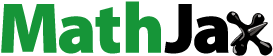
Abstract
The present paper describes the Sustainable Water Management Index, SWaM_Index, useful for managers to measure the sustainability of water management and to assess the effects of the policies undertaken in the direction of sustainability. The structure of the proposed index has been derived from European legal framework of the water resources sector, and the index is obtained from elementary indicators of environmental, economic, social and institutional nature, subsequently aggregated in sub-themes, themes and pillars. The index is well applicable on a national, regional but also local scale and the sustainable management of water resources is assessed considering natural systems, artificial systems and socio-economic and institutional aspects.
PUBLIC INTEREST STATEMENT
This paper deals with the sustainability of water management and proposes an index useful to managers to make sustainability estimates and measure of the effectiveness of the sustainability policies adopted.
The proposed index refers to the EU directives and takes into consideration the natural systems, the artificial systems and socio-economic and institutional aspects. The index reduces the analyst’s subjectivity since the measurement unit of the single indicators is given by its distance to the corresponding target, being the latter properly and objectively fixed according to the European legal frameworks of the sector.
The index is well applicable on a national, regional but also local scale.
The proposed index has been conceived and derived from proper legal framework on water resources. In this work, reference has been made to EU legislation, but it may also be adapted to different regulatory contexts.
1. Introduction
The concept of sustainability is now part of the daily technical and socio-economic discussion, not just scientific. Studies are needed to quantify and measure it. Sustainability estimation procedures and methodologies would make it possible to translate today’s universally recognized priority objective into concrete applications.
The World Commission on Environment and Development report “Our common future”—the Brundtland report—defines sustainable development as something that meets the needs of the present without compromising the ability of future generation to meet their own needs (World Commission on Environmental and Development Our Common Future [WCED], Citation1997). This has stimulated activity and discussion in many sectors about the need to achieve a balance between environment, society and economy. Several studies have been aimed at implementing a more specific definition of sustainability, and its quantification and measurement.
Differences in disciplinary perspectives, and in the philosophical and ethical interpretation of sustainable development, have resulted in sustainability concepts giving priority to either economic or environmental objectives—e.g. the opposing paradigms of “weak” and “strong” sustainability (Hediger, Citation2006). The paradigm of weak sustainability considers that natural capital can be substituted by anthropogenic capital, while the strong sustainability approach is based on an ecological-economic framework and argues that many of the most fundamental services provided by nature cannot be replaced by services produced by human or man-made capital (Ayres, Citation2007; Costanza, Cumberland, Daly, Goodlan, & Norgaard, Citation1997; Daly, Citation1991; Hediger, Citation1999; Neumayer, Citation2003).
The concept of sustainable development is difficult to implement. Many institutions worldwide are committed today to trying to transfer it into structures and models for use as tools to measure sustainability and the effectiveness of the policies adopted in its terms. This arises particularly from a growing tendency to structure strategies for sustainable development in long, medium and short-term plans, all accompanied by general and specific measurable objectives. There has been a proliferation of models, more or less structured, based on indicators providing more or less complete representations of sustainability. There are now many indices for measuring sustainability and progress towards sustainable development, at global and national scales.
The objectives of global-scale sustainability policies are perceived only when closely related to measurable results in the field. Therefore, current research on sustainability is an important reference point for sectorial research, such as the management of water resources. Actually, the main themes of current scientific interest concerning water resources are related to proper allocation of available resources, assessing vulnerability to natural disasters and other incidents, evaluation of the climate change effects on water schemes, and management optimization (Bi, Dandy, & Maier, Citation2015; Ciaponi & Creaco, Citation2018; Kazem, Mc.Phee, Torkaman, Rashid, & Kazem, Citation2016; Maiolo, Mendino, Pantusa, & Senatore, Citation2017; Maiolo & Pantusa, Citation2017, Citation2018; Maiolo et al., Citation2018; Vasan & Simonovic, Citation2010). Sustainable water resource systems are designed and operated so that they are relatively adaptive, robust, and resilient to change. Sustainable water resources systems must be capable of functioning effectively under conditions of changing supplies, management objectives, and demands (Loucks, Citation1999).
