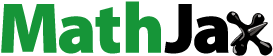
Abstract
The acceptance of liquefied petroleum gas (LPG) cookstove has increased across the country in recent years; however, their performance has not been investigated and established. To achieve this, laboratory tests were carried out to evaluate the thermal efficiency, and industrial combustion analyser (E-instrument E8500) was then used to characterize and quantify the air emissions from combustion of conventional LPG cookstoves in the country. The average thermal efficiency for all tested burners was 66.267 ± 7.218% meeting Tier 4 of the International Organization for Standardization, International Workshop Agreement 11. The mean concentration of CO, NOx, HC, and SO2 was in the range of 3–530, 48–810.5, 99–4176, and 0–4,respectively,and the range of CO2 was 1,792–3,592 mg/m3,which were compared with stationary source limit by Federal Ministry of Environment Standard, Nigeria. The toxicity potential of NOx and SO2 was below unity, 25% of the burners tested were below unity for CO, and HC was higher than unity for all tested burners. Most cookstoves met the guidelines for CO, NOx, and SO2. Hence, LPG in Nigeria could serve as a source of energy for cooking.
PUBLIC INTEREST STATEMENT
This study investigated the emission characterization and performance of conventional liquefied petroleum gas (LPG) cookstove burners. To achieve this, laboratory tests were carried out to evaluate the thermal efficiency, the firepower, and water boiling test (WBT), and industrial combustion analyser (E-instrument E8500) was then used to characterize and quantify the air emissions from combustion of conventional LPG cookstoves in the country. The quantified air emission was then used to determine the toxicity potential of the gas burners.
1. Introduction
The transition from fuelwood to kerosene for domestic cooking in Nigeria was smooth due to government subsidies on kerosene, which increased the availability and affordability of the product. However, in 2016, kerosene subsidy was removed, and this led to an increase in pump price and the product became scarce due to its price doubling (Isihak, Akpan, & Adeleye, Citation2012). Cooking with kerosene produces health-damaging air pollutants that are above the World Health Organization (WHO) Air Quality Guidance levels; therefore, kerosene is now considered as a polluting fuel and WHO recommends against using it as a domestic cooking fuel (WHO, Citation2014).
With scarcity of kerosene and unstable electricity from the national grid, adoption of liquefied petroleum gas (LPG) for cooking in the country has improved. LPG is readily available and affordable in urban areas of Nigeria (Van Leeuwen, Evans, & Hyseni, Citation2017). It has also been established as a source of energy used for cooking with expected health, economic, environmental, and social benefits (Bruce, Aunan, & Rehfuess, Citation2017). LPG is the most convenient and clean fuel for domestic use and is very popular these days (Rosenthal, Quinn, Grieshop, Pillarisetti, & Glass, Citation2018). LPG is an exceptional energy source due to various advantages, which include clean cooking environment as well as high heat content of the fuel (Wang, Liu, Huang, & Liu, Citation2016). The major potential hazard with LPG is fire and explosion. Hazard may also rise at the point of use if ventilation is inadequate and the products of combustion are not dispersed into the atmosphere (Ifegbesan, Rampedi, & Annegarn, Citation2016). The risk associated with such hazards can be controlled using available proven technologies, which include safety equipment and general procedures given by the LPG industries or outlets. The user of this fuel should be able to recognize the smell of odourized LPG, the need for care, and adequate ventilation (Hutton, Rehfuess, & Tediosi, Citation2007).
In domestic combustion system, the burner head plays a pivotal role in the combustion of LPG fuel. An inefficient design of a burner often leads to an incomplete combustion. To solve this problem, it is important to choose the correct design for the burner head and its material of construction (Panigrahy, Mishra, Mishra, & Muthukumar, Citation2016). The classification of gas burner is done by the size of the burner that mixes LPG and air before burning in the combustion chamber. The primary purpose of using LPG burners is to produce the maximum heat possible when combusting the fuel (Hou & Chou, Citation2013). The combustion in the burner of a domestic LPG cooking stove takes place in a gaseous environment, and the flame stabilizes over the surface of the burner (Singh, Chhabra, Sehgal, & Singh, Citation2017). There are many different types of burners with distinct designs often made from different materials (Shen et al., Citation2018).
