Abstract
Cities that expand and grow with new areas allowed for construction as a result of a rapidly increasing population have become the centre of global energy consumption. Most of the energy consumed is still based on fossil fuels, which is considered the most important cause of the rise in greenhouse gases and thus the deepening climate crisis. Therefore, the transformation of urban areas into energy-efficient and low-carbon cities has become a necessity to create a sustainable future. The climate data-based evaluation of the relationship between buildings, which have the largest energy-saving potential, and urban geometry, which affects the energy performance of buildings in the long term, is crucially important. This study aims to evaluate the potential impacts of climate change on the energy performance of buildings at the settlement scale. For this purpose, changes in building energy performance within different settlement pattern alternatives created for Istanbul (a temperate-humid climate), where new settlement patterns are rapidly developed, were evaluated according to present and future weather files. The evaluation results have demonstrated that sustainable energy use in urban areas is only possible through the integration of a building’s energy performance, urban geometry and climate change in the planning and design stages.
PUBLIC INTEREST STATEMENT
With the rapid growth of urban areas all around the world, settlement patterns are considered to be the main source of CO2 emissions responsible for climate change. Therefore, settlement patterns and buildings in these areas should be planned, developed and used optimally in relation to energy consumption and associated CO2 emissions to create a sustainable future. The climate data-based evaluation of the relationship between buildings, which have the largest energy-saving potential, and urban geometry, which affects the energy performance of buildings in the long term, is crucially important. This study aims to carry out a parametric analysis to determine the potential impacts of climate change on the energy performance of buildings at the settlement scale.
1. Introduction
Today, creating a continuous and sustainable energy supply to meet the development needs of countries with an increasing population and rising urbanization is the most important problem to be solved. As the relation between rapid urbanization and energy efficiency has not yet been established, climate change issues that negatively affect quality of life and the global economy have become an important international discussion. Therefore, it is now a major turning point in the fight against global climate change in the 21st century.
As the negative effects of climate change are felt most profoundly in cities, it is essential to evaluate the extent of the impact, taking into consideration urbanization and future weather files. While more than half of the global population lives in urban areas, 2.5 billion more people will be added to the urban population by 2050 (UNFCCC, Citation2018). Furthermore, it is estimated that the global average temperature has increased 1.0°C from the pre-industrial period due to human activities; based on this trend, the average temperature will increase 1.5 °C between the years 2030–2052 since the pre-industrial period (IPCC, Citation0000). Therefore, serious problems are inevitable as a result of increasing energy consumption per person, the associated CO2 emission levels and the insistence on energy policies based on fossil fuels. Urban areas are considered the most important factor in finding a solution to climate change caused by urban development dynamics. In this regard, buildings in urban areas, which account for three-fourths of global primary energy use and approximately 60% of greenhouse gas emissions in the world (UN-Habitat, Citation0000), are of critical importance. Building and construction sectors in developing and developed countries account for 36% of global final energy consumption and approximately 40% of total direct and indirect CO2 emissions. The amount of energy required for buildings—and the construction of new buildings—is forecasted to increase around 3% every year due to several factors, such as the rapid increase in building floor areas and the use of energy-consuming devices (IEA, Citation2018).
When creating a sustainable future, changing buildings that have the highest energy-saving potential into highly efficient structures with less energy consumption—and therefore less CO2 emissions and energy costs—is considered one of the fastest and most cost-efficient solutions. In this regard, the evaluation of buildings not as standalone structures but as a part of settlement patterns, which affect the energy performance of buildings for many years without any major change, result in more informed decisions being made to create sustainably built environments and increase energy conservation (Ali-Toudert, Citation2009; Bakker & Steemers, Citation2000; Ewing & Rong, Citation2008; Hachem, Athienitis, & Fazio, Citation2011; Ratti, Baker, & Steemers, Citation2005; Ratti, Raydan, & Steemers, Citation2003; Shang, Lin, & Hou, Citation2013; Strømann-Andersen & Sattrup, Citation2011; Taleghani, Tenpierik, Van den Dobbelsteen, & de Dear, Citation2013; Van Esch, Looman, & de Bruin-hordijk, Citation2012). Additionally, considering that the lifespan of a building is 50 years or longer, analysis of how buildings will react to climate change in the future and the potential change in their energy consumption is also important (Bulkeley & Tuts, Citation2013; Dhar & Khirfan, Citation2017; Shibuya & Croxford, Citation2016; Smith & Levermore, Citation2008; Zhai & Helman, Citation2019). When the relation between urban areas and climate is examined, it is possible to conclude that design parameters, including the settlement pattern, building form, building envelope and landscape planning, have an impact on the climate in urban areas—and urban climate has an impact on the energy performance of buildings and the use of urban open spaces (Bakker & Steemers, Citation2000; Ratti et al., Citation2003, Citation2005; Ewing & Rong, Citation2008; Ali-Toudert, Citation2009; Strømann-Andersen & Sattrup, Citation2011; Hachem et al., Citation2011; Van Esch et al., Citation2012; Taleghani et al., Citation2013; Shang et al., Citation2013; Smith & Levermore, Citation2008; Bulkeley & Tuts, Citation2013; Shibuya & Croxford, Citation2016; Dhar & Khirfan, Citation2017; Zhai & Helman, Citation2019; Oke, Citation1988; Bourbia & Awbi, Citation2004; Höppe, Citation2002; Toudert & Helmut, Citation2006; Ali-Toudert & Mayer, Citation2007; Wong et al., Citation2011; Andreou, Citation2013; Kariminia, Ahmada, & Saberi, Citation2015). Therefore, the relation between the settlement pattern and a building’s energy consumption underlines a complex balance that includes climate parameters and urban canyons defined as the space above the street and between the buildings. The relationship between different settlement patterns and building energy performance should be analysed in detail during the design stage, taking into consideration present and future weather files.