Various approaches and models have been developed for measuring sustainability in water resources. The Delft Hydraulics Laboratory in the Netherlands proposed a procedure for measuring the contribution(s) of a water resources project to sustainable development (Baan, Citation1994). It is based on the combination of indices related to five criteria, each subdivided into sub-criteria. The five main criteria are: socio-economic aspects and impacts on growth, resilience, and stability; the use of natural and environmental resources including raw materials and discharge of wastes within the carrying capacity of natural systems; enhancement and conservation of natural and environmental resources, and the improvement of the carrying capacity of natural and environmental resources; public health, safety, and well-being; flexibility and sustainability of infrastructure works, management opportunities for multifunctional use, and opportunities to adapt to changing circumstances. Each sub-criterion is given equal weighting. The sum of numerical values given to each sub-criterion is a sustainability index.
Loucks (Citation1997) proposed another approach for identifying sustainability indices relative to a weighted combination of reliability, resilience and vulnerability measures of various economic, environmental, ecological and social criteria. First, the appropriate economic, environmental, ecological and social criteria must be identified. They must then be capable of expression quantitatively (or linguistically) and be determined from water resource system time-series variables.
Cai, McKinney, and Lasdon (Citation2002) proposed a new long-term modelling framework using quantified sustainability criteria in a basin optimization model, ensuring risk minimization in water supply, environmental conservation, equity in water allocation, and economic efficiency in water infrastructure development.
Sullivan (Citation2002) proposed a water poverty index (WPI) to assess the link between poverty and water availability. It was concluded that connecting the physical and social sciences to address the problem would make it possible to find a fair solution for water allocation.
Although Canada does not face the same water poverty challenges as elsewhere, it does experience regional and community level disparities in water sustainability. Departing from WPI, the Policy Research Initiative (PRI Citation2007) developed the Canadian Water Sustainability Index (CWSI), integrating the physical, environmental and socio-economic aspects of water resources in Canada.
A different approach to sustainability assessment is based on the concept of entropy, as a measure to describe process reversibility, which is also usable in addressing the problems and choices inherent in water resource management. Such an approach proposed by Nachtnebel (Citation2002) uses the laws of thermodynamics to assess the energy required to bring a system to its initial condition. This can be a useful indicator for evaluating sustainable development.
Maiolo, Martirano, Morrone, and Pantusa (Citation2006) proposed a sustainability synthetic territorial index (ISST) to assess the sustainable management of water resources. This model was derived in the EC research project EPSILON “Environmental Policy via Sustainability Indicators On a European-wide NUTS-III level” (Bonazountas, Smirlis, & Kallidromitou, Citation2004) and is applicable at the local scale.
Chaves& Alipaz (Citation2007) developed a watershed sustainability index (WSI), to integrate hydrologic, environmental, life and policy issues, as well as existing pressures and policy responses, in one aggregated indicator, which uses a pressure-state-response function.
Ali (Citation2009) proposed the Arab water sustainability index (AWSI), a conceptual framework incorporating various physical, socio-economic and environmental elements of water status in the Arab region. A multivariate model, based on the principal component analysis, has been designed to provide information on the most meaningful parameters. The index structure was developed by mathematical aggregation of eight indicators based on 22 variables condensed into manageable information sets. These are further condensed into an index that can be translated into a policy-oriented measure.
The Sustainability Index for Integrated Urban Water Management (SIUWM) was proposed by De Carvalho, Carden, and Armitage (Citation2009); the index contains 5 components derived from 20 indicators and, ultimately, 64 variables. Sustainability is extrapolated from the perspective of the “triple bottom line”, which highlights social, environmental and economic dimensions.
In a two-part series, a water sustainability index for West Java, Indonesia was proposed by (Juwana et al., Citation2010a; Juwana, et al., Citation2010b). The first part is related to the conceptual framework and comprises a set of four components and 11 indicators. The second part introduces the application of the Delphi technique to finalize the West Java Water Sustainability Index (WJWSI) framework based on feedback from stakeholders.
A water resources sustainability index to evaluate and compare alternative management policies for water resources systems was proposed by Sandoval-Solis, McKinney, and Loucks (Citation2011). This is a variation of the sustainability index developed by Loucks (Citation1997) with improvements in structure, scale, and content, to make it more flexible and adjustable to individual basin requirements.
A sustainable cities water index used to examine the water sustainability of 50 cities in 31 countries worldwide was proposed by Arcadis (Citation2012). Cities are ranked according to how sustainability they manage and maintain water, as well as against their natural risk and vulnerability measured in terms of resilience, efficiency and quality.
