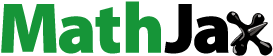
Abstract
Water pollution by dyes from industries is a serious environmental problem. The toxicity of dyes constitutes a great risk to the environment and human health. Due to the simplicity and cost-effectiveness of the remediation process, adsorption has been widely accepted in wastewater treatment. Recent advances in nanotechnology offer considerable advantages in wastewater treatment via the introduction of porous nanomaterials, namely, metal oxide nanoparticles, carbon nanomaterials, and nanocomposites as adsorbents. In this study, a comparison is done between the functionalised and bare nanoparticles in enhancing the adsorption activity of chitosan in adsorbing methylene blue dye. Functionalized CuO nanoparticles were synthesized and characterized by FTIR, Powder X-ray Diffraction (XRD), SEM, and XPS methods. The crystallite size of CuO particles was found to be ~10 nm. The CuO nanoparticle was then functionalized to produce CuO-EDTA and CuO-EDTA-Silane complexes. The development of such functionalized nanoparticles leads to multi-functional properties such as the ability as catalysts in the presence of sunlight for degradation of dyes as well as adsorption of dyes. The BET adsorption study of the functionalized nanoparticles revealed it has a higher surface area than the pure CuO nanoparticles. These bare and functionalised nanoparticles were then separately dispersed with chitosan and adsorption study was done separately. The functionalised nanoparticles with chitosan shows more adsorption activity.
PUBLIC INTEREST STATEMENT
Water pollution by dyes or drug intermediates from industries is a serious environmental problem. The toxicity of dyes constitutes a great risk to the environment and human health. Due to the simplicity and cost-effectiveness of the remediation process, adsorption has been widely accepted in wastewater treatment. In this paper, we report the functionalized CuO nanoparticles for such applications.
1. Introduction
Dyes are the major pollutants in the water bodies near to paint, pigment and textile industries. These dyes accumulate in the water bodies leading to a reduction in the photosynthetic activity of aquatic flora. This also leads to an increased biological oxygen demand (BOD) and chemical oxygen demand (COD) due to which anaerobic degradation of dyes takes place in the water which is potentially harmful to aquatic life (Mishra et al., Citation2018). Copper oxide nanoparticles are widely used in the field of catalysis, superconductors, ceramics as a kind of important inorganic material (Christian, Citation2008; Ibrahim et al., Citation2017; Ren, Citation2009). It can be used as a catalyst and catalyst support, as well as electrode active material. The nano copper oxide shows superior catalytic activity and selectivity than that of the bulk copper oxide powder. The functionalization of nanoparticle leads to high surface functionality, increases the surface area and more importantly helps in controlling the agglomeration of particles over time. This can offer excellent adsorption activity of dyes and pesticides to the nanoparticles (Frost, Citation2017; Guzman, Citation2012).
To date, a large number of functionalized and non-functionalized copper oxide nanoparticles have been synthesized for various applications. Most of the CuO nanoparticles were studied for their antimicrobial activity. Generally, metal nanoparticles such as Pt, Rh, Pd, and Ru are used as catalysts (Aijaz, Citation2012; Kolhatkar, Citation2013; Li, Citation2013; Xu, Citation2018). Due to the high cost of these metal nanoparticles, they are replaced with non-noble metal nanoparticles like Cu, Zn, Co, Ni, Mo (Al-Quaradawi et al., Citation2002; Begletsova, Citation2018; Carpio, Citation2014; Dehaghi, Citation2014; Hughes, Citation2018; John, Citation2015; Magdalena, Citation2018; Mwafy, Citation2019; Usai, Citation2019) or wherever applicable with their oxides (Albert, Citation2018; Becheri, Citation2008; Fang, Citation2019; Li, Citation2018; Moret, Citation2016; Shashikala et al., Citation2013; Vithanage et al., Citation2015; Zhang et al., Citation2013).