Several factors have been reported to be responsible for the efficiency of the cookstoves. In the case of LPG burners, the design of the burner head, the design of the cooking vessel, and the rate of flow of the gas were the major factors (Shrestha, Citation2001). Studies further concluded that increasing the efficiency of the cooking systems is possible by using burners with optimum design, using vessels with flat bottoms, operating at flow rates that provide the highest efficiency, and using a porous burner (Khan, Saxena, & Katiyar, Citation2015). The calorific value of the fuel is a major factor for calculating the efficiency of a stove. The calorific value of the fuel varies due to composition, ways of fuel extraction, and contamination with different substances (Saleh, Citation2008). Various researches had been done to determine the calorific value of LPG. According to Agarwal, Anand, and Gupta (Citation2015), the calorific value was 46.1MJ/kg. The calorific value of 49.0 MJ/kg and 45.8 MJ/kg was reported by Zhang et al. (Citation2000) and Smith et al. (Citation2000), respectively.
WBT is developed to provide details about the time required to boil water and the thermal efficiencies of cookstoves (WHO, Citation2014). There are various protocols available for conducting the WBT (IS 4246:2002) (Agarwal et al., Citation2015). Shen et al. (Citation2018) also reported a different procedure for the WBT. One of the main challenges with this test protocol is that different procedures can be used by different users (Chen et al., Citation2016). The important feature of LPG is that its stove efficiency of about 55–60% is much higher than that of many other stoves. The thermal efficiency of the LPG cooking stove with regular cast iron burner head available in the market showed an efficiency of 47–49% (Khan et al., Citation2015).
The combustion of LPG in air usually produces emissions that are known to be hazardous to human health and climate (Waheed, Baek, Javed, & Kristiyanto, Citation2015). The main purpose for combustion of LPG in cookstoves is to release all the heat in the fuel (Panigrahy et al., Citation2016). Good combustion is achieved by maintaining high temperature to ignite the fuel, adequate mixing of oxygen and LPG, and sufficient residence time for combustion (Roden et al., Citation2009).
National efforts are directed to the usage of clean fuels, such as LPG, for cooking with a view to reduce indoor air pollution and improve the quality of air and human health protection. Consumption of LPG in Nigeria has increased due to its availability in some major parts across the country, and this will have an environmental impact on indoor air quality, and its implications as regards various air pollutants from the combustion of LPG during household cooking needed to be evaluated. Improved adoption of LPG for cooking in Nigeria has resulted in the presence of different burners in the country. However, the indoor air quality implications of these burners are yet to be established. Hence, this study identified common domestic burners available in Nigeria markets and characterized gaseous air pollutants from the combustion of LPG. These are considered necessary in order to evaluate the air quality implication of the use of LPG as a source of household cooking energy.
2. Methodology
2.1. Burners identification and collection
Market survey was carried out to identify common burners available in the Nigeria market. The burners were assembled at the Environmental Engineering Research Laboratory, Department of Chemical Engineering, Obafemi Awolowo University, Ile-Ife, Nigeria. Common burners in the Nigerian market were identified based on their material of construction, the number of ports (hole), and port diameter. Both old and new burners were tested to determine the effect of deterioration in the performance of a burner. The field test for the burners, which includes kitchen performance test (KPT), affects the cooking procedures of the households using the burners, which creates a great challenge for the research work; hence, the burners are assembled into the laboratory for the study with exception to burner P.
2.2. Experimental procedure
The E8500 plus combustion analyzer was used for emission characterization in this study. It is a complete, portable tool for EPA compliance-level emission monitoring of boilers, engines, and other combustion equipments. This combustion analyzer used has the capability to measure gaseous emission including hydrocarbons (HCs), carbon monoxide (CO), carbon dioxide (CO2), oxides of nitrogen (NO, NO2, NOX), sulphur dioxide (SO2), and hydrogen sulphide (H2S).
The experimental design involves the combustion of constant mass of LPG with the identified burner head in this study. The gas was passed for a certain period to allow uniform inlet pressure of the gas to the burner head, the stove is switched on, and the combustion analyzer gas sampling probe is inserted into the combustion zone, with the probe inserted at about 1.5 cm above the burner head. The concentration of each of the gases is stored in the combustion analyzer from the beginning of the combustion process. The experimental procedure was repeated thrice and average values were reported.
2.2.1. Performance parameters testing
The WBT was used to assess the performance parameters such as thermal efficiency (E), the firepower (P), and the specific fuel consumption (SC) of the stove.