A regional integration framework for assessing water resource sustainability was proposed by Gonzales and Ajami (Citation2015). To compute a quantitative measure of sustainability, a numerical index comprising supply, demand, and adaptive capacity indicators, was developed. It includes an innovative way to account for the importance of having diverse supply sources.
A sustainable water index (SWI) to estimate the extent of water use sustainability at administrative level in Korea, adopting the Pressure-State-Response (PSR) framework from Organisation for Economic Cooperation and Development (OECD), was developed by Kim, Kim, and Kong (Citation2016).
Table summarizes the main models and approaches for measuring water resources sustainability described above.
Table 1. Main models for measuring water resources sustainability
Most models reported in the literature are very complex, and sustainability assessments are mainly the field of supranational institutions. Increasing sensitivity to the issue of sustainability has led to regulatory changes worldwide. In the EU, for example, recent water resources directives are oriented towards sustainability. Therefore, the models for estimating water management sustainability should take account of EU planning and guidelines in this area.
In this context, the main objective of the work described here is to define a sustainable water management index that can be used to measure the sustainability of water management and the effectiveness of the sustainability policies adopted. As a framework for water resources management, this index refers to the EU directives establishing Community action in the field of water policy. In particular, the Index refers to Directive 2000/60/EC (Citation2000), to Council Directive 98/83/EC (Citation1998), to Directive 2006/118/EC (Citation2006), to Commission Directive 2014/80/EU (Citation2014), to Directive 2006/7/EC (Citation2006) and to Directive 2008/56/EC (Citation2008). The index is based on potential increases in the availability of data and can be applied at national, regional and/or local level.
SWaM_Index is calculated from basic environmental, economic, social and institutional indicators, and is designed to be used easily by the various administrations involved in the sector. Using a proper hierarchical relationship, the indicators are aggregated into sub-themes, themes and pillars, and the synthetic index is calculated using a proper clustering technique. Water resources management is considered in terms of both natural (the different types of water resources existing naturally) and artificial systems (set up to manage natural resource use), as well as environmental and socio-economic points of view. The index is based on a proper water resources legal framework. While EU directives are referred to in this paper, SWaM_Index proposes a methodological approach which can be adapted to other regulatory contexts.
2. Method
Three sustainability dimensions, known as pillars, are used in SWaM_Index:
− ARTIFICIAL SYSTEMS (AS)
− NATURAL SYSTEMS (NS)
− SOCIO-ECONOMIC-INSTITUTIONAL SYSTEMS (SEI)
These pillars are subdivided in themes, each theme into sub-themes and, finally, each sub-theme into elementary indicators.
Pillar AS is based on two directives from the European Parliament:
Directive 2000/60/EC (Citation2000)
Council Directive 98/83/EC (Citation1998).
It consists of 3 themes, 6 sub-themes, and 49 indicators.
The NS pillar is based on:
Directive 2000/60/EC (Citation2000) for the definition of quality policies and objectives for all waterbodies, and for surveillance procedures;
Directives 2006/118/EC (Citation2006) and Commission Directive 2014/80/EU (Citation2014) on measures to prevent pollution and deterioration of groundwater;
Directive 2006/7/EC (Citation2006) on the management of bathing water quality;
Directive 2008/56/EC (Citation2008) on the definition of marine water quality policies and objectives.
It has 5 themes, 8 sub-themes, and 33 indicators.
The SEI pillar is based on statistical, socio-economic and environmental data available from national and/or European databases. It consists of 3 themes, 7 sub-themes, and 11 indicators.
According to the model structure, the construction of synthetic index necessarily has to be done following different levels of aggregation, taking into account a horizontal dimension, in which the indicators are normalized, and a vertical dimension, in which the normalized data are composed.
The first activity is a data pre-processing consisting of two main steps: (1) a quality check on the data, disregarding those with evident errors; (2) a rectification of the indicator direction, assigning—before the normalization and aggregation steps—a multiplication factor of −1 to those indicators moving in a direction opposed to sustainability (for instance, the indicator of the losses in a water distribution network has to be rectified, since higher values—i.e. high losses—move away—from and not toward—to sustainability).
After pre-processing, the data must be normalized using any one of the many methods available. For this study, the distance-to-target method was used because the careful definition of the different targets, despite being difficult, enables direct measurement of the effectiveness of the sustainability policies adopted.
