Abstract
In the current context of climate change and global energy demand, the use of energy from waste has become one strategy for the reduction of greenhouse gas (GHG) emissions and the replacement of fossil fuels by other non-conventional energy sources through the use of biogas produced in landfills. Although there have been some improvements in solid waste management practices in Colombia, current levels of recycling and materials recovery are still poor as only about 10% of the waste produced is recovered, so it is expected that, as for most developing countries, final disposal in landfills will continue to be the main form of municipal solid waste (MSW) management in the coming decades. The optimization of waste degradation and stabilization processes is an essential key aspect for the environmental performance and economic sustainability of waste management systems in developing countries like Colombia. However, assessing the feasibility of biogas production in landfills requires a reasonable level of accuracy for the generation of methane, a sufficient understanding of the underlying generation processes and their relation with the physicochemical characteristics of the waste and landfill disposal conditions. Source segregation of MSW is either poor or non-existing in Colombia, as in most developing countries, which makes difficult to predict landfill gas generation even with the aid of current landfill emissions models. Only a few studies have been conducted to characterize biogas and methane production potential of mixed MSW landfilled in Latin-American countries, with few studies reported in Brazil and in Colombia. In this study, we show the results of biochemical methane potential (BMP) tests with 4–5 years old samples of municipal solid waste (MSW) excavated from a landfill site located in Colombia. Collected samples were characterized and the easy and medium biodegradable fractions used in the experiments. The results show an average total production of 34.8 − 37.9 L CH4 kg-1 DM added which is comparable with similar studies using excavated landfilled waste of similar characteristics. These results suggest that considering the potential of methane production from landfilled waste in developing countries, it is an alternative that could be considered to enhance the environmental performance of landfill sites by reduction of the emissions of uncontrolled CH4 and promote the use of non-conventional energy sources.
PUBLIC INTEREST STATEMENT
The development of sustainable methods for the management of municipal solid wastes (MSW) is a priority within the current global initiatives to achieve the sustainable development goals. In this work, the energy utilization potential of wastes sent to landfills, and its relation to the compositional characteristics of MSW generated in low- and middle-income economies is investigated. This is of great interest worldwide but is particularly relevant for developing countries where enhancing the environmental performance of final disposal sites and the sustainability of their operations is a priority in terms of establishing integrated solid waste management systems for emerging and developing economies.
1. Introduction
Worldwide approximately 80% of the municipal solid waste (MSW) generated in urban areas end up in landfills and only 20% is disposed of in properly constructed and managed systems such as landfills (Themelis, Elena, Barriga, Estevez, & Velasco, Citation2013). In developing countries more than half of the waste is disposed of in open dumps (Hoornweg & Bhada-Tata, Citation2012) and although there have been advances, in several regions, such as in Latin America, the levels of coverage and quality are still very poor with serious implications for health and the environment (Wilson et al., Citation2015). Although it is possible that the generation of MSW in developing countries will change over time, it is very likely that the amount of waste sent to landfills will not decrease in the short or medium term and landfilling continue to be the main option for the final disposal of solid waste. Although some cities in low and middle-income countries have achieved recycling rates comparable to those from developed countries, waste recovery programs are still to be developed (Hoornweg & Bhada-Tata, Citation2012; HABITAT, Citation2010).
Globally, uncontrolled gas emissions coming from landfills are estimated to be between 3% and 5% and they constitute the third source of anthropogenic methane emissions (USEPA/ISWA, Citation2012). In Colombia, it is estimated that 5.03% of emissions are coming from landfills which represent 9048.25 Gg of CO2 equivalent (Pedraza et al., Citation2005), which reflects a considerable reduction potential for the sector (Larochelle, Turner, & LaGiglia, Citation2012). Nevertheless, the country agreed to reduce GHG emissions by 20% by the year 2030 (DNP, Citation2016).
Uncontrolled methane emissions from landfills are considered as a lost opportunity to capture and use a significant energy resource. The Sustainable Development Goals (SDG), which mean to achieve a more sustainable and inclusive future (United Nations Department of Economic and Social Affairs (UN DESA), Citation2018), establish the Goal 7 as the affordable and clean energy access. This initiative has been adopted by Colombia and constitutes a strong income for the setting of an agenda of science and technology (Agudelo Vélez, Chavarro Bohorquez, Hernández Tasco, & Niño Mendieta, et.al Citation2018). The Colombian government has worked towards the development of an agenda and adopted actions to reduce in 20% by the year 2030 the emissions of greenhouse gases, which links to the Law 1715 of 2014 that promotes (1) the integration to the national energy system of energy using renewable sources and (2) the increase in energy access of the off-grid zones and establish a suitable atmosphere for development of projects in this area (Law Citation1715 of 2014, Citation2014).