Thermal efficiency (E) is a ratio of the work done by heating and evaporating water to the energy consumed by LPG combustion. The thermal efficiency of each burner is calculated using EquationEquation (1)(1)
(1) (Anozie, Bakare, Sonibare, & Oyebisi, Citation2007);
where E is thermal efficiency (%), Mw is the mass of water (kg), Cp is the specific heat capacity of water at constant pressure and mean temperature of water (J/kgK), T2 is the boiling temperature (°C),T1 is the initial water temperature (°C), Me is the mass of water evaporated (kg), L is the latent heat of vaporization of water (kJ/kg), Mf is the weight of fuel burnt (kg), and Ef is the calorific value of the fuel (MJ/kg).
Firepower (P) is a ratio of the LPG energy consumed by the stove per unit time (in W) during the test. Also referred to as cooking power, mathematically, it has a unit in KJ/s and expressed as in EquationEquation (2)(2)
(2) (Kshirsagar & Kalamkar, Citation2014)
Specific fuel consumption (SC) is the ratio of the amount of LPG consumed to the amount of water boiled for each trial. In this case, specific fuel consumption refers to a measure of the amount of LPG required to produce 3 kg of boiling water, mathematically expressed as in EquationEquation (3)(3)
(3) (Kshirsagar & Kalamkar, Citation2014).
Combustion efficiency gives the percentage of how effective a heating equipment is able to convert fuel into useful heat energy. Modified combustion efficiency (MCE, defined as CO2/(CO2 + CO) on a molar basis) is considered as a proxy for true combustion efficiency (Shen et al., Citation2018).
Burning rate is defined as the ratio of LPG consumed to the time taken for combustion (Shen et al., Citation2018).
2.2.2. Experimental procedures for WBT
The water boiling procedure used in this study is the standard WBT (Anozie et al., Citation2007). 3 kg of water was measured into the aluminium cooking pot; the temperature of the water inside the pot was taken as the initial temperature with a thermometer. A thermometer (0–100°C) was used to evaluate the water temperature during experimentation. A stirrer made of aluminum was used for stirring the water; this is to achieve uniform distribution of heat and temperature inside the pot. The weight of cooking pot (0.333 kg) and the weight of water (3 kg) used in the vessel were noted. The initial temperature of water (T1) was also measured. The weight of cylinder along with fuel (W1) was noted. The burner was lighted and water was heated with a temperature difference of 70°C from the initial value of the temperature and stirred continuously for uniformity of temperature until the final temperature was reached. Then the burner was put off. Again, the weight of the cylinder (W2) was recorded. Quantity of water evaporated after boiling was determined. The difference in the weight of cylinder (W2−W1) gives the mass of LPG consumed for heating water by temperature (T2−T1). The time at which the water in the pot reached the final temperature was recorded using a stopwatch. The experiment was carried out under a tripod stand. The burner head was removed and another one was inserted to the cylinder. The experiment was repeated three times and the average values were taken as final reading to ascertain the burner consistency when used in the household setup. The line diagram and experimental setup for WBT are presented in Figure and Plate 1. The uncertainty in the mass of cylinder used is 3 ± 0.001 kg, mass of water boiled is 3 ± 0.001 kg, and thermometer used is 100 ± 0.1°C.
Figure 1. The water boiling test experiment (adapted from Khan et al., Citation2015)
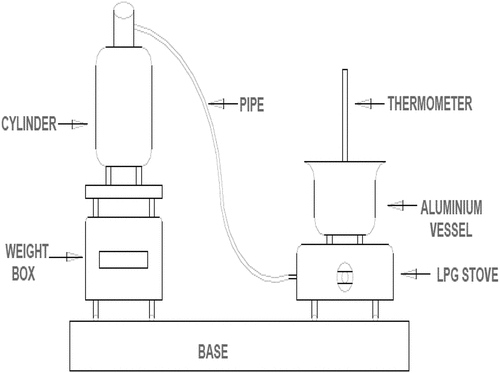
2.3. Toxicity potential of emission by tested burners
Toxicity potential (TP) is a quantitative toxic equivalency introduced to express the potential harm of a unit of air pollutants released into the immediate environment. It is expressed as the ratio of measured pollutants concentration to the statutory limit of concentration (Fakinle et al., Citation2018; Fakinle, Sonibare, Akeredolu, Okedere, & Jimoda, Citation2013). The TP helps in assessing the effects of the emission of air pollutants from the combustion of LPG in the indoor environment on human health. It is calculated using EquationEquation (4)(4)
(4) , given the consideration to the standard set for stationary source of emission by the Federal Ministry of Environment (FMEnv) standards as presented in Table .