Chitosan is a natural cationic, cellulose biopolymer of high molecular weight obtained commercially from deacetylation of chitin by thermochemical reaction (Hansan et al., Citation2003). Chitosan contains high units of two functional groups, hydroxyl (-OH) and amino (-NH2), which are responsible for the reactivity of this polymer as an excellent natural adsorbent and give chitosan its powerful adsorptive capacity (Wan, Citation2012). In recent studies, Chitosan-based metal particles composites have been studied as an alternative adsorbent for water treatment such as metals, metal oxides, etc. (Dehaghi, Citation2014)
In the present study, CuO nanoparticles (CuO NPs) are functionalised with carboxylic acid and silane functional groups, using the reagents EDTA (Ethylenediamine tetraacetic acid) and APTES (Aminopropyl triethoxysilane). The overall study has been carried out in three steps. The first step deals with the synthesis of pure CuO nanoparticles, second step with the synthesis of EDTA-CuO and EDTA-Silane CuO nanoparticles and in the third step; we have investigated the adsorption of dye by the composite of these nanoparticles separately with chitosan, i.e.,, CuO-Chitosan, EDTA-CuO-Chitosan and EDTA Silane- CuO- Chitosan composites.
2. Experimental details
2.1. Materials
Cu(NO3)2. 3H2O (Merck, 0.4 M), Sodium hydroxide (Merck, 2 M), EDTA (Merck, 0.1 M), APTES (Sigma-aldrich, 99%), Chitosan (Sigma-aldrich, 500 ppm), Methylene blue (Sigma-aldrich, 2 ppm), Methyl orange (Sigma-aldrich, 1 ppm), 0.01 M HCl, Glacial acetic acid, double-distilled water.
2.2. Preparation of CuO nanoparticles (NPs)
To a 50 mL copper nitrate trihydrate (0.4 M) was added 1 ml of glacial acetic acid and heated to 100° C with constant stirring. Then, 100 mL of 2 M Sodium hydroxide was added till pH reaches 7. The colour of the solution turned from green to black and the large quantity of black precipitate was formed immediately. The precipitate was kept overnight, filtered and washed 3–4 times with distilled water. Subsequently, the washed precipitates were dried at 80°C for 16 h. Finally, the precursors were calcined at 500°C for 4 h. (Phiwdang, Citation2013) investigated by X-Ray Diffractometry (XRD). The morphology was monitored by scanning electron microscope (SEM). Chemical properties were investigated by Fourier transform infrared spectroscopy.
2.3. Preparation of EDTA functionalized CuO NPs
The EDTA functionalized CuO NPs were synthesized by using a similar procedure used for the synthesis of CuO nanoparticles. Then, it was added with 50 mL of 0.1 M EDTA solution soon after the addition of 2 M sodium hydroxide. Heated the solution until it turns to a black precipitate with constant stirring. The precipitate was kept overnight and filtered and dried.
2.4. Preparation of EDTA-Silane functionalized CuO NPs
For the synthesis of EDTA – Silane functionalized CuO NPs, the procedure adopted is the same as that of bare copper oxide nanoparticles. Then, it was added with a mixture of a solution of 25 mL 0.1 M EDTA and 25 mL amino propyl triethoxy silane after the addition of 2 M sodium hydroxide. Heated the solution until a black precipitate was formed with constant stirring. The precipitate was kept overnight and filtered and dried.
2.5. Characterization
2.5.1. FTIR analysis
FTIR spectra of pure CuO, EDTA-CuO nanoparticles, EDTA-Silane CuO nanoparticles were studied by using Thermo Fischer Nicolet IS5 (USA) in the wavelength range of 4000–400 cm−1.
2.5.2. XRD analysis
The crystalline structure of the CuO and functionalized CuO NPs were analyzed with Rigaku miniflex X-ray diffraction, Japan (Cu Kα radiation, with a wavelength of λ 50.1546 nm) at 15 kV and 50 mA and the spectra of 2θ range from 5º to 80º.
2.5.3. XPS analysis
The surface composition of the pure and functionalised CuO nanoparticles was analyzed with Kratos axis ultra and Kratos axis nova (UK). It is a surface-sensitive analytical technique, probing the 5–10 nm of a sample surface for elemental and chemical-state information.
2.5.4. SEM analysis
The surface morphology of the nanoparticle was determined using Zeiss EVO 18 (Germany) which produces images of a sample by scanning the surface with a focused beam of electrons.
2.5.5. BET adsorption study
To study the adsorption behaviour of the nanoparticles, it is important to know the effective surface area of it (Li, Citation2013). The BET adsorption isotherm is the method used to determine the surface area of the sample. Brunauer–Emmett-Teller (BET) (using Quantachrome Instruments Version 3.0, USA) theory explains the physical adsorption of gas molecules on a solid surface and serves as the basis for an important analysis technique for the measurement of the specific surface area of materials. Nitrogen is the most commonly employed gaseous adsorbate used for surface probing by BET methods. For this reason, standard BET analysis is most often conducted at the boiling temperature of N2 (77 K).