When life cycle environmental impacts of buildings are examined, they are mostly due to heating and cooling energy consumption during the operational stage of buildings (Alves, Duarte, & Gonçalves, Citation2016; UNEP, Citation0000; Vianna, da Veiga, & Abranches, Citation2009). Global warming is anticipated to increase the amount of energy required to meet thermal comfort conditions in buildings, thereby having a negative effect on energy performance (Cartalis, Synodinou, Proedrou, Tsangrassoulis, & Santamouris, Citation2001; Kapsomenakis, Kolokotsa, Nikolaou, Santamouris, & Zerefos, Citation2013; Lam, Tang, & Li, Citation2008; Lam, Wan, Lam, & Wong, Citation2010; Santamouris, Citation2016). Increased energy consumption in buildings will lead to a rise in global warming, resulting in even higher energy consumption levels. To find a solution to this vicious cycle without compromising the ability of future generations to meet their needs, the use of sustainable energy should be encouraged. Studies have shown that an increase or decrease in energy consumption has a varying effect on climate change, depending on the climate characteristics of the region in which the study was conducted (Lam et al., Citation2008, Citation2010). Increased cooling needs in buildings and the consumption of more energy for air-conditioning will lead to more CO2 emissions, which in turn will accelerate climate change. Even if there is a significant reduction in heating energy consumption in cold climate regions, the increase in cooling energy consumption will become more important on the final energy consumption (or the energy delivered to buildings). That is why the impact of climate change on total primary energy requirements is still uncertain: the effects of climate change vary drastically on a spatial and temporal scale.
Relying solely on technological developments to solve these serious problems caused by climate change will be insufficient. Therefore, it is necessary to adapt buildings to provide indoor and outdoor thermal comfort conditions passively in order to reduce or eliminate negative effects of climate change on their energy performance. Settlement pattern design, which is one of the most important factors in achieving thermal comfort conditions using passive methods, should be taken into account when adapting these buildings. Considering that urban renewal occurs quickly, especially in developing countries, and decisions regarding settlement and building designs continue to affect energy performance of buildings for many years, comparing and evaluating energy analysis findings using present and future weather files and an effective roadmap is necessary.To achieve this, the appropriate values for design parameters used in sustainable settlements and buildings must be determined. Accordingly, this study aims to investigate changes in a building’s energy performance within different settlement pattern alternatives, based on future climate data using the comparative method. For this purpose, Turkey was selected, as it is one of the regions to be affected most by global climate change—and a developing country with a growing building construction sector. The analysis was designed and carried out for Istanbul, where new settlement patterns (in which energy-efficient approaches are being disregarded) are rapidly developed.
2. Methodology
It is evident that the anticipated increase in both mean and extreme temperatures due to climate change will cause an increase in energy consumption to achieve indoor thermal comfort conditions in buildings designed under current standards. Therefore, this study recommends an approach in which the impacts of climate change on building energy performance are investigated using present and future weather files in the context of settlement patterns. Appropriate values for design parameters used in sustainable settlements and buildings will be determined based on present and future weather files.
The approach addresses the following objectives:
to identify design parameters of the reference building
to identify design parameters of the settlement pattern
to develope settlement pattern alternatives
to identify weather files
to evaluate the energy performance of the building within each settlement pattern alternative, using present and future weather files
Since Turkey is largely dependent on import energy sources and now faces energy demands that continue to rise above the global average, mitigating or preventing the effects of climate change is crucial to reducing energy use in their economy. Therefore, with this approach in mind, a parametric analysis was carried out for Istanbul (a temperate-humid climate) where new settlement patterns are being built rapidly.