The MSW generated in developing countries have different compositions and in consequence characteristics from that of developed countries, which has an impact on the degradation processes in landfills. For example, the organic fraction, composed mainly for food and food waste, represents a significant proportion of the solid waste generated in low-income countries, of around 64%, while in high-income countries this fraction barely reaches 28% (Hoornweg & Bhada-Tata, Citation2012). Also, the lower content of paper and cardboard in MSW of developing countries has an impact on the generation of methane-enriched biogas, a compound of environmental interest not only because of its climate change potential but also for being a powerful GHG and at the same time a source of alternative energy. This could mean higher but less prolonged rates of biogas production due to the higher proportion of easily biodegradable waste and a lower content of materials with high lignocellulosic content (Zheng et al., Citation2015). The content of materials such as plastics, textiles and paper and cardboard, regarded as impermeable or partially impermeable two-dimensional materials, affect the direction of the fluid paths and create regions of preferential flow paths that may impact on the hydraulic conductivity (Caicedo, Sandoval, & Whitting, Citation2016).
Estimation of the biogas production potential is an important aspect when the viability of a biogas production project needs evaluation (UNEP and ISWA, Citation2015; Habitat, Citation2010; Purmessur & Surroop, Citation2019; Fei, Wen, & De Clercq, Citation2019). Experimentally, methods that favor anaerobic degradation conditions are commonly used. The BMP (Biochemical Methane Potential) is one of the extensive use (Pearse, Hettiaratchi, & Kumar, Citation2018; Raposo, De La Rubia, Fernández-Cegrí, & Borja, Citation2012), where anaerobic degradation conditions are achieved using bacteria and nutrients and adjustment of key operation parameters such as pH, temperature or inoculum–substrate ratio (I/S).
In studies using MSW, BMP assays have been used to evaluate the biodegradability of the organic fraction under conditions similar to those experienced in final disposal sites (Knox, Braithwaite, Caine, & Croft, Citation2005; Cossu, Lai, & Sandon, Citation2012; Binner & Zach, Citation1999). Most of those studies have been carried out using landfilled waste produced in developed countries (Pearse et al., Citation2018; Wagland, Tyrrel, Godley, & Smith, Citation2010) where either the substrate concentration or the optimal I/S, two of the most influential factors in BMP assays, have been reported (Boulanger, Pinet, Bouix, Bouchez, & Mansour, Citation2012).
Although some laboratory studies have shown differences for wet and high bio-waste fractions residues (Zheng et al., Citation2015; Sponza & Aǧdaǧ, Citation2004; Swati, Karthikeyan, Kurian, Visvanathan, & Nagendran, Citation2011), the information for fresh residues and excavated from landfills in developing countries is scarce. It is therefore necessary to investigate the potential to produce biogas using landfilled waste from developing countries and consider the differences in composition, environment and construction and operation conditions to determine reference values for the BMP that allow the estimation of the potential and give an idea of the degree of stabilization reached by the waste under the management conditions.
In this study, BMP tests were carried out to determine the potential of CH4 production to evaluate the anaerobic biodegradability of excavated MSW taken from a sanitary landfill in Valle del Cauca—Colombia.
2. Materials and methods
2.1. Waste source
The residue used for this work was excavated from a regional sanitary landfill located at the northern part of the Valle del Cauca region in Colombia, in coordinates 03° 57ʹ47.9ʹ’N; 076° 15ʹ00.57ʹ’W, which serves 25 small and medium-sized municipalities cities and receives approximately 760 tons of waste/day. The site covers over 75 hectares (ha), started operations in 1998 and to date has received over 2 million tons of MSW. The leachate collection system consists of a geo-membrane over which is placed a layer of gravel covered with geo-textile. The site has a passive landfill gas (LFG) venting system that consists of venting wells that penetrate to the bottom of the waste but does not have an existing active LFG collection and control system.
A waste sample of 200 kg was collected with the help of a backhoe at a depth of between 3 and 4 m from a cell known to have refuse 4–5 years old, closed in 2011 and covered with soil and vegetation. The area where the landfill is located has an average temperature of 23°C and a bimodal precipitation regime with peak levels of rainfall occurring both during March–May and September–November, whereas during July–August the annual precipitation can be below 1500 mm.
2.2. Waste characterization
Composition analysis was carried out on site, in a wet state to represent closely the conditions of the waste in the landfill, following methodologies for unprocessed MSW based on a quartering method (ASTM International, ASTM D5231-92, Citation2016; Ministerio de Desarrollo Económico de Colombia, RAS, Citation2000). Recognition of categories was made manually and included green waste, paper and cardboard, plastics, sanitary (diapers and sanitary towels) and textiles before the fractions were dried and following the methods reported by other authors (Pearse et al., Citation2018; ASTM International, ASTM D5231-92, Citation2016; Velkushanova, Caicedo, Richards, & Powrie, Citation2009). A category commonly found in sanitary landfills was also included, consisting of the mixture of degraded organic matter and fine material with the appearance of soil, known as non-identifiable. Food waste was not considered as a category given the age of the residue of 4 to 5 years. Removal of large volume elements and metals was carried out on site immediately after taking the sample. All the samples were immediately transported to a local laboratory. Moisture content of 44.5% was measured for the waste sample by drying at 105°C until a constant weight was reached.
Results are shown in Table where information reported by the landfill operator and statistics for colombian cities is also presented.