2.5.6. Adsorption study
The Methylene blue dye was used without further purification. 500 ppm (mg/dm3) chitosan solution was prepared by dissolving chitosan in 0.01 M HCl (500 mL solution). 2 g/dm3 of methylene blue was prepared using distilled water to prevent interference from other substances. 0.1 g of both EDTA, EDTA-Silane functionalized and bare CuO NPs were weighed and transferred to three different reagent bottles. 50 mL of chitosan solution was added to each of the bottles. Then, the nanoparticles were dispersed in the chitosan solution for equal distribution of particles using a sonicator for 5 minutes. Then, to that solution was added 6 mL of Methylene blue solution and diluted the mixture to 100 mL by adding distilled water. The solutions were mixed for 10 minutes using an electrically operated agitator at 100 rpm speed. The pH of each solution was brought to three by adding HCl. Then, the solutions were mixed again for 10 minutes at 100 rpm speed and the pH of the solution was corrected to 10 for each sample by adding NaOH. Solutions were mixed for 10 minutes at 100 rpm speed and allowed to settle the solidified chitosan. After a predetermined time, the solutions were filtered and measured the absorbance using UV-Visible spectrophotometer (Aguado, Citation2009; Chen, Citation2011; Dehaghi, Citation2014; Haldorai, Citation2014; Huang, Citation2009; Kumashiro, Citation1999; Lin, Citation1999; Phan, Citation2006; Sang, Citation2008; Shen, Citation2012; Tofighy, Citation2011; Volanti, Citation2008).
3. Results and discussion
3.1. Characterization
Characterization is to be done to find the structure, size, and shape of the synthesized nanoparticles. To characterize the synthesized bare and functionalized CuO nanoparticles, the following techniques were used.
3.1.1. XRD analysis
Figure shows the XRD spectra of pure CuO nanoparticle. The XRD pattern revealed the orientation and crystalline nature of copper oxide nanoparticles. Crystalline peaks corresponding to (110), (002), (111), (202), (020), (202), (113), (311), (220), and (311) diffraction planes at 2θ angles of 32.2, 35.2, 38.4, 48.4, 53.2, 57.9, 61.2, 65.9, 67.8 and 71.9°, respectively, were observed. No another diffraction peaks of other phases are detected, investigating the phase purity of CuO-NPs (Syame et al., Citation2017). The average crystallite size of the synthesized copper oxide nanoparticles was calculated using Debye-Scherrer equation
where D is the particle size, λ = 1.54060 for Cu Kα1, K is the crystallite shape factor (a good approximate is K = 0.9), d is the FWHM (Full Width Half Maxima) obtained from the spectra using the Origin 9 software which was observed to be 0.90059, θ is the angle from the origin (in radians) corresponding to FWHM which was observed to be 0.3357 radians. On substituting these values in the equation, the calculated particle size (D) was observed to be 93.4276 Å or 9.3428 nm. Hence, the crystallite size of particles was found to be around 10 nm.
3.1.2. Scanning electron microscopy
Figure ) shows the SEM images of pure CuO NPs. The SEM images of pure CuO nanoparticle shows that it has a flower like shape and it is crystalline (Volanti, Citation2008). Figure ) and Figure ) shows the SEM images of EDTA-functionalized and EDTA-Silane functionalized CuO nanoparticles. The SEM images of EDTA functionalized CuO nanoparticles shows that they are amorphous and the EDTA-Silane functionalized CuO nanoparticles are crystalline in nature. The EDTA-functionalised CuO is present as clusters or agglomerates and EDTA-Silane functionalized CuO is rod-shaped crystals.
3.1.3. FTIR analysis
FTIR study was done to confirm whether the nanoparticle has got functionalized successfully or not. The sharp peak at 610 cm−1 has been assigned due to the Cu -O stretching peak (Rohilla, Citation2020). Figure shows the comparison of FTIR spectra of pure CuO, EDTA functionalized CuO and EDTA-Silane functionalized CuO NPs.
Figure 3. FTIR spectra showing the comparison between the peaks of pure and functionalized CuO nanoparticles.