2.1. Identifying design parameters of the reference building
Detailed analyses and information based on statistical data are necessary to identify a reference building. However, it is not possible to determine a nationwide reference residential building due to the limited statistics on existing building stock in Turkey. Conversely, when comparing building permits granted in the first nine months of 2017 and those during the same period in 2010 in statistics published by the Turkish Statistical Institute (TUIK), the number of buildings increased by 72% to 125,846, the floor area increased by 147% to 222,439,778 m2, and the number of apartments increased by 136% to 1,106,389 (TSI, Citation2011, Citation2017). According to building permits granted in 2017, the floor area in residential buildings accounted for 58% of the total floor area of all buildings, and residential buildings with the highest percentages contained two or more housing units (TSI, Citation2017). Furthermore, one of the most important changes in the last 10 years in the building sector of Turkey is the increment of public/mass housing projects by the Housing Development Association (TOKI) throughout the country. When the high number of mass housing projects were reviewed, modules with a floor area of 100 m2 were used as the reference residential building. Two different types of plans were selected (Figure ):
the first had a square building floor plan, which was created using four modules with a form factor (“length:depth ratio” of the building) of 1.00
the second had a rectangle building floor plan, which was created using two modules with a form factor of 2.00.
In terms of the building height, a five-storey residential building, which was representative of the residential buildings commonly built in Istanbul, was evaluated. The information and values of the building, occupant and active building systems, which were used to determine the reference residential building, are shown in Table . Developing details for the layering of the building envelope and the building envelope cross-sections—commonly used in existing mass housing projects—were used, and the total heat transfer coefficient values (U, W/m2K) of the opaque and transparent components of the building envelope complied with the limit values set for Istanbul in TS 825 (Turkish Standards Institution, Citation2013) (Table ).
Table 1. Characteristics of the reference residential building
Table 2. Characteristics of the reference residential building elements
2.2. Identifying design parameters of the settlement pattern
Various settlement patterns were created using height-to-width (H/W) ratios typically used to define urban geometries (Ahmad, Khare, & Chaudhr, Citation2005; Ali-Toudert, Citation2009; Bourbia & Awbi, Citation2004; Oke, Citation1988; Strømann-Andersen & Sattrup, Citation2011; Van Esch et al., Citation2012), and the effects of such urban geometries were analysed to access outdoor thermal comfort levels together with settlement pattern/building interactions. Since Istanbul is in a temperate-humid region, the heating effects of solar radiation during the heating period was significant. The depth of shadow on January 21st was calculated, and the optimum space between buildings (in which buildings do not cast a shadow onto other buildings in the settlement pattern) was found at 1.86 times of building height between 11:00–13:00 (Table ). Therefore, in order to develop different urban geometries, the H/W ratio is considered to be 1.00 for uniform canyon, 0.50 for shallow canyon and 2.00 for deep canyon (Ahmad et al., Citation2005). The street width for each of these three H/W values was calculated according to the building height of the five-storey building (15 m) used in the study. The street on which the reference building is located is oriented east-west (0°), northeast-southwest (45°), north-south (90°) and northwest-southeast (135°), alternatively by rotating 45° in order to determine the effect of outdoor conditions depending on orientation, which buildings in different settlement patterns with different H/W values are exposed to.
Table 3. The ratio of depth of shadow to building heights in Istanbul (on January 21)
Since the urban canyon was designed on a two-dimensional section, ignoring cross-roads and with the assumption that buildings were to be placed on the canyon axis with a semi-infinite length (Oke, Citation1988), a settlement pattern based on slab was used to analyse the effect of H/W ratios on outdoor conditions, and a settlement pattern based on pavilion was used to analyse the effect of outdoor conditions, which the buildings are exposed to from four directions. Accordingly, for the three different H/W ratios, a settlement pattern based on the pavilion model was used with buildings with both a square and rectangle floor plan. Urban texture based on a slab model was used for buildings with a rectangle floor plan (Figure ).
2.3. Developing settlement pattern alternatives
When developing settlement pattern alternatives with the identified design parameters, a minimum of nine (3 × 3) detached blocks were accepted within a study area of approximately 30,000 m2. The number of buildings in the settlement pattern alternatives varied depending on the urban geometries. Settlement pattern designs were developed with the assumption that all buildings in a settlement had the same design parameters as the reference residential building. Thirty-six settlement pattern alternatives were developed in the study (Table ).
Table 4. Settlement pattern alternatives developed in the study
2.4. Identifying weather files
Climate data used in the analysis of building energy performance is mainly hourly data based on global climate models and existing outdoor weather conditions. Climate data changes during the lifespan of a building; the Intergovernmental Climate Change Panel (IPCC) has been making comprehensive scientific assessments on anthropogenic climate change since 1988. The goal of these assessments is to present climate change, its effects and the options for adaptation or reduction in a comprehensive, objective and transparent way based on scientific, technical and socioeconomic data. Groups of experts at the IPCC have created global climate models (GCM) and made global warming forecasts based on their observations. Therefore, the evaluation of a building’s energy performance should include not only existing climate conditions, but also systematic descriptions and assumptions about potential climate conditions in order to be consistent and to enable buildings to be adaptable. As described in the IPCC Third Assessment Report (TAR) and Fourth Assessment Report (AR4), 40 scenarios were developed based on several variables, including future potential carbon emission levels along with an increased population, increased energy consumption and economic development, as well as four basic scenario groups: A1, A2, B1 and B2. Scenario group A1 describes alternative ways of technological change in energy systems, classified into three sub-scenarios based on technological developments and changes. In scenario A1F1, fossil fuels are used at a high percentage; in scenario A1T, non-fossil energy sources are used; in scenario A1B, all resources are used equally. In scenario group A2, assumptions that vary depending on non-homogenous local characteristics are defined. Scenario group B1 assumes that the global population will increase until the mid-century before levelling off and then decreasing, causing rapid changes in economic structures. It also emphasizes global solutions in which economic, social and environmental sustainability is maintained using clean and resource-effective technologies. Scenario group B2 considers local solutions first in order to achieve economic, social and environmental sustainability (IPCC, Citation2018).
