducted by Benichou (Citation2005) have shown that the thermal conductivity can be defined by three regions: a linear decrease up to 200°C, a constant value between 200°C and 800°C, and a sharp increase after 800°C.
Ghazi Wakili, Hugi, Wullschleger, and Frank (Citation2007), meanwhile, measured thermal conductivity with three types of specimens from the same plasterboard. The first was untreated, the second was exposed at 200°C for 2 days to complete the evaporation of water, and the third was exposed to standard fire according to ISO 834 (Citation2014) for 45 minutes. The thermal conductivity for the untreated test piece is 0.28 W/mK, 0.14 W/mK for the specimen exposed at 200°C, and 0.27 W/mK for the specimen exposed to normalized fire. Based on the measured results, the authors assumed that the conductivity did not change between the reactions (i.e., between 200°C and 700°C) and they proposed the variation of the thermal conductivity according to three regions based on three measured values.
Rahmanian and Wang (Citation2012) and Ranawaka and Mahendran (Citation2009) presented the “hybrid” method, based on experimental tests and the thermal conductivity model incorporating the effect of radiation in pores, to determine the effective thermal conductivity of gypsum plasterboard in function of temperature. The thermal conductivity proposed by the author is divided into three regions: a constant value between 20°C and 95°C corresponding to the state before the evaporation of free water and chemically bonded, a linear reduction due to evaporation water up to 155°C, and a nonlinear increase.
The values of thermal conductivity reported by various authors in the literature are shown in Figure . It can be seen that there is a significant dispersion from 500°C. This dispersion could be related to the difference of the plasterboard studied and the heating rate.
3. The experimental identification for thermal conductivity of gypsum plasterboard under fire conditions
The thermal conductivity of the gypsum plasterboard, used in protecting steel structures under fire conditions, was determined by heating the plasterboard on one side in an electric furnace. This is similar to the case when the gypsum plaster board is covered up partly by steel structures and exposed on one side.
The temperature at the hot side is heated (in the furnace) as the heating curve in ISO 834.
The samples were placed in front of the furnace and the inner side of the panel directly contacting the heat source (hot side). This heat source can be adjusted to maintain a certain stable heating temperature. The location of contact between the sample and the furnace was inserted with a sealed insulation material, ensuring the heat in the furnace stays packed in. The heat transfer in the panel was assumed to be unidirectional, stable at the cross section of the panel and considered as homogeneous isotropic material. The temperature at the surface of the panel exposed to heat (the hot side) and the outer surface (cold side) was measured using temperature probes. Temperatures in the outer panels were monitored until stabilized and thereby determining the necessary values and calculating the corresponding thermal conductivity follow EquationEquation (4)(4)
(4) . Electric furnace was located in the air-conditioned room with temperature outside the furnace kept stable.
The thermal conductivity was determined using the following formula:
where I is the intensity of electricity provided for the furnace, A; U is the voltage provided for the furnace, V; A is the contact area of the sample with the furnace, is the thickness of the sample, m; T1 is temperature contacting heat, °C; T2 is the temperature contacting the environment, °C.
In the above formula, heat transferred from the hot surface to cold surface was assumed to be linear allocation based on the thickness of panels.
The investigation of thermal conductivity of gypsum plasterboard heated on one side is described in the following paragraph:
Laboratory equipment used are as follows: electric furnaces ELF 11/4 model, with a temperature range from 0°C to 1,100°C; Model 191-WRNK temperature probe—and the thermometer Model XMTD—2001. Samples include fireproof gypsum plasterboard manufactured by GYPROC with different thickness—12.7 and 15.8 mm. The size of the samples is 300 × 300 mm2.
Samples are heated at the hot surface at various temperature levels: 50°C, 100°C, 120°C, 200°C, 400°C, 600°C, 800°C, 1,000°C. The temperature values were chosen based on the changing behavior of gypsum as analyzed in the previous paragraph. At each temperature level, three samples were experimented. The experimental procedure and temperature measurement locations were arranged as follows:
Inside the furnace: one measuring point (point 1)
On the outer surface of the panel: one measuring point (point 3)
In the middle of the panel: one measuring point (point 2)
The order of testing the temperature at each level is as follows:
The sample is weighed prior to testing (volume m1 is recored);
The sample is jigged in front of the furnace;
The probes are installed;
The temperature in the furnace is increased to the temperature level experiments (recording time);
When the furnace temperature reaches the temperature level experiments, the temperature at the cold surface is monitored until it has stabilized; and temperature values measured at three points 1, 2, 3 have a linear relationship.
After the temperature has stabilized, the temperature, intensity, voltage, and values of weight m2 are recored.
3.1. Effects of moisture loss
To determine the effects of moisture loss, the effects of moisture loss shall be investigated by one- sided heating method.
Figure presents the measured temperatures of 15.8 mm sample when the temperature level was 200°C at the hot side. At this temperature level, the time needed to obtain a stable temperature at mid-thicknesses and at cold side of the sample was 17 minutes.
The other results at different temperature levels show that the time needed to obtain a stable temperature in the sample is from 15 to 20 minutes (Table ).