Table 1. Composition of excavated MSW (age waste) and reconstituted specimen as used in this study
2.3. Preparation of samples
The waste processing for the BMP tests was similar to that suggested in other studies that evaluated the biodegradability and the potential for biogas production of MSW (Kelly et al., Citation2006; Cossu & Raga, Citation2008; Zheng, Phoungthong, Lü, Shao, & He, Citation2013). The process consisted of separating and drying the biodegradable fraction (green waste, paper and cardboard, sanitary and non-identifiable waste) and then reducing their size for the tests. Green waste, paper and cardboard, sanitary and non-identifiable waste were dry (70°C), cut by hand and then shredded using a forage mill (TRF 300, Trapp, Jaraguá do Sul, Brazil). Thus, the biodegradable fractions were reduced to a particle size <12.5 mm, size chosen considering that the diameter of the reactors used during the tests were 125 mm, and that it is generally accepted that the effect of side walls are limited whenever the relation between the maximum particle size of the specimen and the diameter of the cell is 1/10 (Xie, Aldenkortt, Wagner, & Rettenberger, Citation2006) and to approximate the physical characteristics of landfill waste, which affect the movement of fluids and the distribution of solutes and biomass in landfills (Caicedo-Concha, Sandoval-Cobo, & Whiting, Citation2016).
Once sorted and dried, the size of all the fractions was reduced to less than 12.5 mm. The size of the particles of paper and cardboard, plastics, sanitary and textiles fractions were manually reduced using a knife. The fractions were vacuum-packed and maintained refrigerated to 4°C for 7 days whilst they were delivered and received in the laboratory located in Southampton, United Kingdom. Once received, they were kept at −18°C until the start of the experiments. The fractions were used for the reconstitution of the waste sample to be used in the experiments, according to the landfill composition as presented in Table .
For the reconstituted waste sample named as Aged Waste, total solids and volatile solids (TS and VS) were measured using Standard Methods 2540 G (American Public Health Association/American Water Works Association/Water Environment Federation, APHA, Citation2005). For each fraction, an elemental composition using a FlashEA 1112 Elemental Analyser (Thermo Finnigan, Italy) following the manufacturer standard procedures, with Atropine and Nicotinamide as standards for C, H and N was performed. Birch was used as a standard for sulphur determination with the addition of vanadium pentoxide catalyst and a desiccating column to remove the H peak. Results for the reconstituted aged waste were calculated using its composition as reported in Table . Results are presented in Table .
Table 2. Elemental analysis, %TS and %VS in the waste studied
2.4. Inoculum characterization
The inoculum used was from a mesophilic anaerobic digester treating municipal wastewater solids at Millbrook wastewater treatment plant, Southampton, UK and was maintained at 35°C in containers with sufficient free space to allow for degassing. Inoculum physicochemical characterization included pH, TS, and VS determinations according to APHA, 2005 (American Public Health Association/American Water Works Association/Water Environment Federation, APHA, Citation2005). The characteristics of the inoculum used are shown in Table .
Table 3. Characteristics of the inoculum used in this study
2.5. Determination of the biochemical methane potential (BPM)
Reconstituted samples following the as-placed composition reported in Table were used in the Biochemical Methane Potential (BMP) experiments. Samples of dried and shredded age MSW samples (250 g) were incubated at 35 ± 1°C using 1-l batch reactors where they were mixed with inoculum, at a I/S of 0.81 g VS inoculum/g VS substrate, which is within the range recommended by authors such as Holliger, et.al (2016) (Holliger et al., Citation2016) for the evaluation of easily degradable substrates as the residue used in this study, with minor fractions of biodegradable materials and following a procedure similar to that reported by Zhang, W. et al. (2012) (Zhang, Banks, & Heaven, Citation2012). Tests were run by triplicate during 30 days against blanks with no substrate added to determine the contribution of the inoculum on the CH4 production, time that was defined based on previous studies with excavated MSW (Kelly et al., Citation2006) (Liu, Zhang, El-Mashad, & Dong, Citation2009) and the achievement of the asymptotic phase in the cumulative production curves of CH4 (Holliger et al., Citation2016). Before the start of incubation, reactors were flushed with nitrogen to remove oxygen. Biogas was collected in 1.9-L cylinders filled with an acidified sodium chloride solution (pH < 2).
The bioreactors were coupled with agitators that were turned on every 3 days and operated for 5 min each time. The measured volume of biogas and CH4 produced was corrected for the gas produced from the blank reactors containing only anaerobic biosolids. Samples of biogas were taken periodically and their composition measured by gas chromatography in a Varian star 3400 CX Chromatograph using a mixed gas standard of 65% CH4 and 35% CO2 (v/v) for calibration (BOC, UK). Cumulated volumes of biogas and CH4 produced were reported for a dry gas at standard conditions of temperature (0°C) and pressure (1 atm).
3. Results and discussion
3.1. Waste characterisation
Table shows the physical composition for the aged waste used in this study, and well as the historic information of the waste landfilled in the site and the average composition reported in Colombian cities. For comparison purposes, Figure presents the composition of the aged waste sampled for this study and that taken of a fresh waste sample under similar conditions before being disposed of in the landfill site.