In this paper, scenario A2 which draws a structure similar to the existing one, emphasizing population increase, local economic development and which are used the most in studies was selected. To generate future weather files according to the scenario A2, the projected years were taken into account within three periods: 2011–2030 (referred as “2030s”), 2046–2065 (referred as “2060s”), 2080–2099 (refered as “2090s”). For this study, the selected representative periods/years were: Base case (BC), using 2010 weather file (as the year 2010 was the hottest year ever recorded in Turkey); near-future (NF) was represented by 2030; intermediate-future (IF) was represented by 2060; far-future (FF) was represented by 2090 (The anomalies of temperature and global radiation of the three periods were used for creating the representative year weather file). Four representative years were used for the simulations carried out for Istanbul, representing a temperate-humid climate region. Weather data required for the scenarios were prepared in an EPW (Energy Plus Weather File) format with the Meteonorm 7.0 program, which is a combination of climate database, spatial interpolation tool and a stochastic weather generator. Meteonorm can calculate typical years with hourly or minute time resolution for any site and can also be used for climate change studies. This tool uses the GCMs under the IPCC AR4 rather than climate data stored in typical weather files, in other words, the results of IPCC AR4 are used as input instead of climate values (Meteonorm Software, Citation2013; Moazami, Nik, Carlucci, & Geving, Citation2019; Remund, Müller, & Reahm, Citation2010). In this regard, when the future weather data in EPW files created by Meteonorm was analysed, the change can be seen only on global radiation and air temperature except wind speed. It can be seen that the forecast changes of global radiation until 2100 with all scenarios are relatively small compared to temperature changes. However, air temperature averaged over the period 2080–2099 are projected to likely exceed 2°C. The characteristics of the weather files (BC and A2 scenarios) are provided in Table .
Table 5. Characteristics of the weather files (BC, NF, IF, FF)
2.5. Evaluating the impacts of climate change on building energy performance in the context of urban geometry
Energy analyses were carried out using the weather files selected to evaluate potential impacts of climate change on the building’s energy performance within different settlements. The DesignBuilder simulation program (DesignBuilder Software, Citation2017), which is the comprehensive interface of EnergyPlus dynamic thermal simulation engine was used in these energy analyses. Annual heating, cooling and total (heating + cooling) energy consumptions of the reference residential building in 36 different settlement pattern alternatives developed for Istanbul were calculated for four different weather files. The results of these calculations are compared and shown in Figure and Table .
Figure 3. Heating (a), cooling (b) and total (heating + cooling) (c) energy consumptions of the reference residential building defined in different settlement patterns
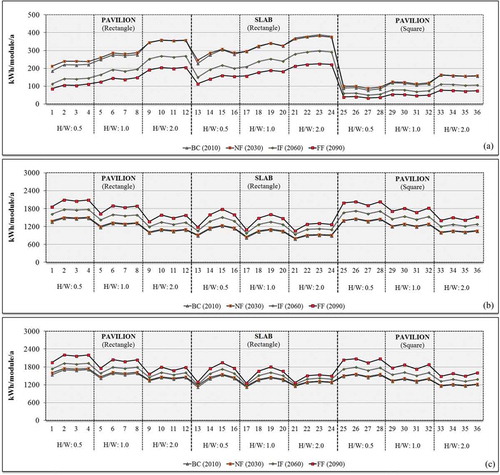
Table 6. Energy analysis results of the reference residential building in different settlement patterns
When the energy analysis results of the reference residential building—defined in the settlement pattern alternatives based on pavilions with a rectangle floor plan—was examined, the lowest heating energy consumption within the BC scenario obtained in alternative 1 with H/W: 0.5 and orientated 0°. The lowest cooling and total energy consumption were obtained in alternative 9 with H/W: 2.0 and oriented 0°. On the other hand, the highest heating energy consumption in the same scenario was found in alternative 10 with H/W: 2.0 and oriented 45°. The highest cooling and total energy consumption were obtained in alternative 4 with H/W: 0.5 and oriented 135°. For the NF, IF and FF scenarios, the lowest heating energy consumption was found in alternative 1; when compared to the BC scenario, it was calculated that heating energy consumption increased by 14% in 2030 and decreased 39% and 53% in 2060 and 2090, respectively. The lowest cooling and total energy consumption were obtained in alternative 9. When compared to the BC scenario, the increment values of 3%, 21% and 39% were calculated for cooling energy consumption concerning to the representative future years, and it was found that total energy consumption increased by 2%, 9% and 17% in 2030, 2060 and 2090, respectively. Under future climate scenarios developed for the A2 scenario, the highest heating energy consumption was found in alternative 10; when compared to the BC scenario, heating energy consumption did not change significantly in 2030 and decreased by 25% and 43% in 2060 and 2090, respectively. The highest cooling and total energy consumption were obtained in alternative 4. When compared to the BC scenario, cooling energy consumption increased by 2%, 20% and 41%, and total energy consumption increased by 3%, 13% and 29% in 2030, 2060 and 2090, respectively.