The targets were taken directly from the most binding EU-level documents available in terms of water policy, in particular, those Directives used as indicators (and noted above).
After data normalization, the first aggregation is carried out to move from elementary indicators to sub-themes. The second aggregation moves from sub-themes to themes, and the third from themes to pillars, before the fourth yields the synthetic index. The index is denoted by Ij the elementary indicator and Tj the corresponding target, with Dj the distance to target defined—EquationEquation (1)(1)
(1) :
In EquationEquation (2)(2)
(2) , DjN is the distance to the normalized target, [−1, 1] obtained through the first aggregation:
where SSN is the normalized sub-theme, [−1, 1].
Through the second aggregation:
where TTN is the normalized theme, [−1, 1].
Through the third aggregation:
where PpN is the normalized pillar, [−1, 1].
The fourth aggregation leads to:
Indicators that have non-numeric values are assigned 1 if the target is achieved and −1 otherwise. Where indicator values refer to classes or categories (e.g. “sufficient/good/high”), numerical values are assigned proportionally between −1 and 1.
The SWaM_Index have then been clustered in the following five categories following a fixed system:
− Very low (−1 ≤ values < −0,6)
− Low (−0,6 ≤ values < −0,2)
− Medium (−0,2 ≤ values < 0,2)
− High (0,2 ≤ values < 0,6)
− Very high (0,6 ≤ values ≤ 1)
The total number of indicators for the three pillars is synthetically shown in the Table . Table – show the schematic structures of pillars AS, NS and SEI, and the target values. The complete structure of the pillars with the description of all the indicators is provided in Annex.
Table 2. Number of indicators for the SWaMI_Index
3. Discussion and conclusions
The present work proposes an index for the sustainable management of water resources. This index, SWaM_Index, has some special features. Regarding the normalization method, among different normalisation methods available in the literature, the distance-to-target method has been selected, because a careful definition of the different targets, even though is a difficult task, it allows to directly measure the effectiveness of the adopted sustainability policies. The indicator measurement unit is the distance to the corresponding target, which is determined objectively on the base of the European legal framework for the sector concerned. Although the index requires an effort in the data collection phase, the index structure is derived from the legal frameworks, which makes it robust in terms of scientific rationale and applicability in the real world and has the potential to increase data availability because many of the procedures and instruments are legal requirements in the EU.
Most models analyse water management sustainability starting from a global analysis, evaluating general sustainability objectives and problems, at the national and supranational level, while SWaM_Index can be applied at national, regional and/or local level.
In view of this, the most important features of this index are related to its potential for use by managers as a tool for estimating sustainability and measuring the effectiveness of sustainability policies. It can also be a useful scientific tool to build validated parametric structures. The characterization of the sustainability indicators also enables quick assessment of management actions with reference to different uses and scenarios.
The perspectives that can spring from the described index and from its following validation are of great interest: from a simple tool of benchmarking among adjacent and/or distant territorial zones, to more complex support tool to the decisions of the Water Systems managers.
Additional information
Funding
Notes on contributors
M. Maiolo
M. Maiolo is Associate Professor at the Department of Environmental and Chemical Engineering, University of Calabria. His research interests are related to sustainable management of water resources, optimization of water resource management, hydraulic and maritime engineering, with specific experience in drinking water risk, drinking water consumption, drinking water supply systems. He is coordinator of Italian Regional Project (POR CALABRIA FESR 2014-2020) – Innovation and Competitiveness (I&C) -project: “origAMI – original Advanced Metering Infrastructure.
D. Pantusa
D. Pantusa received the degree in Civil Engineering in 2002 from University of Calabria and her Ph.D degree from University of Calabria in 2007. She is currently Research Associate at the Department of Innovation Engineering, University of Salento. Her research activity is mainly focused on sustainable management of water resources, drinking water risk, drinking water supply systems, optimization of water resource management, drinking water consumption, hydraulic and maritime engineering.
References
- Ali, H. M. M. (2009) Development of arab water sustainability index using principal component analysis. Proceedings of the Thirteenth International Water Technology Conference IWTC13, Hurghada, Egypt
- Arcadis (2012) Sustainable cities water index. Retrieved from: https://www.arcadis.com/media/4/6/2/%7B462EFA0A-4278-49DF-9943-C067182CA682%7DArcadis_Sustainable_Cities_Water_Index-Web.pdf
- Ayres, R. U. (2007). On the practical limits to substitution. Ecological Economics, 61, 115–14. doi:10.1016/j.ecolecon.2006.02.011
- Baan, J. A. (1994, June) Evaluation of water resources projects on sustainable development. Proceedings of the International Symposium on Water Resources Planning in a Changing World (p. 19), 28-30, Karlsruhe, Germany: Water International.