Figure 1. Composition of aged waste landfilled (left) as used in the experiments and fresh landfilled waste for comparison purposes (right)
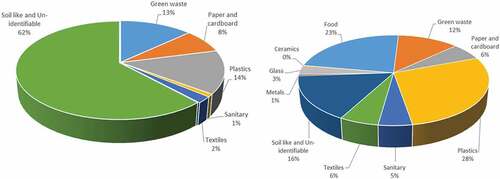
It can be seen that on dry base green waste, paper and cardboard and textiles (8.2%, 6.1% and 3.1%, respectively) were identified despite the 4 to 5 years of degradation of the waste. It was also identified a high content of non-easily identifiable materials (65.5% on dry base), this is a well-degraded material characterized by soil-like and putrescible fractions difficult to associate with a specific category of waste, which constitutes the greatest proportion and it is similar to that reported by other authors (Machado, Karimpour-Fard, Shariatmadari, Carvalho, & Do Nascimento, Citation2010)(Quaghebeur et al., Citation2013)(Garcia et al., Citation2016).
Plastic (e.g., plastic bags, packaging material, bottles and containers) was the second-largest category (16% on dry basis), represents a high proportion of MSW in developing countries and has low biodegradability (EPA, Citation2014; Shah, Hasan, Hameed, & Ahmed, Citation2008). In Colombia, its value as a recyclable material is low due to contamination and its low weight/volume ratio, so plastics currently are disposed of in landfills (Vergara, Damgaard, & Gomez, Citation2016). Important amounts of sanitary waste (1.1% on dry basis), mostly toilet paper, was found in the studied waste, similar to what has been reported for MSW produced in emerging economies such as China (Zheng et al., Citation2015; Yang, Damgaard, Scheutz, Shao, & He, Citation2018). Zheng et al. (2013) found that toilet paper has similar biodegradability to that of most food waste fractions and higher than that of office paper or yard waste (Zheng et al., Citation2013). Therefore, it is possible that putrescible materials originating from food waste, and to a lesser extent, moderate biodegradable residues such as toilet paper and green waste, are responsible for the greater generation of CH4 in landfills in developing countries. Behaviour that differs from that of developed countries, in which more than 90% of the methanogenic potential comes from materials rich in cellulose and hemicellulose, such as paper and cardboard (Barlaz, Ham, & Schaefer, Citation1989), possibly due to the fact that content of such materials in the MSW in developed countries is approximately 31%, whereas it is only 5% in developing countries (Hoornweg & Bhada-Tata, Citation2012).
Table shows the results obtained for the elemental analysis. It can be seen how the carbon content, limiting reactant for the production of CH4, varies from 44.04% in the textile fraction to 8.61% in the non-identifiable fraction. Textiles, green waste and paper and cardboard fractions, given their carbon content (44.04%, 26.58% and 19.44%, respectively), contribute the most to the production of biogas in landfills, whilst plastics and non-identifiable fractions (14.85% and 8.61%, respectively) can be considered as a retardant for the production of biogás. VS analysis of the non-identifiable fraction shows a content of only 18.6% for volatile solids based on total solids, suggesting that such a fraction does not contribute importantly to the biogas production in landfills. This figure is similar to that reported by Machado et al. (2009) (Machado, Carvalho, Gourc, Vilar, & Do Nascimento, Citation2009) in the range of 16.0–23.2% for an aged waste being disposed of between 3.9 and 8.8 years collected from a landfill in Brazil. Carbon content estimation in the reconstituted aged waste is around 15% (15.55%) based on the measured compositions reported in Table . Although several authors agree that VS values provide an indication of the biodegradability of several organic substrates (Raposo et al., Citation2012; Campuzano & González-Martínez, Citation2016), their values do not necessarily reflect the biodegradability of MSW, especially for waste disposed of in landfills that is highly heterogeneous and combines materials with rapid and slow degradation rates (Garcia et al., Citation2016).
3.2. Biochemical methane potential tests
Table and Figure summarize the main results obtained for these experiments, the samples of aged waste are identified as Ag 1, Ag 2 and Ag 3. Figure presents the cumulative volume of CH4 produced in each of the experiments. Biogas and methane production results were within the range reported in BMP tests using landfilled MSW with similar characteristics for waste disposed of in landfills in other low- and middle-income countries (Bilgili, Demir, & Varank, Citation2009; Machado et al., Citation2009; Ahmadifar, Sartaj, & Abdallah, Citation2015). Biogas production of 49.2–56.8 L kg-1 of dry matter (DM) and methane yields in the range of 34.8−37.9 L CH4 kg-1 DM were obtained by the end of the tests. Methane content increased from 0% to 42.2%vol. over the first 15 days, indicating a relatively early adaptation of the microbial population to methanogenic growth conditions.
Table 4. Results of biogas and methane production obtained in this study
The latency phase for the aged waste was of approximately 8 h, this is the time to exceed the biogas production over that produced by the inoculum, which suggests a rapid start of CH4 generation probably associated with the composition of the waste, a substrate of rapid assimilation and the agitation carried out every 3 days to the bioreactors (Francois, Feuillade, Skhiri, Lagier, & Matejka, Citation2006). The activity of the inoculum and its characteristics generally influence the process and production of CH4, which in this case was accounted for in the calculations. The curves indicate that when the time for testing (30 days) was completed, the cumulative production of CH4 had slow down and moving towards being stable.
The methane potential of the waste disposed of in landfills depends on MSW composition and on the environmental and operating conditions of the disposal sites. Other studies characterizing the BMP of landfill MSW recommend that this potential should be managed to improve the environmental performance of landfills during their active operational phase and to enhance solid waste stabilization that helps to reduce both long-term CH4 emissions and post-closure management costs for landfills (Scharff, van Zomeren, & van der Sloot, Citation2011).