With regard to the energy analysis results of the reference residential building—defined in the settlement pattern alternatives based on slabs with a rectangle floor plan—the lowest heating energy consumption within the BC scenario was found in alternative 13 with H/W: 0.5 and orientated 0°; the lowest cooling energy consumption was obtained in alternative 21 with H/W: 2.0 and oriented 0°; and the lowest total energy consumption was found in alternative 17 with H/W:1.0 and oriented 0°. On the other hand, the highest heating energy consumption in the same scenario was obtained in alternative 23 with H/W: 2.0 and oriented 90°; and the highest cooling and total energy consumption were found in alternative 15 with H/W: 0.5 and oriented 90°. For the NF, IF and FF scenarios, the lowest heating energy consumption was obtained in alternative 13; when compared to the BC scenario, it was found that heating energy consumption increased by 8% in 2030 and decreased 34% and 50% in 2060 and 2090, respectively. The lowest cooling energy consumption was obtained in alternative 21. When compared to the BC scenario, the cooling energy increased by 4%, 20% and 35% in 2030, 2060 and 2090, respectively. The lowest total energy consumption was found in alternative 17; and the increment on total energy consumption in comparison to the BC scenario was calculated as 3%, 6% and 12% concerning the future scenarios. Under future climate scenarios developed for the A2 scenario, the highest heating energy consumption was found in alternative 23; when compared to the BC scenario, heating energy consumption decreased by 1%, 23% and 42% in 2030, 2060 and 2090, respectively. In terms of both cooling and total energy consumption, the highest values were found in alternative 15. The increment values related to the cooling energy consumption for future scenarios in comparison to the BC scenario were minimum 2%, maximum 46%. With regard to the total energy consumption, when it was compared to the BC scenario, it increased by 2%, 13% and 27% in 2030, 2060 and 2090, respectively.
When heating energy consumption per module for the reference residential building—defined in the settlement pattern alternatives based on pavilions with a square floor plan—was examined, the lowest heating energy consumption within the BC scenario was obtained in alternative 27 (77 kWh/module/a) with H/W:0.5 and oriented 90°. The lowest cooling and total energy consumption within the BC scenario were obtained in alternative 33 with H/W:2.0 and orientated 0°. The highest cooling and total energy consumption in the same scenario were found in alternative 26 with H/W: 0.5 and oriented 45°. For the NF, IF and FF scenarios, the lowest heating energy consumption was obtained in alternative 27; when compared to the BC scenario, it was found that heating energy consumption increased 15% in 2030 and decreased by 35 % and 57 % in 2060 and 2090, respectively. The lowest cooling and total energy consumption were obtained in alternative 33. When compared to the BC scenario, the cooling energy increased by 4%, 21% and 41% in 2030, 2060 and 2090, respectively. In terms of total energy consumption, the increment was calculated as minimum 2%, maximum 29% concerning the future scenarios. Under future climate scenarios developed for the A2 scenario, the highest heating energy consumption was found in alternative 33; when compared to the BC scenario, heating energy consumption did not change significantly in 2030 and decreased by 32% and 53% in 2060 and 2090, respectively. The highest cooling and total energy consumption was obtained in alternative 26. When compared to the BC scenario, cooling energy consumption increased by 1%, 19% and 40% in 2030, 2060 and 2090, respectively, and total energy consumption increased by min 2%, maximum 35% concerning the future scenarios.
3. Conclusion
The results of this study show that the energy performance of buildings is affected by climate change, and the effect of these changes will increase in the coming years. New settlement patterns have been designed for urban climates that will be affected by rapid urbanization and climate change, the effects of which are felt at varying levels in these different settlement patterns. Therefore, it has become increasingly difficult to maintain thermal comfort conditions in changing urban climates, especially due to the increasing trend of urbanization and the effects of climate change. Considering the lifespans of buildings and settlements, it is certain that future-oriented approaches in designing building environments will have significant benefits in the long term for energy-based economies affected by climate change. Turkey, a developing country in one of the most sensitive regions for climate change, must integrate their approaches in ongoing urban renewal processes in order to limit an increase in unsustainable energy, thereby decreasing the burden of energy costs on the economy.