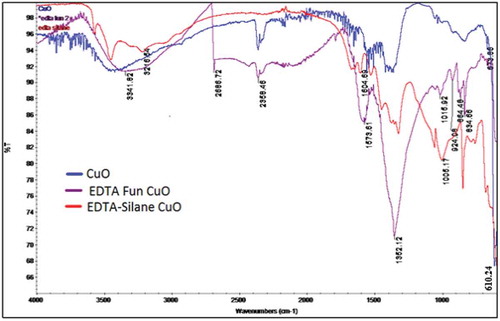
The IR spectrum of CuO-EDTA functionalized nanoparticle shows a sharp peak at 1352.12 cm−1 which corresponds to the C-N stretching in EDTA and a peak near 600 cm−1 representing the Cu-O stretching vibration. Hence, it is proved that the nanoparticle has got functionalized with EDTA successfully. The IR spectrum of EDTA-Silane functionalised CuO nanoparticle show a broad peak at 1005.17 cm−1 for Si-O stretching, a peak at 1322 cm−1 for O-C stretching and a sharp peak near 700 cm−1 for Cu-O stretching vibrations.
3.1.4. XPS analysis
Figure -) shows the XPS spectra of EDTA functionalized CuO NPs. When electromagnetic radiation of high frequency i.e., X-ray made to fall on the atom, the electron from the innermost shell gets knocked out. This electron is called the photoelectron. This photoelectron is detected at the detector to know the binding energy and hence to find the atom present on the surface of the sample. Figure ) shows the characteristic binding energy of 284 eV which is specific for the carbon atom. Figure ) shows the characteristic binding energy of 932 eV which is specific for the Cu atom. Figure ) shows the characteristic binding energy of nearly 950 eV which is specific for the oxygen atom. There are some low-intensity peaks in the spectra which are produced due to the collision of photo-ejected electrons with other electrons of different layers.
Figure 4. (a) XPS spectra of EDTA functionalized CuO nanoparticles showing the presence of C by ejection of electron from C-1 s orbital. (b) XPS spectra of EDTA functionalized CuO nanoparticles showing the presence of Cu by the ejection of electron from the Cu-2p orbital. (c) XPS spectra of EDTA functionalized CuO nanoparticles showing the presence of Cu by the ejection of electron from the O-1 s orbital.
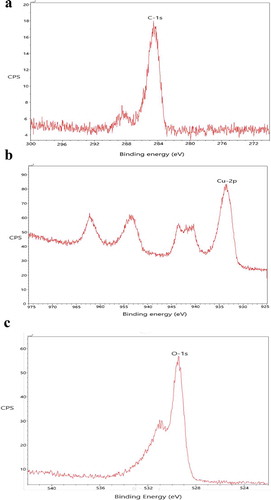
3.1.5. BET adsorption study
From the literature, it was found that the average surface area of CuO nanoparticle is 15.6931 m2/g and they are non-porous (Ren, Citation2009). Figure ,) shows the surface area and pore diameter of the nanoparticles, respectively. Here, the BET adsorption study of EDTA functionalized CuO shows that the mean surface area is 22.749 m2/g. The particles were also found to be porous and the pore diameter was found to be 1.347 nm and pore volume 0.038 cc/g. Hence, on functionalization, the surface area has increased and the nanoparticle has also turned into porous which is more suitable for the adsorption activity.
3.2. Applications
3.2.1. Study of adsorption activity
The following results were obtained from the effect of pure and functionalized CuO nanoparticles on the adsorption activity of chitosan. Figure shows the formation of the chitosan complex with the functionalised copper oxide nanoparticles. This study was done using the methylene blue dye as the adsorbent as it is the main pollutant in the industrial and textile wastewater. The analysis of the effect of nanoparticle on the adsorption activity of chitosan was done using UV-Visible spectrophotometry (Li, Citation2013).
Figure shows the UV-Visible spectra of the dye solution treated with pure and functionalized CuO nanoparticle after the adsorption process. UV-Visible spectrum has characteristic peaks that shows a decrease in absorbance when pure and functionalized CuO nanoparticles were used for adsorbing the dye. This decrease in absorbance refers to decrease in colour of the dye i.e., decrease in the concentration of the dye in the solution since absorbance is directly proportional to the concentration as explained by Beer-Lamberts law (Vithanage et al., Citation2015). There is a decrease in the concentration of dye solution as the added nanoparticle contains more functional groups. This infers more dye has been adsorbed by the chitosan due to the effect of functionalised CuO nanoparticles. Figure shows the decrease in the intensity of the dye solution when treated with functionalized and pure CuO nanoparticles.