An accurate estimation of landfills methane generation requires the consideration of several factors, not only the potential of biogas, as it is as discussed by (Purmessur & Surroop, Citation2019; Fei et al., Citation2019). Factors affecting that estimation include waste composition and variations over time, environmental, climatic and operational management conditions and rates of waste disposal, among others. Quantities, as well as the composition, are important factors to assess. This evaluation needs to consider variations over time, as consumption patterns have seen lately variations in Colombia, leading to changes in rates of landfill gas generation and in the net amount of landfill methane produced (Kaza et al., Citation2018; S. de S. P. D. Superservicios, Citation2018). The quantity of waste being disposed of in landfill sites has also seen variations since the country is encouraging the operation of regional sites over local sites in an effort to increase operational efficiency (S. de S. P. D. Superservicios, Citation2018). Site-specific environmental conditions, such as precipitation, need special consideration in landfills located in tropical regions, moreover where it is expected that climate change will have serious impacts in Colombia (IPCC Intergovernmental Panel on Climate Change, Citation2014). Operational management condition is another factor that has an impact on the rate of landfill gas generation and capture, as it has been pointed by some studies (Sponza & Aǧdaǧ, Citation2004; Francois et al., Citation2006; Bilgili et al., Citation2009).
Some tools used for the estimation of landfill gas produced in landfills include first-order decay models such as Landgem (Reinhart, Faour, & You, Citation2005) and IPCC (Eggleston, Buendia, Miwa, Ngara, & Tanabe, Citation2008) models, which take into consideration time factors of the degradation process such as the fraction of degradable carbon, the effects of temperature, pH, moisture and waste composition as well as a methane correction factor to account for site-specific management and operational conditions among others (Fei et al., Citation2019). First-order decay models have been used together with BMP measurements to determine appropriate gas generation parameters for landfills (Machado et al., Citation2009) (Kim & Townsend, Citation2012). This type of approach, however, requires spatio-temporal analysis of waste degradation characteristics, generally involving BMP characterisation for waste samples of different ages and, ideally, spatially distributed within the landfill in order to account for landfills heterogeneity.
It is likely that in landfills in tropical developing countries such as Colombia, the generation rates of CH4 in the final stages of degradation are lower than those in developed countries, mainly due to the higher contents of putrescible materials originating from food waste and materials with low lignocellulosic contents, as shown by (Zheng et al., Citation2015) for landfill waste in China. Nevertheless, it must be taken into account that biodegradability tests such as BMP experiments fail to represent other factors that affect the degradation processes in dynamic and highly heterogeneous systems such as landfills (Pearse et al., Citation2018). Therefore, in addition to assessing the biodegradability and the CH4 potential of waste with different ages and compositional characteristics, it is advisable to perform larger tests to evaluate aspects related to the specific environmental and operational conditions of the landfills in developing countries.
4. Conclusions
The composition of the aged waste excavated from the landfill consisted primarily of fine materials with the appearance of soil and highly degraded organic matter (65.5% dry base), plastics (16%), green waste (8.2%) and paper and cardboard (6.1%). Textiles (3.1%), as well as sanitary residues mainly in the form of toilet paper (1.1%), were also identified.
The short latency periods observed during the Biochemical Methane Potential (BMP) tests suggest a rapid establishment of methanogenic degradation conditions, which may be associated with the high proportion of easily assimilated organic materials and the affinity of the inoculum biomass for the substrates. The BMP values obtained are similar to those reported in other studies with aged waste excavated from landfills. These results demonstrate the potential for CH4 utilization from landfills in tropical developing countries such as Colombia. Depending on its management, the CH4 could be a significant source of GHG emissions or an alternative energy source that contributes to improving the environmental performance of final disposal sites in developing countries. These findings can give valuable insight into the real potential of biogas and CH4 generation from the un-segregated MSW produced and disposed of at landfills in developing countries; also can potentially contribute to a better assessment of the recovery potential, treatment and utilization schemes for landfill gas in developing countries like Colombia.
Acknowledgements
Support for carrying out this research was given from the Newton Caldas Research Links BC 028-EDU2016 and the cooperation between the Universidad Cooperativa de Colombia and Universidad del Valle through the joint research project “Adding value to municipal solid waste through the production of biogas-call 2016”. The authors also acknowledge the contribution of COST Action FP1306 via promoting networking and collaboration.
Additional information
Funding
Notes on contributors
Diana M. Caicedo-Concha
Diana M. Caicedo is a lecturer in Industrial and Environmental Engineering and researcher at the Universidad Cooperativa de Colombia. Her research interests are related to sustainable energies, solid waste management and solid waste valorization. Her current research projects include the study of opportunities to add value to domestic and rural wastes and the application of sustainable energy solutions to vulnerable zones.
John J. Sandoval-Cobo
John Jairo Sandoval-Cobo is a MSc in Civil and Environmental Engineering currently conducting doctoral research on sustainable management alternatives for landfills as a member of the Study and Control of Environmental Pollution research group (ECCA) at Universidad del Valle. His research experience include solid and wastewater treatment using sustainable technologies, ground water modeling and remediation of contaminated sites and industrial effluents.