In this regard, with the approach developed in this study it is possible to have a holistic evaluation of the impacts of future weather data on the energy performance of residential buildings in the context of urban geometry. In the parametric analysis of Istanbul, where the number of new settlements is rapidly increasing, settlement pattern alternatives based on pavilion and slab, with five-storey buildings using square and rectangle floor plans with H/W: 0.5, 1.0 and 2.0, were developed. Separate energy performance analyses were carried out using four different weather files in order to determine the potential effects of climate change on the energy performance of the reference residential building in each settlement pattern alternative. The findings of the energy analysis demonstrated that the solar radiation and solar gain of the modules in the reference residential building increased as a result of the high space between buildings with a decreasing H/W ratio; therefore, heating and total energy consumption decreased, and cooling energy consumption increased. When energy analysis results of the reference residential building in settlement pattern alternatives based on a square and a rectangle floor plan were examined, the effect of orientation on the energy consumption per module in the reference residential building with a rectangle floor plan was higher than the effect on the energy consumption per module in the reference residential building with a square floor plan. When the long façades of the reference residential building with a rectangle floor plan were parallel to the north-south direction, in other words for alternatives in which the reference street had an east-west orientation (0º), heating and cooling energy consumption was lower. For the reference residential building with a square floor plan, the orientation had a neglectable level of impact on the energy performance of the building. And additionally, it is possible to say that the effective orientation on the energy performance of the reference residential building with a square floor plan was north-south reference street orientation (90º). In terms of settlement pattern type, the results indicated that the cooling and total energy consumption per module in the slabs were lower than that in the pavilions. Yet the heating energy consumption per module in the settlement pattern based on pavilions was lower than the settlement pattern based on slabs. In terms of future climate scenarios developed for the A2 scenario, the analysis results demonstrated that the temperature increase will be somewhat limited in the near-future (until the end of the 2030s) in Turkey; however, when the results were evaluated for the following period, particularly the intermediate-future and far-future, it was determined that temperatures will rise rapidly. Therefore, annual cooling energy consumption is estimated to increase, whereas heating energy consumption is estimated to decrease. It was concluded that, in the future, cooling energy consumption will account for a higher percentage of total energy consumption. The most important change was found in the energy performance analysis for scenario A2 in the year 2090, which predicted a 60% decrease in heating energy consumption and a 50% increase in cooling energy consumption. Considering the weather files used in this study, it can be surmised that the main reason why there is an expected increase in cooling energy consumption is the increase in air temperatures as opposed to solar gains, which remain constant. Additionally, the findings of this study support the assertion that climate change decreases the length of the heating period and increases the length of the cooling period. These estimates demonstrate the potential surge in the demand for electricity and the maximum load—in other words, the primary energy source in most buildings will be electricity, not natural gas or similar fuels, and there will be a significant upward trend for electricity consumption. Higher CO2 emissions caused by this increase in electricity consumption for cooling needs will contribute to global warming and, in turn, the rise of temperatures will further increase electricity demand and consumption.
Therefore, instead of using active cooling systems to achieve thermal comfort conditions in buildings, passive solutions—which will play an active role in reducing cooling requirements—should be considered. In this respect, the evaluation of the impacts of climate change on the energy performance of buildings within a certain settlement pattern becomes even more important. Buildings must be adapted to provide indoor and outdoor thermal comfort conditions in order to reduce or nullify the negative effects of climate change on their energy performance.
Findings of this study have helped make predictions about the impacts of climate change on the energy performance of the reference residential building, reflecting common mass housing typology in Turkey using different settlement pattern alternatives. Since Turkey is largely dependent on import energy sources and now faces an energy demand above the global average, using future weather data to prevent or minimize the impacts—and performing impact analysis based on these data—plays an important role in reducing the energy and carbon intensity of the Turkish economy. Due to the worrying rise in global temperatures and the result this will have on the energy performance of buildings, the efficiency of the settlement pattern, which is the most important parameter affecting energy performance, will inevitably be deeply altered. However, if climate change is considered a cross-cutting issue, the number of analyses should be much higher to achieve an acceptable consensus. Potential impacts of future weather data on the energy performance of buildings must be determined in order to change the decision-making mechanism for the design of settlement patterns—ones that can be adapted to changing conditions during this period in which urban renewal projects continue at full speed in developing countries such as Turkey. Considering the threatening surge in global temperatures—and the impacts of such an increase on a building’s energy performance—the importance of making correct decisions and creating sustainable environments for national resources will become more imperative.
Additional information
Funding
Notes on contributors
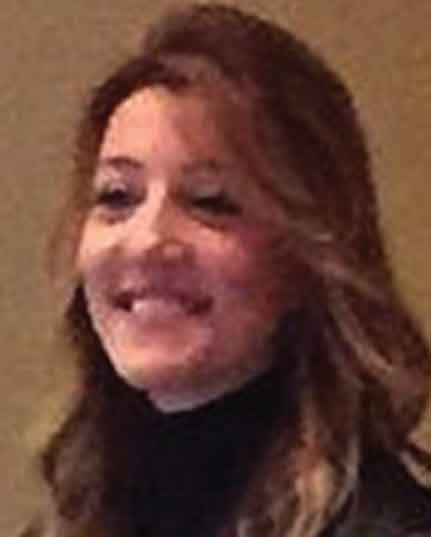
Suzi Dilara Mangan
Suzi Dilara Mangan has been working as a Visiting Researcher at the Department of the Built Environment, Eindhoven University of Technology (TU/e) since January 2019. Her current research focuses on developing a Decision Support Tool that will allow different users to take correct decisions to ensure energy and cost efficiency in both the design of new buildings and settlements, and retrofitting of existing buildings. Headlines of her research areas are; sustainability in architecture, sustainable energy in buildings, energy efficient building and settlement design, climate responsive design and building energy simulation.