- Bi, W., Dandy, G. C., & Maier, H. R. (2015). Improved genetic algorithm optimization of water distribution system design by incorporating domain knowledge. Environmental Modelling & Software, 69, 370–381. doi:10.1016/j.envsoft.2014.09.010
- Bonazountas, M., Smirlis, Y., & Kallidromitou, D. (2004, October) Assessing Sustainability of EU regions: The case of the EPSILON tool, 18th International Conference on Informatics for Environmental Protection, Enviroinfo Geneva. Retrieved from: http://enviroinfo.eu/sites/default/files/pdfs/vol110/0001.pdf
- Cai, X. M., McKinney, D. C., & Lasdon, L. S. (2002). A framework for sustainability analysis in water resources management and application to the Syr Darya Basin. Water Resources Research, 38, 211–214. doi:10.1029/2001wr000214
- Chaves, H. M. L., & Alipaz, S. (2007). An integrated indicator based on basin hydrology, environment, life, and policy: The watershed sustainability index. Water Resources Management, 21, 883–895. doi:10.1007/s11269-006-9107-2
- Ciaponi, C., & Creaco, E. (2018). Comparison of pressure-driven formulations for WDN simulation. Water, 10(4), 523–537. doi:10.3390/w10040523
- Coalition Eau (2016) Is drinking water affordable for all? http://www.emwis.org/documents/meetings/events/selected-events-5th-world-water-forum-istanbul-36778/rapports-prepares-par-henri-smets-pour-istanbul/01-EAU-FR-5mars.pdf/download/1/01-EAU-EN-5mars.pdf
- Commission Directive 2014/80/EU of 20 June 2014 amending Annex II to Directive 2006/118/EC of the European Parliament and of the Council on the protection of groundwater against pollution and deterioration Text with EEA relevance, OJ L 182, p. 52–55, http://data.europa.eu/eli/dir/2014/80/oj
- Costanza, R., Cumberland, J., Daly, H., Goodlan, R., & Norgaard, R. (1997). An introduction to ecological economics. Boca Raton, USA: CRC Press LLC Corporate Blvd. N.W. 2000. ISBN-13: 978-1884015724.
- Council Directive 98/83/EC of 3 November 1998 on the quality of water intended for human consumption, OJ L 330, p. 32–54, http://data.europa.eu/eli/dir/1998/83/oj
- Daly, H. E. (1991). Steady-state economics. Washington, D.C., USA: Island Press. ISBN: 9781597268721.
- De Carvalho, S. C. P., Carden, K. J., & Armitage, N. P. (2009). Application of a sustainability index for integrated urban water management in Southern African cities: Case study comparison – Maputo and Hermanus. Water SA, 35, 144–151. doi:10.4314/wsa.v35i2.76727
- Directive 2000/60/EC of the European Parliament and of the Council of 23 October 2000 establishing a framework for Community action in the field of water policy, OJ L 327, p. 1–73, https://eur-lex.europa.eu/eli/dir/2000/60/oj
- Directive 2006/118/EC of the European Parliament and of the Council of 12 December 2006 on the protection of groundwater against pollution and deterioration, OJ L 372, p. 19–31, http://data.europa.eu/eli/dir/2006/118/oj
- Directive 2006/7/EC of the European Parliament and of the Council of 15 February 2006 concerning the management of bathing water quality and repealing Directive 76/160/EEC, OJ L 64, p. 37–51, http://data.europa.eu/eli/dir/2006/7/oj
- Directive 2008/56/EC of the European Parliament and of the Council of 17 June 2008 establishing a framework for community action in the field of marine environmental policy (Marine Strategy Framework Directive) (Text with EEA relevance), OJ L 164, p. 19–40, http://data.europa.eu/eli/dir/2008/56/oj
- Gonzales, P., & Ajami, N. K. (2015). Urban water sustainability: An integrative framework for regional water management, Hydrol. Earth System Science, 12, 11291–11329. doi:10.5194/hessd-12-11291-2015
- Hediger, W. (1999). Reconciling “weak” and “strong” sustainability. International Journal of Social Economics, 26, 1120–1144. doi:10.1108/03068299910245859