Table 1. The time to reach a stable temperature with 12.7 and 15.8 mm samples
From the results in Tables , and Figure , one can give the conclusion as follows:
(1) From 200°C, the thermal conductivity is virtually unchanged and is considered as constant;
(2) With the studied gypsum plasterboard, at a temperature level, the time to reach a stable value was in the range of 15–20 minutes. This steady state corresponds to the total dehydration of free water and chemical water in gypsum plasterboard (within the region in which the temperature was greater than 200°C) according to EquationEquations (1(1)
(1) ) and (Equation2
(2)
(2) ).
The moisture loss of 12.7- and 15.8-mm gypsum plasterboard with temperatures at hot side, mid-thicknesses and at cold side are summarized in Tables and , respectively.
Table 2. Moisture loss of 12.7 mm sample with temperature at different points
Table 3. Moisture loss of 15.8 mm sample with temperature at different points
Table 4. Thermal conductivity of GYPROC
Figure shows the influence of the moisture loss on the thermal conductivity of 12.7- and of 15.8-mm gypsum plasterboard.
Figure 4. Comparison of the thermal conductivity of the two GYPROC FIREBLOC gypsum plasterboards experimented with foreign research findings
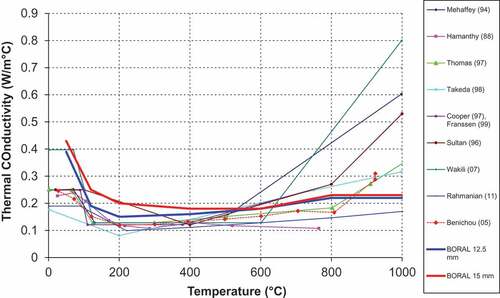
Figure 5. Comparison of temperature of GYPROC gypsum plasterboard with different heating rates and standard fire curve ISO 834
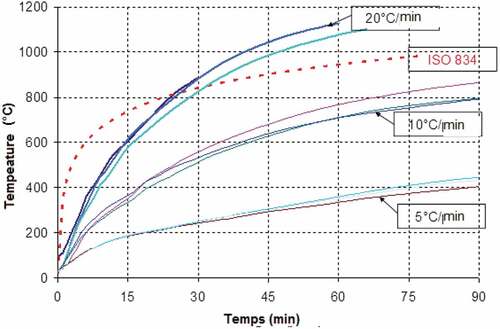
Figure 6. Comparison of thermal conductivity of GYPROC gypsum plasterboard with different heating rates (20°C/min, 10°C/min, 5°C/min)
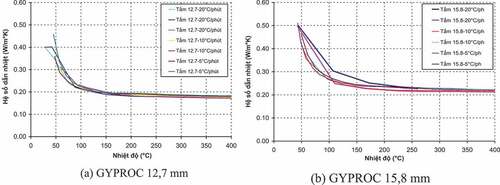
From Tables , and Figure , the following conclusions were drawn:
(1) Influence of the moisture loss to thermal conductivity of gypsum plasterboard with two different thicknesses was similar; (2) the moisture loss was not the same between the 12.7 and 15.8 mm samples (e.g., with 12.7 mm samples, the moisture loss reached 20% when the temperature of hot side was 1,000°C. While the moisture loss of 15.8 mm samples reached 15% when the temperature of hot side was 1,000°C). Thus, the thermal conductivity of 12.7 mm sample is smaller than the coefficient of 15.8 mm sample. This difference can be explained by the temperature distribution which is not uniform through the thickness of the samples and affects the dehydration rate.
3.2. Effect of thickness
Experiments tests were conducted with 2 thickness of Gyproc Panel in order to determine their thermal conductivity. The values of the thermal conductivity calculated from the experimental measurements were given in the Table :
In the Figure , from the heating degree to temperatures of 200°C or higher, the value of thermal conductivity of two panels virtually unchanged and was regarded as constant; the law of the influence of the water loss to the thermal conductivity as for two gypsum plasterboards with two different thicknesses was similar; at the same heat level, the value of water loss of thin and thick panel was not the same (e.g., 12.7 mm GYPROC panel with total complete dehydration at about 20% when the temperature at the hot surface was 1,000°C, while the 15.8 mm GYPROC panels have a total complete dehydration at 15% when the hot surface temperature was 1,000°C) and the value of the thermal conductivity of the thin panel was smaller than the thick one. This can be interpreted as thinner gypsum plasterboard will dehydrate much more and therefore it has a smaller thermal conductivity. Thus, with a certain thickness, the thin panels used to protect the structures under the effect of the fire will be more effective if a sheet with a equivalent thickness is used.
3.3. Effect of heating rate
For each type of fires, the heating rate usually follows a certain law. To examine the impact of this parameter, the experimental tests were conducted in the condition that the furnace temperature was continuously increased as in three heating curves, at the rate of 20°C/min, 10°C/min, and 5°C/min, respectively (Figure ). At each heating rate, three samples for experiments were taken. The experimental results in Figure showed that (1) the effect of heating rate on the thermal conductivity of thin panels was smaller than that of thicker ones; (2) the effect of heating rate on the thermal conductivity of the experimental panels of different thickness mainly ranging from 30°C to 200°C, and within this temperature range, high heating rate (20°C/min) had a greater impact on the thermal conductivity in comparison with lower heating rate; (3) with the two experimental panels, the highest heating rate (20°C/min) gives the largest thermal conductivity.