References
- Agudelo Vélez, M. I., Chavarro Bohorquez, D. A., Hernández Tasco, A., Niño Mendieta, A. M., Tovar Narváez, G.E. & Montenegro Trujillo,I. C. (2018). Green book 2030: national science and innovation policy for sustainable development. Bogotá, Colombia: Colciencias.
- Ahmadifar, M., Sartaj, M., & Abdallah, M. (2015). Investigating the performance of aerobic, semi-aerobic, and anaerobic bioreactor landfills for MSW management in developing countries. J. Mater. Cycles Waste Manag., 18(4):703–714
- American Public Health Association/American Water Works Association/Water Environment Federation, APHA. (2005). Standard methods for the examination of water and wastewater.21st Edition. Washington, DC, USA.
- ASTM International, ASTM D5231-92. (2016). Standard test method for determination of the composition of unprocessed municipal solid waste. West Conshohocken, PA,USA: ASTM International.
- Barlaz, M., Ham, R., & Schaefer, D. (1989, Dec). Mass balance analysis of anaerobically decomposed refuse. Journal of Environmental Engineering, 115(6), 1088–14. doi:10.1061/(ASCE)0733-9372(1989)115:6(1088)
- BID. (2015). Estudio tecnologías alternativas de disposición final o aprovechamiento de residuos sólidos. Propuesta de ajuste al Decreto 838 de 2005. Bogotá, DC: Banco Interamericano de Desarrollo (BID).
- Bilgili, M. S., Demir, A., & Varank, G. (2009). Evaluation and modeling of biochemical methane potential (BMP) of landfilled solid waste: A pilot scale study. Bioresource Technology, 100(21), 4976–4980. doi:10.1016/j.biortech.2009.05.012
- Binner, E., & Zach, A. (1999). Laboratory tests describing the biological reactivity of pretreated residual wastes, In Proceedings of Symposium ORBIT 99 on Organic Recovery and Biological Treatment Symposium, Weimar, Germany.
- Boulanger, A., Pinet, E., Bouix, M., Bouchez, T., & Mansour, A. A. (2012). Effect of inoculum to substrate ratio (I/S) on municipal solid waste anaerobic degradation kinetics and potential. Waste Management, 32(12), 2258–2265. doi:10.1016/j.wasman.2012.07.024
- Caicedo, D., Sandoval, J., & Whitting, K. (2016). An experimental study on the impact of two dimensional materials in waste disposal sites: What are the implications for engineered landfills? Sustainable Environment Research, 26(6), 255–261. doi:10.1016/j.serj.2016.08.001
- Caicedo-Concha, D. M., Sandoval-Cobo, J. J., & Whiting, K. (2016). An experimental study on the impact of two dimensional materials in waste disposal sites: What are the implications for engineered landfills? Sustainable Environment Research, 26(6), 255–261. doi:10.1016/j.serj.2016.08.001
- Campuzano, R., & González-Martínez, S. (2016). Characteristics of the organic fraction of municipal solid waste and methane production: A review. Waste Management, 54, 3–12. doi:10.1016/j.wasman.2016.05.016
- COLOMBIA, C.D. (2014). Ley 1715 de 2014, Regulación de la integración de las energías renovables no convencioanles al Sistema Energético Nacional. Diario Oficial No. 49.150. Imprenta Nacional: Bogotá D.C.
- Cossu, R., Lai, T., & Sandon, A. (2012). Standardization of BOD 5/COD ratio as a biological stability index for MSW. Waste Management, 32(8), 1503–1508. doi:10.1016/j.wasman.2012.04.001
- Cossu, R., & Raga, R. (2008). Test methods for assessing the biological stability of biodegradable waste. Waste Management, 28(2), 381–388. doi:10.1016/j.wasman.2007.01.014
- DNP. (2016). CONPES 3874. Pólítica Nacional para la Gestión Integral de Residuos Sólidos. Bogotá, DC: Departamento Nacional de Planeación (DNP).
- Eggleston, S., Buendia, L., Miwa, K., Ngara, T., & Tanabe, K., “IPCC 2008, 2006 IPCC Guidelines for National Greenhouse Gas Inventories,” 2008.
- EPA, U. S. (2014). Municipal solid waste generation, recycling, and disposal in the United States tables and figures for 2012,” Office of Resource Conservation and Recovery. Retrieved from http://www.epa.gov/epawaste/nonhaz/municipal/pubs/2012_msw_dat_tbls.pdf.
- Fei, F., Wen, Z., & De Clercq, D. (2019). Spatio-temporal estimation of landfill gas energy potential: A case study in China. Renewable and Sustainable Energy Reviews, 103, 217–226. doi:10.1016/j.rser.2018.12.036
- Francois, V., Feuillade, G., Skhiri, N., Lagier, T., & Matejka, G. (2006). Indicating the parameters of the state of degradation of municipal solid waste. Journal of Hazardous Materials, 137, 1008–1015. doi:10.1016/j.jhazmat.2006.03.026
- Garcia, J., Davies, S., Villa, R., Gomes, D. M., Coulon, F., & Wagland, S. T. (2016). Compositional analysis of excavated landfill samples and the determination of residual biogas potential of the organic fraction. Waste Management, 55, 336–344. doi:10.1016/j.wasman.2016.06.003
- HABITAT, U. N. (2010). Solid Waste Management in the World’s Cities: Water and Sanitation in the World’s Cities 2010.