The upper limits of the standard were used for computing the TP of NOx and SO2, which gives a better representation of the TP values of the two pollutants.
Table 1. Emission limits for specific pollutants from a stationary source
3. Results
3.1. Identified common burners in the Nigerian market
The common burners identified in this study (Table ) are found to be of different materials of construction, different numbers of ports, and different port diameters. The burner heads are made of cast iron, brass, and stainless steel (Plate 2 and Plate 3).
Table 2. Specification of LPG burners identified
3.2. Emission characteristic of the burners
The measured average and standard deviation values for air pollutants recorded from the experiment of LPG combustion are presented in Table . The graphical representation is also presented in Figure .
Figure 2. Comparison of the measured average concentrations of gaseous emissions of air pollutant from different burners tested
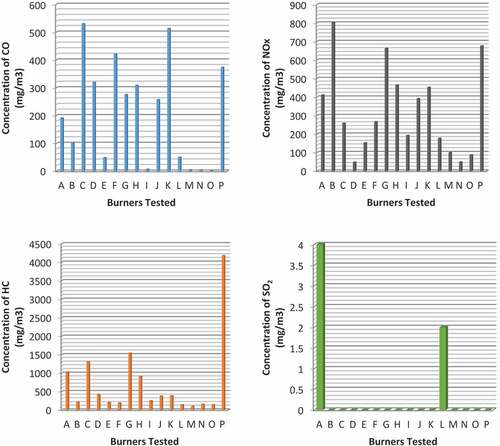
Table 3. Measured mean and standard deviation for the concentration of air pollutants from LPG cookstoves
As presented in Table , the TP of burners was tested using the FMEnv standard for stationary source. The TP was ranged from 0.3 to 51.33 for CO, 0.05 to 0.80 for NOx, 1.98 to 83.52 for HC, and 0 to 0.0006 for SO2.
Table 4. Computed toxicity potential from measured concentration for different burners
The computed performance metrics of the identified burners from the WBT as presented in Table suggest that the differences in burner design, material, and diameter of port across the 15 burners during the experiment may be important factors affecting the observed differences in the thermal efficiency, firepower, specific fuel consumption, burning rate, and combustion efficiency.
Table 5. Average values of parameters obtained during the water boiling test experiment
For all tests in the WBT experiment, the range of thermal efficiencies was 52–79%, 0.86–1.89 KJ/s for stoves firepower, 0.484–0.719 MJ/kg for the specific fuel consumption, 1.480–4.091 g/min for rate of burning, and 77.2–99.8% for the MCE for all stoves tested. However, the overall mean and standard deviation for thermal efficiency was 66.267 ± 7.218%, for firepower was 1.503 ± 0.300 KJ/s, for specific fuel consumption was 0.578 ± 0.059, for burning rate was 3.149 ± 0.835, and for combust7ion efficiency was 92.113 ± 7.072. The mean and standard deviation of time taken to boil the water was 13.400 ± 4.339 min, and the water evaporated during the study was 0.096 ± 0.021 kg.
3.3. Discussion on results
The main composition of exhaust gas emission from the LPG combustion air flames in this study is CO, CO2, HC, NOX, NO, NO2, and SO2. Carbon monoxide monitoring will remain a useful tool in the qualitative ranking of households in terms of emission levels (Ezzati, Saleh, & Kammen, Citation2000). Emission of CO might result from combustion due to low combustion temperature, moisture content, insufficient oxygen, poor mixing of fuel with combustion air, and short residence time of combustion gas in the combustion zone (Tissari, Hytönen, Sippula, & Jokiniemi, Citation2009). In this study, it was observed that burnersM, N,and O made in China have the least emission concentration of air pollutants. However, burners B and D made in China were of high concentrations due to incomplete combustion as they are old burners. Burner E was made in India; it was observed to have a lower concentration of emissions for air pollutants. Burners (A, B, F, G, H, J, K, and L) made in Italy were observed to have the highest concentrations of pollutants emitted (Table ). Burners made in China and India have lower concentrations of air pollutants compared to those made in Italy. The effect of burner’s material of construction on emissions was considered in this study. It was observed in the study that burners made of brass and cast iron were observed to have a lower emission of air pollutants, while burners made of stainless steel were observed to have a higher concentration of air pollutants. The effect of the number of ports and port diameter was also considered on the emission of air pollutants by each burner. It was observed that the single burners with the highest number of ports have fewer emission concentration levels and higher combustion efficiency. Burners M and N with 29 ports and 0.2 cm for each were observed to have the lowest emission concentrations for air pollutants with a combustion efficiency of 99.7% and 99.8%, respectively. Burner O also has lower emission concentration for air pollutants with a combustion efficiency of 99.8%. It is observed that the higher the combustion efficiency, the higher the number of ports and lower emission concentrations of air pollutants associated with LPG combustion.