References
- Ahmad, K., Khare, M., & Chaudhr, K. K. (2005). Wind tunnel simulation studies on dispersion at urban street canyons and intersections-a review. Journal of Wind Engineering and Industrial Aerodynamics, 93, 697–19. doi:10.1016/j.jweia.2005.04.002
- Ali-Toudert, F. (2009, 29 June–3 July). Energy efficiency of urban buildings: Significance of urban geometry, building construction and climate conditions. The Seventh International Conference on Urban Climate, Yokohama, Japan.
- Ali-Toudert, F., & Mayer, H. (2007). Effects of asymmetry, galleries, overhanging façades and vegetation on thermal comfort in urban street canyons. Solar Energy, 81, 742–754. doi:10.1016/j.solener.2006.10.007
- Alves, C. A., Duarte, D. H. S., & Gonçalves, F. L. T. (2016). Residential buildings’ thermal performance and comfort for the elderly under climate changes context in the city of Sao Paulo. Brazil. Energy and Buildings, 114, 62–71. doi:10.1016/j.enbuild.2015.06.044
- Andreou, E. (2013). Thermal comfort in outdoor spaces and urban canyon microclimate. Renewable Energy, 55, 182–188. doi:10.1016/j.renene.2012.12.040
- Bakker, N., & Steemers, K. (2000). Energy and environment in architecture: A technical design guide. London, UK: E &FN Spon.
- Bourbia, F., & Awbi, H. B. (2004). Building cluster and shading in urban canyon for hot dry climate Part 2: Shading simulations. Renewable Energy, 29, 291–301. doi:10.1016/S0960-1481(03)00171-X
- Bulkeley, H., & Tuts, R. (2013). Understanding urban vulnerability, adaptation and resilience in the context of climate change. Local Environment, 18, 646–662. doi:10.1080/13549839.2013.788479
- Cartalis, C., Synodinou, A., Proedrou, M., Tsangrassoulis, A., & Santamouris, M. (2001). Modifications in energy demand in urban areas as a result of climate changes: An assessment for the southeast Mediterranean region. Energy Conversion and Management, 42, 1647–1656. doi:10.1016/S0196-8904(00)00156-4
- DesignBuilder Software. (2017). DesignBuilder 5.0.3.007 user manual. Stroud, UK: DesignBuilder.
- Dhar, T. K., & Khirfan, L. (2017). A multi-scale and multi-dimensional framework for enhancing the resilience of urban form to climate change. Urban Climate, 19, 72–91. doi:10.1016/j.uclim.2016.12.004
- Ewing, R., & Rong, F. (2008). The impact of urban form on U.S. residential energy use. Housing Policy Debate, 19, 1–30. doi:10.1080/10511482.2008.9521624
- Hachem, C., Athienitis, A., & Fazio, P. (2011). Investigation of solar potential of housing units in different neighborhood designs. Energy and Buildings, 43, 2262–2273. doi:10.1016/j.enbuild.2011.05.008
- Höppe, P. (2002). Different aspects of assessing indoor and outdoor thermal comfort. Energy and Buildings, 34, 66–665. doi:10.1016/S0378-7788(02)00017-8
- IEA. https://www.iea.org/topics/energyefficiency/buildings/
- IPCC. Special report: Global warming of 1.5°C, intergovernmental panel on climate change. Retrieved from https://www.ipcc.ch/sr15/chapter/summary-for-policy-makers/
- IPCC. (2018). Emissions scenarios. Retrieved from http://www.ipcc.ch/ipccreports/sres/emission/index.php?idp=3
- Kapsomenakis, J., Kolokotsa, D., Nikolaou, T., Santamouris, M., & Zerefos, S. C. (2013). Forty years increase of the air ambient temperature in Greece: The impact on buildings. Energy Conversion and Management, 74, 353–365. doi:10.1016/j.enconman.2013.05.005
- Kariminia, S., Ahmada, S., & Saberi, A. (2015). Microclimatic conditions of an urban square: Role of built environment and geometry. Procedia-Social and Behavioral Sciences, 170, 718–727. doi:10.1016/j.sbspro.2015.01.074
- Lam, J. C., Tang, H. L., & Li, D. H. W. (2008). Seasonal variations in residential and commercial sector electricity consumption in Hong Kong. Energy, 33, 513–523. doi:10.1016/j.energy.2007.10.002
- Lam, J. C., Wan, K. K. W., Lam, T. N. T., & Wong, S. L. (2010). An analysis of future building energy use in subtropical Hong Kong. Energy, 35(2010), 1482–1490. doi:10.1016/j.energy.2009.12.005
- Meteonorm Software. (2013). Meteonorm 7.1.0 handbook. Meteonorm.