- Holliger, C., Alves, M., Andrade, D., Angelidaki, I., Astals, S., Baier, U., Bougrier, C., ... Wierinck, I. (2016). Towards a standardization of biomethane potential tests. Water Science and Technology, 74(11), 2515–2522.
- Hoornweg, D., & Bhada-Tata, P. (2012). What a waste: A global review of solid waste management. World Bank, Washington, DC.
- IPCC Intergovernmental Panel on Climate Change. 2014. Climate change 2014 impacts, adaptation, and vulnerability part B: Regional aspects.
- Ivan, C., María, T., Aura, V., Paola, A., & Mario, H. (2016). Anaerobic co-digestion of organic residues from different productive sectors in Colombia: Biomethanation potential assessment. Chemical Engineering Transactions, 49, 385–390.
- Kaza, S., Yao, L., Bhada-Tata, P., & Van Woerden, F. (2018). What a waste 2.0: a global snapshot of solid waste management to 2050. World Bank Publications
- Kelly, R. J., Shearer, B. D., Kim, J., Goldsmith, C. D., Hater, G. R., & Novak, J. T. (2006). Relationships between analytical methods utilized as tools in the evaluation of landfill waste stability. Waste Management, 26(12), 1349–1356. doi:10.1016/j.wasman.2005.11.019
- Kim, H., & Townsend, T. G. (2012). Wet landfill decomposition rate determination using methane yield results for excavated waste samples. Waste Management, 32(7), 1427–1433. doi:10.1016/j.wasman.2012.03.017
- Knox, K., Braithwaite, P., Caine, M., & Croft, B. (2005). Brogborough landfill test cells: The final chapter. A study of landfill completion in relation to final storage quality (FSQ) criteria. In S. Margherita di Pula (ed.,) Sardinia 2005 – 10th International waste management and landfill symposium. Cagliari, Italy.
- Larochelle, L., Turner, M., & LaGiglia, M. (2012). Evaluation of NAMA opportunities in Colombia’s solid waste sector. Washington, DC: Center for clean air policy.
- Lima, R. M., Santos, A. H. M., Pereira, C. R. S., Flauzino, B. K., Pereira, A. C. O. S., Nogueira, F. J. H., Valverde, J. A. R. (2018). Spatially distributed potential of landfill biogas production and electric power generation in Brazil. Waste Management, 74, 323–334. doi:10.1016/j.wasman.2017.12.011
- Liu, G., Zhang, R., El-Mashad, H. M., & Dong, R. (2009). Effect of feed to inoculum ratios on biogas yields of food and green wastes. Bioresource Technology, 100(21), 5103–5108. doi:10.1016/j.biortech.2009.03.081
- Machado, S., Carvalho, M. F., Gourc, J. P., Vilar, O. M., & Do Nascimento, J. C. F. (2009). Methane generation in tropical landfills: Simplified methods and field results. Waste Management, 29(1), 153–161. doi:10.1016/j.wasman.2008.02.017
- Machado, S. L., Karimpour-Fard, M., Shariatmadari, N., Carvalho, M. F., & Do Nascimento, J. C. F. (2010, Dec). Evaluation of the geotechnical properties of MSW in two Brazilian landfills. Waste Management, 30(12), 2579–2591. doi:10.1016/j.wasman.2010.07.019
- Ministerio de Desarrollo Económico de Colombia. (2000). Reglamento Técnico del Sector de Agua Potable y Saneamiento Básico- RAS 2000: Titulo F. Ministerio de Desarrollo Económico de Colombia.
- Pearse, L. F., Hettiaratchi, J. P., & Kumar, S. (2018). Towards developing a representative biochemical methane potential (BMP) assay for landfilled municipal solid waste – A review. Bioresource Technology, 254, 312–324. doi:10.1016/j.biortech.2018.01.069
- Pedraza, A., Cabrera, M., Duarte, M., Gutiérrez, M., Lamprea, P., & Lozano, R. (2005). Visión general del inventario nacional de emisiones de gases de efecto de invernadero. In Inventario nacional de emisiones de gases de efecto de invernadero 2002-2004. Segunda comunicación nacional ante la Convención Marco de las Naciones Unidas sobre el cambio climático (pp. 17–66). Bogotá, D. C: IDEAM..