The results obtained in this study are compared with emission limits for specific pollutants from stationary sources by FMEnv as presented in Table . It was observed that only burners I, M, N, and O met the limit set for CO, while burners A, B, C, D, E, F, G, H, J, K, L, and P were observed to breach the standard limit set for CO. Emission of NO for all the burners met the stationary source standard limit. However, HC for all burners breached the limit set for the standard emission. SO2 was below the lower limit of the standard.
Burner C has the highest concentration of CO value of 530 mg/m3, with burner O having the lowest value of 3 mg/m3. During the experiment of this study, both yellow and blue flames were observed. The blue flame was observed during the testing of all burners and the yellow flame was observed for burners D, E, F, G, J, and L due to incomplete combustion and aging of the burners. Most of the burners with yellow flame were old burners and observed to have a higher concentration of CO emission, which shows that the age of a burner also determines its emission level. The intermediate product CO is more likely to be converted to the CO2 provided enough oxygen is available at relatively high temperature. It was found that by using a higher power level (firepower), CO2 emission increases. This is due to the increase of fuel rate leading to higher carbon conversion at a higher power level.
Emission of NO, NO2, and NOx also varied differently among the burner tested; they might be from the elemental nitrogen content of the fuel (Werther, Saenger, Hartge, Ogada, & Siagi, Citation2000) and also from nitrogen present in the combustion air. It was observed in this study that as the ambient air and temperature in the combustion zone increases, the formation of NO and NOxincreases. NO emission increases due to the higher temperature of the flue gases caused by higher heat release rate at a higher power level.
Emission of HC was observed to be highest in the burner with a yellow flame. It was also found that the unburnt HC emission level also increases with the power level. Emissions of SO2 were observed in burners A and L; this is because the sulfur level in the LPG combusted is comparatively very low. Emission concentration of air pollutants from the burners tested was mostly below the set standard permitted, except for HC that breaches all standard limit set for a stationary source by the FMEnv. Hence, LPG cookstove in Nigeria could serve as a source of cooking energy with sustainable health and environmental effect.
The coefficient of variations (COVs) for the emission concentration for all tested burners in this study were in the range of 8–75% for CO, 7–80% for NOx, and 20–47% for HC as presented in Table . This shows that the emission concentration varied between the burners tested. The higher the COV, the greater the level of dispersion around the mean value, and the lower the COV, the more precise the estimate is.
Table 6. Coefficient of variance in percentage for tested burners
TP value that is above unity poses a great concern to human health and immediate environment in the indoor where such is detected. Using the FMEnv standard as presented in Table , the upper limits of the standard were used for computing the TP of NOx and SO2, which gives a better representation of the TP values of the two pollutants. Table shows that burners I, M, N,and O were below unity and burners A, B, C, D, E, F, G, H, J, K, L, and P were all above unity for CO. For NOx all burners indicate TP values that are below unity; however, the TP value for HC was above unity for all tested burners and its value for SO2 was below unity.