The percentage of removal of dye using chitosan incorporated with different CuO nanoparticle were calculated by the following equation,
- Initial absorbance
—Final absorbance
Using this expression, it was found that when bare CuO nanoparticle was added to chitosan, the percentage removal of dye was 24%, when EDTA functionalized CuO nanoparticle was added to chitosan, the percentage removal of dye was 35% and when EDTA-Silane functionalized CuO nanoparticle was added to chitosan, the percentage removal of dye was 54%. Table shows the data of percentage removal of dye. From this data, it was found that the functionalised CuO nanoparticles can increase the adsorption capacity of chitosan and as the functional groups on CuO increased, there was an increase in adsorption of dye. This is due to the combination of the nanoparticles with the chitosan takes place on dispersion which results in the addition functional groups on to chitosan from the nanoparticle. These more electronegative groups present in the functional group will easily adsorb the aromatic groups, i.e. the methylene blue dye, which results in the adsorption of such molecules on to the adsorbent, i.e. the chitosan. The nanoparticles also play an important role in increasing the surface area of the adsorbent.
Table 1. Percentage adsorption by nanoparticles
4. Conclusion
The nanoparticles were synthesized by the co-precipitation method using copper nitrate as the precursor. The bare nanoparticle was characterized by XRD and SEM to determine the size, which proves that the synthesized nanoparticles were in nano range. The functionalization of CuO NPs was effectively done by introducing EDTA and EDTA-Silane complex to the precursor. The functionalized CuO was characterized by FT-IR and SEM techniques. These studies prove that the CuO NPs were successfully functionalized and it was in nano-size range. The XPS analysis of EDTA functionalized CuO proves that the surface has atoms such as Cu, C and O. The BET adsorption study of the EDTA functionalized CuO nanoparticle has an enhanced surface area and also shows the presence of pores which the bare nanoparticle doesn’t have. The increased surface area of the nanoparticles imparts various abilities to the nanoparticle i.e. it can enhance the adsorption activity of chitosan, and also increase photocatalytic activity. The adsorption activity of the nanoparticle shows that, bare CuO nanoparticle combined chitosan was used, the percentage removal of dye was 24%, when EDTA functionalized CuO nanoparticle combined chitosan was used the percentage removal was 35% and when EDTA-Silane functionalized CuO nanoparticle combined chitosan was used the percentage removal of dye was 54%. The functionalized CuO nanoparticle enhances the adsorption activity of chitosan by introducing functional groups to the chitosan and also the nanoparticle can impart swelling behavior to the chitosan. Though precipitation method doesn’t offer particles of uniform size, the method is cost-effective and suitable for bulk applications like industrial waste water treatments where cost considerations are high.
Additional information
Funding
Notes on contributors
M. P. Geetha
Ms Pratheeksha P is an alumna of the Post Graduate Department of Chemistry, St Agnes Centre for Post Graduate studies and Research, Mangalore, India and currently working as an Assistant Professor in the same Institute. Geetha Mable Pinto, is currently serving as Associate Professor in the Post Graduate Department of Chemistry, St Agnes centre for Post Graduate studies and Research, Mangalore, India. She has about 20 years of research & teaching experience. Her research interests include corrosion and nanochemistry.
Dr. Bhat works as an Associate Professor, Department of Chemistry, Manipal Institute of Technology, Manipal, India. He has obtained six sigma green belt certification from GE-India. His research interests include Synthetic chemistry, and Physics and Chemistry of Materials. He recently co-edited a book titled “Fiber-reinforced-nanocomposites-fundamentals-and-applications” published by Elsevier (2020).