- Moazami, A., Nik, V. M., Carlucci, S., & Geving, S. (2019). Impacts of future weather data typology on building energy performance-Investigating long-term patterns of climate change and extreme weather conditions. Applied Energy, 238, 696–720. doi:10.1016/j.apenergy.2019.01.085
- Oke, T. R. (1988). Street design and urban canopy layer climate. Energy and Buildings, 11, 103–113. doi:10.1016/0378-7788(88)90026-6
- Ratti, C., Baker, N., & Steemers, K. (2005). Energy consumption and urban texture. Energy and Buildings, 37(2005), 762–776. doi:10.1016/j.enbuild.2004.10.010
- Ratti, C., Raydan, D., & Steemers, K. (2003). Building form and environmental performance: Archetypes, analysis and an arid climate. Energy and Buildings, 35, 49–59. doi:10.1016/S0378-7788(02)00079-8
- Remund, J., Müller, S., & Reahm, B. (2010). The use of Meteonorm weather generator for climate change studies. EMS Annual Meeting Abstracts, 7, 417.
- Santamouris, M. (2016). Cooling the buildings—past, present and future. Energy and Buildings, 128, 617–638. doi:10.1016/j.enbuild.2016.07.034
- Shang, C., Lin, K., & Hou, G. (2013). Simulating the impact of urban morphology on energy demand-A case study of Yuehai, China. 49th ISOCARP Congress, Brisbane, Australia.
- Shibuya, T., & Croxford, B. (2016). The effect of climate change on office building energy consumption in Japan. Energy and Buildings, 117, 149–159. doi:10.1016/j.enbuild.2016.02.023
- Smith, C., & Levermore, G. (2008). Designing urban spaces and buildings to improve sustainability and quality of life in a warmer world. Energy Policy, 36, 4558–4562. doi:10.1016/j.enpol.2008.09.011
- Strømann-Andersen, J., & Sattrup, P. A. (2011). The urban canyon and building energy use: Urban density versus daylight and passive solar gains. Energy and Buildings, 43, 2011–2020. doi:10.1016/j.enbuild.2011.04.007
- Taleghani, M., Tenpierik, M., Van den Dobbelsteen, A., & de Dear, R. (2013). Energy use impact of and thermal comfort in different urban block types in the Netherlands. Energy and Buildings, 67, 166–175. doi:10.1016/j.enbuild.2013.08.024
- Toudert, F. A., & Helmut, M. (2006). Numerical study on the effects of aspect ratio and orientation of an urban street canyon on outdoor thermal comfort in hot and dry climate. Building and Environment, 41, 94–108. doi:10.1016/j.buildenv.2005.01.013
- Turkish Standards Institution (TSI). (2011). Building permit statistics. Ankara, Turkey: Author.
- Turkish Standards Institution (TSI). (2017). Building permit statistics. Ankara, Turkey: Author.
- Turkish Standards Institution (TSI). (2013). TS 825 thermal insulation requirements for buildings. Ankara, Turkey: Turkish Standards Institution.
- UNEP. Buildings and climate change: Status, challenges and opportunities. United Nations Environment Programme. Retrieved from http://wedocs.unep.org/handle/20.500.11822/7783
- UNFCCC. Rapid urbanization increases climate risk for billions of people. Report 03/07/2017, United Nations Framework Convention on Climate Change. Retrieved from https://unfccc.int/news/rapid-urbanizationincreases- climate-risk-for-billions-of-people
- UN-Habitat. Retrieved from http://unhabitat.org/urbanthemes/energy/
- Van Esch, M. M. E., Looman, R. H. J., & de Bruin-hordijk, G. J. (2012). The effects of urban and building design parameters on solar access to the urban canyon and the potential for direct passive solar heating strategies. Energy and Buildings, 47, 189–200. doi:10.1016/j.enbuild.2011.11.042
- Vianna, S. B., da Veiga, J. E., & Abranches, S. (2009). A Sustentabilidade do Brasil. Giambiagi & Barros (orgs.) Brasil Pós-Crise, Agenda Paraa Próxima Década. Rio de janeiro: Ed. Campus, 305–324.
- Wong, N. H., Jusuf, S. K., Syafıi, N. I., Chen, Y., Hajadi, N., Sathyanarayanan, H., & Manickavasagam, Y. V. (2011). Evaluation of the impact of the surrounding urban morphology on building energy consumption. Solar Energy, 85, 57–71. doi:10.1016/j.solener.2010.11.002
- Zhai, Z. J., & Helman, J. M. (2019). Implications of climate changes to building energy and design. Sustainable Cities and Society, 44, 511–519. doi:10.1016/j.scs.2018.10.043