The mean and standard deviation of thermal efficiency in this study was 66.267 ± 7.218%, meeting guidelines for the highest tier level (Tier 4) under the International Organization for Standardization (ISO) (IWA, Citation2012). Thermal efficiencies in this study were in the range 52–79% which was similar to or somewhat higher than the range of reported values of 46–62% tested across five stoves (Shen et al., Citation2018). MacCarty, Still, and Ogle (Citation2010), Smith et al. (Citation2000), and Zhang et al. (Citation2000) also reported that thermal efficiencies were in the range of 42−54%, which were somewhat lower than what is obtained in present study. Cooking vessels used in both previous and present study were flat-bottomed pot made of stainless steel. Figure shows the comparison of thermal efficiencies with other studies. The differences observed could be as a result of different compositions of LPG, stove power level, and stove conditions. The effect of material selection on thermal efficiency was also studied in this study, and it was observed that burners of the same characteristics and specifications made of brass have higher efficiency than those of stainless steel and cast iron (brass > stainless steel > cast iron). Yunus & Saxena (Citation2013) reported a 4% increase when brass head was replaced with cast iron. Burners made of brass material were better conductors of heat than those of stainless steel and cast iron. Burners made with stainless steel were found to have higher thermal efficiencies than those made of iron. The ambient temperature during the experiment in this study was between 29°C and 31°C. It was observed that, in this study, the thermal efficiency increases as the ambient temperature increases. Muthukumar, Anand, and Sachdeva (Citation2011) also reported the same in their study.
Specific fuel (energy) consumption is a more reliable indicator of cookstove performance than thermal efficiency (Kshirsagar & Kalamkar, Citation2014). It was observed in this study that as thermal efficiencies increases, the specific fuel consumption decreases, which shows that there is an inverse relationship between the two stove performance parameters. Comparing with (IWA Citation2012) standard, the specific fuel consumption meets the lowest tier level (Tier 0).
MCE is seen as an approximation to true combustion efficiency. It was, however, observed in the present study that the lower the concentration of CO, the higher the MCE, and vice versa. Burners with blue flame were observed with higher MCE due to better combustion.
Thermal efficiency, firepower, burning rate, and combustion efficiency varied significantly among the 15 burners used during the test as presented in Table . Firepower correlated positively with the fuel burning rate (r = 0.928, P < 0.01); however, the correlation between the two parameters correlates negatively with thermal efficiency (r = −0.579 and r = −0.831, respectively, P < 0.01). Specific fuel consumption correlates negatively with thermal efficiency (r = −0.892, P < 0.01). Combustion efficiency was seen to have a positive correlation with thermal efficiency (r = 0.304, P < 0.01)
4. Conclusions and recommendation
The study provides adequate information on gaseous air pollutants associated with the combustion of LPG and performance metrics of common burners identified in the Nigeria market based on the material of construction, number of port, and port diameter. Several laboratory tests were carried out on these burners, and their emission was characterized using E-8500 combustion analyzer. Results obtained in this study could further encourage and increase the confidence of both government and private agencies on the ongoing LPG intervention programs across the country.
This study data will provide useful information on the impacts of LPG interventions on indoor and outdoor air quality in Nigeria. This is expected to influence the country policy on indoor air quality regulation. The use of LPG cookstoves, compared to solid fuel stoves, provided a significant decrease in the concentration of air pollutants, and this led to the improvement in indoor air quality. The study confirmed that high thermal efficiency and low emission of air pollutants from LPG cookstoves, material of construction of burners, and burner’s age also affect the level of emission. There is a need for future study on LPG cookstoves which should include both field and further laboratory experiment. Different compositions of LPG and minor constituents should be investigated to ascertain its effect on the level of emission. The effect of operating the cookstove at both higher and lower power levels on air pollutants should also be investigated. The government and private companies should subsidize the price of LPG across the country as this will encourage more acceptances from the user.
Additional information
Funding
Notes on contributors
Bamidele Sunday Fakinle
Bamidele Sunday Fakinle obtained his PhD in Chemical Engineering from Obafemi Awolowo University, Ile-Ife, Nigeria. He is a certified engineer and a consultant in air quality and life cycle analysis. He can be contacted at: [email protected]
Olawale Daniel Oke
Olawale Daniel Oke obtained MSc in Chemical Engineering from Obafemi Awolwo University, Ile-Ife, Nigeria. His area of specialization is air quality.
Olayemi Abosede Odunlami
Olayemi Abosede Odunlami obtained PhD in Chemical Engineering from Obafemi Awolowo University, Ile-Ife, Nigeria. She is a lecturer at Covenant University, Ota, Nigeria.
Jacob Ademola Sonibare
Jacob Ademola Sonibare is a professor of Chemical Engineering, Obafemi Awolowo University, Ile-Ife, Nigeria. He is a consultant in air quality and life cycle analysis. He can be contacted at: [email protected]
Funso Alaba Akeredolu
Funso Alaba Akeredolu is a professor of Chemical Engineering, Obafemi Awolowo University, Ile-Ife, Nigeria. He is a certified engineer and a consultant in air quality and life cycle analysis.