- Hediger, W. W. (2006). and strong sustainability, environmental conservation and economic growth. Natural Resource Modeling, 19, 1–30. doi:10.1111/j.1939-7445.2006.tb00185.x
- Juwana, I., Perera, B., & Muttil, N. (2010a). A water sustainability index for West Java - Part 1: Developing the conceptual framework. Water Science and Technology, 62, 1629–1640. doi:10.2166/wst.2010.452
- Juwana, I., Perera, B., & Muttil, N. (2010b). A water sustainability index for West Java-part 2: Refining the conceptual framework using Delphi technique. Water Science and Technology, 62, 1641–1652. doi:10.2166/wst.2010.453
- Kazem, M., Mc.Phee, D., Torkaman, A., Rashid, A., & Kazem, A. (2016). Climate change and economic approaches into water allocation optimization via direct benefits of water the case study of Rudbar Lorestan hydropower dam (Iran). Sustainable Water Resources Management, 2, 461. doi:10.1007/s40899-016-0067-2
- Kim, I., Kim, Y., & Kong., I. (2016, May) Assessing sustainable water use and local economy in Korea. Proceedings of the 36°Annual Conference of the International Association for Impact Assessment, 11-14, Nagoya, Japan.
- Loucks, D. P. (1997). Quantifying trends in system sustainability. Hydrological Sciences Journal, 42, 513–530. doi:10.1080/02626669709492051
- Loucks, D. P. (1999). Sustainability criteria for water resources systems. London, UK: Cambridge University Press. ISBN 0521560446, 9780521560443.
- Maiolo, M., Martirano, G., Morrone, P., & Pantusa, D. (2006). Assessment criteria for a sustainable management of the water resources. Water Practice & Technology, 1. doi:10.2166/wpt.2006.012
- Maiolo, M., Mendino, G., Pantusa, D., & Senatore, A. (2017). Optimization of drinking water distribution systems in relation to the effects of climate change. Water, 9, 803. doi:10.3390/w9100803
- Maiolo, M., & Pantusa, D. (2017). Combined reuse of wastewater and desalination for the management of water systems in conditions of scarcity. Water and Ecology, 4, 116–126. doi:10.23968/2305–3488.2017.22.4.116–126
- Maiolo, M., & Pantusa, D. (2018). Infrastructure Vulnerability Index of drinking water systems to terrorist attacks. Cogent Engineering, 5, 1456710. doi:10.1080/23311916.2018.1456710
- Maiolo, M., Pantusa, D., Carini, M., Capano, G., Chiaravalloti, F., & Procopio, A. (2018). A new vulnerability measure for water distribution network. Water, 10, 1005. doi:10.3390/w10081005
- Nachtnebel, H. P. (2002). Irreversibility and sustainability in water resources systems. Risk, Reliability, Uncertainty, and Robustness of Water Resources Systems. International Hydrology Series. Cambridge University Press: London, UK, ISBN: 9780511546006.
- Neumayer, E. (2003). Weak versus strong sustainability: Exploring the limits of two opposing paradigms.UK: Edward Elgar Cheltenham. ISBN 1843764881.
- Policy Research Initiative. (2007) Canadian water sustainability index. Retrieved from: http://policyresearch.gc.ca/doclib/SD_PR_CWSI_web_e.pdf
- Sandoval-Solis, S., McKinney, D. C., & Loucks, D. P. (2011). Sustainability index for water resources planning and management. Journal of Water Resources Pl-Asce, 137, 381–390. doi:10.1061/(ASCE)WR.1943-5452.0000134
- Sullivan, C. (2002). Calculating a Water Poverty Index. World Development, 30, 1195–1210. doi:10.1016/S0305-750X(02)00035-9
- Vasan, A., & Simonovic, S. P. (2010). Optimization of water distribution network design using differential evolution. Journal of Water Resources Pl-Asce, 136, 279–287. doi:10.1061/(ASCE)0733-9496(2010)136:2(279)
- World Commission on Environmental and Development Our Common Future (WCED). (1997). The Brundtland Report (pp. 0–338). New York, NY: Oxford University Press. Retrieved from http://www.un-documents.net/our-common-future.pdf
ANNEX
Table 3. The AS pillar structure
Table 4. The NS pillar structure
Table 5. The SEI pillar structure