From the above results, it is found that there is a need to determine the thermal conductivity for each specific type of panel. In favor of safety, experiments can be conducted for the largest panels and heat transfer at 20°C/min to obtain the thermal conductivity and calculate the most unfavorable results.
4. Conclusion
In this paper, an experimental procedure has been developed to define the thermal conductivity of gypsum plasterboard matching the hot and humid climate conditions of Vietnam and the actual impact of the fire, namely
With two types of gypsum plasterboard studied, the shapes of the thermal conductivity curves are similar between the samples;
From 600°C to 1,000°C, the thermal conductivity is not increased linearly for some references. This demonstrates the quality of the two samples is good and can be used to protect steel structures under fire conditions with temperatures up to 1,000°C;
The thermal conductivity of 12.7 mm sample is smaller than the coefficient of 15.8 mm sample. Thus, with a certain thickness, the thin plates used to protect steel structures in fire conditions are better than using a plate which has a thickness equivalent;
From 200°C, the thermal conductivity is virtually unchanged and is considered as a constant. With the gypsum plasterboard studied, at a temperature level, the time to reach a stable value was in the range of 15–20 minutes. This steady state corresponds to the total dehydration of free water and chemical water in gypsum plasterboard (within the region in which the temperature was greater than 200°C);
With a temperature level, the moisture loss was not the same between the 12.7 and 15.8 mm samples. Moreover, the thermal conductivity of 12.7 mm sample is smaller than the coefficient of 15.8 mm sample;
The influence of heating rate on the thermal conductivity with the 12.7 mm samples is relatively small in comparison with the 15.8 mm samples. The influence of heating rate is more evident from 30°C to 200°C, and in this range of temperature, the thermal conductivity corresponding to the highest heating rate (20°C/min) is greater than the coefficient in the lower heating rate.
In the next stage, the experimental thermal conductivity will be used in the numerical model to simulate the fire behavior of the steel column protected by gypsum plasterboard.
correction
This article was originally published with errors, which have now been corrected in the online version. Please see Correction (http://dx.doi.org/10.1080/23311916.2020.1787692)
Additional information
Funding
Notes on contributors
Nguyen Vo Thong
NGUYEN Vo Thong 22 November 1955 Associate Professor, Senior Researcher, Vietnam Institute for Building Science and Technology (IBST)
Main research directions: Structural behavior under extreme load (wind, storm, earthquake, fire) and structural design calculation;
Results of training and scientific research:
Guided three PhD students and they successfully defended their doctoral dissertation;
Headed 26 scientific research subjects;
Published 54 scientific articles in journals, in the proceeding of scientific conferences in Vietnam and other countries;
The lead author of 15 books and national standards on structure (in Vietnamese).
References
- Benichou, N. (2005). Thermal properties of lightweight-framed construction components at elevated temperatures. Fire and Materials, 29(3), 165–10. doi:10.1002/fam.880
- BS ISO 834-11:2014 Fire resistance tests. Elements of building construction. Specific requirements for the assessment of fire protection to structural steel elements.
- Ghazi Wakili, K., Hugi, E., Wullschleger, L., & Frank, T. H. (2007). Gypsum board in fire—Modeling and experimental validation. Journal of Fire Sciences, 25(3), 267–282. doi:10.1177/0734904107072883
- Mehaffey, J. R., Cuerrier, P., & Carisse, G. (1994). A model for predicting heat transfer through gypsum-board/wood-stud walls exposed to fire. Fire and Materials, 18(5), 297–305. doi:10.1002/(ISSN)1099-1018
- Rahmanian, I., & Wang, Y. C. (2012). A combined experimental and numerical method for extracting temperature-dependent thermal conductivity of gypsum boards. Construction and Building Materials.
- Ranawaka, T., & Mahendran, M. (2009). Experimental study of the mechanical properties of light gauge cold-formed steels at elevated temperatures. Fire Safety Journal, 44(2), 219–229. doi:10.1016/j.firesaf.2008.06.006
- Sultan, M. A. (1996). A model for predicting heat transfer through non-insulated unloaded steel-stud gypsum board wall assemblies exposed to fire. Fire Technology, 32(3), 239–259. doi:10.1007/BF01040217
- Thomas, G. (2002). Thermal properties of gypsum plasterboard at high temperatures. Fire and Materials, 26(1), 37–45. doi:10.1002/(ISSN)1099-1018
- Thomas, G. (2010). Modelling thermal performance of gypsum plasterboard-lined light timber frame walls using SAFIR and TASEF. Fire and Materials, 34(8), 385–406. doi:10.1002/fam.v34:8
- Tran, H. (2012). Numerical and experimental study of behavior of gypsum plasterboard separating elements under fire conditions (in French). Ecole normal superior Cachan.