Olufemi Sunday Oni
Olufemi Sunday Oni obtained MSc in Chemical Engineering from Obafemi Awolwo University, Ile-Ife, Nigeria. His area of specialization is air quality. [email protected].
References
- Agarwal, P., Anand, A., & Gupta, R. (2015). Performance analysis of conventional LPG cooking stove. International Journal on Applied Bioengineering, 9, 1.
- FEPA. (1991). Guidelines to standards for environmental pollution control in Nigeria. Federal Environmental Protection Agency (FEPA). Lagos.
- Yunus, K. M., & Saxena, A. (2013). Performance of LPG cooking stove using different design of burner heads. International Journal of Engineering Research and Technology, 2(7), 656 –659.
- Anozie, A., Bakare, A., Sonibare, J., & Oyebisi, T. (2007). Evaluation of cooking energy cost, efficiency, impact on air pollution and policy in Nigeria. Energy, 32(7), 1283–18. doi:10.1016/j.energy.2006.07.004
- Bruce, N. G., Aunan, K., & Rehfuess, E. A. (2017). Liquefied petroleum gas as a clean cooking fuel for developing countries: implications for climate, forests, and affordability. Materials on Development Financing, 7, 1–44.
- Chen, Y., Shen, G., Su, S., Du, W., Huangfu, Y., Liu, G., … Tao, S. (2016). Efficiencies and pollutant emissions from forced-draft biomass-pellet semi-gasifier stoves: comparison of International and Chinese water boiling test protocols. Energy for Sustainable Development, 32, 22–30. doi:10.1016/j.esd.2016.02.008
- Ezzati, M., Saleh, H., & Kammen, D. M. (2000). The contributions of emissions and spatial microenvironments to exposure to indoor air pollution from biomass combustion in Kenya. Environmental Health Perspectives, 108(9), 833. doi:10.1289/ehp.00108833
- Fakinle, B. S., Oni, O. S., Olalekan, A. P., Sonibare, J. A., Okedere, O. B., & Odunlami, O. A. (2018). Total suspended solids and volatile organic compounds in the airshed of a reconstructed road along Lagos-Ibadan express way. International Journal of Civil Engineering and Technology, 9(11), 2420–2427.
- Fakinle, B. S., Sonibare, J. A., Akeredolu, F. A., Okedere, O. B., & Jimoda, L. A. (2013). Toxicity potential of particulates in the airshed of haulage vehicle park. Global Nest Journal, 15(4), 466–473. doi:10.30955/gnj.000948
- Hou, -S.-S., & Chou, C.-H. (2013). Parametric study of high-efficiency and low-emission gas burners. Advances in Materials Science and Engineering, 2013, 1–7. doi:10.1155/2013/154957
- Hutton, G., Rehfuess, E., & Tediosi, F. (2007). Evaluation of the costs and benefits of interventions to reduce indoor air pollution. Energy for Sustainable Development, 11(4), 34–43. doi:10.1016/S0973-0826(08)60408-1
- Ifegbesan, A. P., Rampedi, I. T., & Annegarn, H. J. (2016). Nigerian households’ cooking energy use, determinants of choice, and some implications for human health and environmental sustainability. Habitat International, 55, 17–24. doi:10.1016/j.habitatint.2016.02.001
- Isihak, S., Akpan, U., & Adeleye, M. (2012). Interventions for mitigating indoor-air pollution in Nigeria: a cost-benefit analysis. International Journal of Energy Sector Management, 6(3), 417–429. doi:10.1108/17506221211259655
- IWA, I. (2012). 11: 2012: Guidelines for evaluating cookstove performance. Geneva: International Organisation on Standardisation.
- Khan, M. Y., Saxena, A., & Katiyar, J. (2015). Performance of LPG burner with different size of ball bearing as a porous medium. Journal of Basic and Applied Engineering Research, 2, 955–957.
- Kshirsagar, M. P., & Kalamkar, V. R. (2014). A comprehensive review on biomass cookstoves and a systematic approach for modern cookstove design. Renewable and Sustainable Energy Reviews, 30, 580–603. doi:10.1016/j.rser.2013.10.039
- MacCarty, N., Still, D., & Ogle, D. (2010). Fuel use and emissions performance of fifty cooking stoves in the laboratory and related benchmarks of performance. Energy for Sustainable Development, 14(3), 161–171. doi:10.1016/j.esd.2010.06.002
- Muthukumar, P., Anand, P., & Sachdeva, P. (2011). Performance analysis of porous radiant burners used in LPG cooking stove. International Journal of Energy and Environment, 2(2), 367–374.