- Piñas, J. A. V., Venturini, O. J., Lora, E. E. S., de Oliveira, M. A., & Roalcaba, O. D. C. (2016). Landfills for electricity generation from biogas production in Brazil: Comparison of LandGEM (EPA) and Biogas (Cetesb) models | Aterros sanitários para geração de energia elétrica a partir da produção de biogás no Brasil: Comparação dos modelos LandGEM (E. Rev. Bras. Estud. Popul., 33(1), 175–188. doi:10.20947/S0102-309820160009
- Purmessur, B., & Surroop, D. (2019). Power generation using landfill gas generated from new cell at the existing landfill site. Journal of Environmental Chemical Engineering, 7(3), 103060. doi:10.1016/j.jece.2019.103060
- Quaghebeur, M., Laenen, B., Geysen, D., Nielsen, P., Pontikes, Y., Van Gerven, T., & Spooren, J. (2013). Characterization of landfilled materials: Screening of the enhanced landfill mining potential. Journal of Cleaner Production, 55, 72–83. doi:10.1016/j.jclepro.2012.06.012
- Raposo, F., De La Rubia, M. A., Fernández-Cegrí, V., & Borja, R. (2012). Anaerobic digestion of solid organic substrates in batch mode: An overview relating to methane yields and experimental procedures. Renewable and Sustainable Energy Reviews, 16(1), 861–877. doi:10.1016/j.rser.2011.09.008
- Reinhart, D. R., Faour, A. A., & You, H. (2005). First order kinetics gas generation model parameters for wet landfills. Washington, DC: US. Environmental protection agency.
- S. de S. P. D. Superservicios, “Informe de Disposición Final de Residuos Sólidos – 2017,” 2018.
- Scharff, H., van Zomeren, A., & van der Sloot, H. A. (2011). Landfill sustainability and aftercare completion criteria. Waste Manag. Res., 29(1), 30–40. doi:10.1177/0734242X10384310
- Shah, A. A., Hasan, F., Hameed, A., & Ahmed, S. (2008). Biological degradation of plastics: A comprehensive review. Biotechnology Advances, 26(3), 246–265. doi:10.1016/j.biotechadv.2007.12.005
- Sponza, D. T., & Aǧdaǧ, O. N. (2004). Impact of leachate recirculation and recirculation volume on stabilization of municipal solid wastes in simulated anaerobic bioreactors. Process Biochemistry, 39(12), 2157–2165. doi:10.1016/j.procbio.2003.11.012
- Swati, M., Karthikeyan, O., Kurian, J., Visvanathan, C., & Nagendran, C. (2011). Pilot-scale simulation of landfill bioreactor and controlled dumping of fresh and partially stabilized municipal solid waste in a tropical developing country. Journal of Hazardous, Toxic, and Radioactive Waste, 15(October), 321–330. doi:10.1061/(ASCE)HZ.1944-8376.0000081
- Themelis, N. J., Elena, M., Barriga, D., Estevez, P., & Velasco, M. G., “Guidebook for the application of waste to energy technologies in Latin America and the Caribbean,” 2013.
- UNEP and ISWA. (2015). The Global Waste Management Outlook (GWMO).
- United Nations Department of Economic and Social Affairs (UN DESA). (2018). Sustainable Development Goals Report 2018. p. 64,
- USEPA/ISWA. (2012). International Best Practices Guide for Landfill Gas Energy Project.
- Velkushanova, W., Caicedo, K., Richards, D., & Powrie, D. J. (2009). A detailed characterisation of an MBT waste. In Sardinia 2009, Twelfth International Waste Management and Landfill Symposium.S. Margherita di Pula, Cagliari, Italy
- Vergara, S. E., Damgaard, A., & Gomez, D. (2016). The efficiency of informality: quantifying greenhouse gas reductions from informal recycling in Bogotá, Colombia. Journal of Industrial Ecology, 20(1), 107–119. doi:10.1111/jiec.12257
- Wagland, S. T., Tyrrel, S. F., Godley, A., & Smith, R. (2010). Test methods to aid in the evaluation of the diversion of biodegradable municipal waste (BMW) from landfill. Waste Management, 30(5), 934–935. doi:10.1016/j.wasman.2010.01.016
- Wilson, D. C., Rodic, L., Cowing, M. J., Velis, C. A., Whiteman, A. D., Scheinberg, A., … Oelz, B. (2015). ‘Wasteaware’ benchmark indicators for integrated sustainable waste management in cities. Waste Management, 35, 329–342. doi:10.1016/j.wasman.2014.10.006
- Xie, M., Aldenkortt, D., Wagner, J.-F., & Rettenberger, G. (2006). Effect of plastic fragments on hydraulic characteristics of pretreated municipal solid waste. Can. Geotech. J., 43(12), 1333–1343. doi:10.1139/t06-070
- Yang, N., Damgaard, A., Scheutz, C., Shao, L. M., & He, P. J. (2018). A comparison of chemical MSW compositional data between China and Denmark. Journal of Environmental Sciences, 74, 1–10. doi:10.1016/j.jes.2018.02.010
- Zhang, Y., Banks, C. J., & Heaven, S. (2012). Anaerobic digestion of two biodegradable municipal waste streams. Journal of Environmental Management, 104, 166–174. doi:10.1016/j.jenvman.2012.03.043
- Zheng, W., Lü, F., Bolyard, S. C., Shao, L., Reinhart, D. R., & He, P. (2015). Evaluation of monitoring indicators for the post-closure care of a landfill for MSW characterized with low lignin content. Waste Management, 36, 222–229. doi:10.1016/j.wasman.2014.10.031
- Zheng, W., Phoungthong, K., Lü, F., Shao, L.-M., & He, P.-J. (2013). Evaluation of a classification method for biodegradable solid wastes using anaerobic degradation parameters. Waste Management, 33(12), 2632–2640. doi:10.1016/j.wasman.2013.08.015