- Panigrahy, S., Mishra, N. K., Mishra, S. C., & Muthukumar, P. (2016). Numerical and experimental analyses of LPG (liquefied petroleum gas) combustion in a domestic cooking stove with a porous radiant burner. Energy, 95, 404–414. doi:10.1016/j.energy.2015.12.015
- Roden, C. A., Bond, T. C., Conway, S., Pinel, A. B. O., MacCarty, N., & Still, D. (2009). Laboratory and field investigations of particulate and carbon monoxide emissions from traditional and improved cookstoves. Atmospheric Environment, 43(6), 1170–1181. doi:10.1016/j.atmosenv.2008.05.041
- Rosenthal, J., Quinn, A., Grieshop, A. P., Pillarisetti, A., & Glass, R. I. (2018). Clean cooking and the SDGs: Integrated analytical approaches to guide energy interventions for health and environment goals. Energy for Sustainable Development, 42, 152–159. doi:10.1016/j.esd.2017.11.003
- Saleh, H. (2008). Effect of variation in LPG composition on emissions and performance in a dual fuel diesel engine. Fuel, 87(13–14), 3031–3039. doi:10.1016/j.fuel.2008.04.007
- Shen, G., Hays, M. D., Smith, K. R., Williams, C., Faircloth, J. W., & Jetter, J. J. (2018). Evaluating the performance of household liquefied petroleum gas cookstoves. Environmental Science & Technology, 52(2), 904–915. doi:10.1021/acs.est.7b05155
- Shrestha, J. (2001). Efficiency measurement of biogas, kerosene and LPG stoves. In Center for energy studies. Tribhuvan University Institute of Engineering (pp. 1–17).
- Singh, N., Chhabra, S., Sehgal, S., & Singh, I. (2017). Performance analysis of porous radiant burners for cooking applications. International Journal of Engineering & Technology, 6(3), 65–70. doi:10.14419/ijet.v6i3.7868
- Smith, K. R., Uma, R., Kishore, V., Lata, K., Joshi, V., Zhang, J., … Thorneloe, S. (2000). Greenhouse gases from small-scale combustion devices in developing countries, phase IIa: household stoves in India. In us environmental protection agency (pp. 1–98). NC: Research Triangle Park.
- Tissari, J., Hytönen, K., Sippula, O., & Jokiniemi, J. (2009). The effects of operating conditions on emissions from masonry heaters and sauna stoves. Biomass and Bioenergy, 33(3), 513–520. doi:10.1016/j.biombioe.2008.08.009
- Van Leeuwen, R., Evans, A., & Hyseni, B. (2017). Increasing the use of liquefied petroleum gas in cooking in developing countries. Livewire; 2017/74. Retrieved from https://openknowledge.worldbank.org/handle/10986/26569
- Waheed, K., Baek, S. W., Javed, I., & Kristiyanto, Y. (2015). Investigations on thermal radiative characteristics of LPG combustion: effect of alumina nanoparticles addition. Combustion Science and Technology, 187(6), 827–842. doi:10.1080/00102202.2014.973952
- Wang, Y., Liu, H., Huang, Z., & Liu, Z. (2016). Study on combustion and emission of a dimethyl ether-diesel dual-fuel premixed charge compression ignition combustion engine with LPG (liquefied petroleum gas) as ignition inhibitor. Energy, 96, 278–285. doi:10.1016/j.energy.2015.12.056
- Werther, J., Saenger, M., Hartge, E.-U., Ogada, T., & Siagi, Z. (2000). Combustion of agricultural residues. Progress in Energy and Combustion Science, 26(1), 1–27. doi:10.1016/S0360-1285(99)00005-2
- World Health Organization, WHO. (2014). Indoor Air Quality Guidelines: Household Fuel Combustion. Geneva, Switzerland.
- Zhang, J., Smith, K., Ma, Y., Ye, S., Jiang, F., Qi, W., … Thorneloe, S. (2000). Greenhouse gases and other airborne pollutants from household stoves in China: a database for emission factors. Atmospheric Environment, 34(26), 4537–4549. doi:10.1016/S1352-2310(99)00450-1