References
- Aguado, J. (2009). Aqueous heavy metals removal by adsorption on amine-functionalized mesoporous silica. Journal of Hazardous Materials, 163(1), 213–12. https://doi.org/10.1016/j.jhazmat.2008.06.080
- Aijaz, A. (2012). Immobilizing highly catalytically active pt nanoparticles inside the pores of metal–organic framework: A double solvents approach. Journal of American Chemical Society, 134(34), 13926–13929. https://doi.org/10.1021/ja3043905
- Ajay, V., Kanny, K., Thomas, S. (2018). Methods for synthesis of nanoparticles and fabrication of nanocomposites. Synthesis of Inorganic Nanomaterials, 16, 121–139. https://doi.org/10.1016/b978-0-08-101975-7.00005-1
- Albert, E. L. (2018). Synthesis and characterization of graphene oxide functionalized with magnetic nanoparticle via simple emulsion method. Results in Physics, 11, 944–950. https://doi.org/10.1016/j.rinp.2018.10.054
- Al-Quaradawi, S., et al. (2002). Photocatalytic degradation of methyl orange as a model compound. Journal of Public Policy and Administration, 148(1-3), 161–168. https://doi.org/10.1016/S1010-6030(02)00086-2
- Becheri, A. (2008). Synthesis and characterization of zinc oxide nanoparticles: Application to textiles as UV-absorbers. Journal of Nanoparticle Research, 10(4), 679–689. https://doi.org/10.1007/s11051-007-9318-3
- Begletsova, N. (2018). Chemical synthesis of copper nanoparticles in aqueous solutions in the presence of anionic surfactant sodium dodecyl sulfate. Colloids and Surfaces A: Physicochemical and Engineering Aspects, 552, 75–80. https://doi.org/10.1016/j.colsurfa.2018.05.023
- Carpio, I. E. M. (2014). Graphene oxide functionalized with ethylenediamine triacetic acid for heavy metal adsorption and anti-microbial applications. Carbon, 77, 289–301. https://doi.org/10.1016/j.carbon.2014.05.032
- Chen, Z. (2011). Adsorption behavior of epirubicin hydrochloride on carboxylated carbon nanotubes. International Journal of Pharmaceutics, 405(1–2), 153–161. https://doi.org/10.1016/j.ijpharm.2010.11.034
- Christian, P. (2008). Nanoparticles: Structure, properties, preparation and behaviour in environmental media. ECOTOXICOLOGY, 17(5), 326–343. https://doi.org/10.1007/s10646-008-0213-1
- Dehaghi, S. M. (2014). Removal of permethrin pesticide from water by chitosan–zinc oxide nanoparticles composite as an adsorbent. Journal of Saudi Chemical Society, 18(4), 348–355. https://doi.org/10.1016/j.jscs.2014.01.004
- Fang, X. (2019). Synthesis of cerium oxide nanoparticles loaded on chitosan for enhanced auto-catalytic regenerative ability and biocompatibility for the spinal cord injury repair. Journal of Photochemistry and Photobiology B: Biology, 191, 83–87. https://doi.org/10.1016/j.jphotobiol.2018.11.016
- Frost, M. S. (2017). The response of citrate functionalised gold and silver nanoparticles to the addition of heavy metal ions. Colloids and Surfaces A: Physicochemical and Engineering Aspects, 518, 15–24. https://doi.org/10.1016/j.colsurfa.2016.12.036
- Guzman, M. (2012). Synthesis and antimicrobial activity of silver nanoparticles against gram-positive and gram-negative bacteria. Nanomedicine, 8, 37–45. https://doi.org/10.1016/j.nano.2011.05.007
- Haldorai, Y. (2014). An efficient removal of methyl orange dye from aqueous solution by adsorption onto chitosan/MgO composite: A novel reusable adsorbent. Applied Surface Science, 292, 447–453. https://doi.org/10.1016/j.apsusc.2013.11.158
- Hansan, S., et al. (2003). Adsorption of chromium(VI) on chitosan coated perlite. Separation Science and Technology, 38(15), 3775–3793. https://doi.org/10.1081/SS-120024229
- Huang, S.-H. (2009). Rapid removal of heavy metal cations and anions from aqueous solutions by an amino-functionalized magnetic nano-adsorbent. Journal of Hazardous Materials, 163(1), 174–179. https://doi.org/10.1016/j.jhazmat.2008.06.075
- Hughes, D. L. (2018). Metal removal from soil leachates using DTPA-functionalised maghemite nanoparticles, a potential soil washing technology. Chemosphere, 209, 480–488. https://doi.org/10.1016/j.chemosphere.2018.06.121
- Ibrahim, K., et al. (2017). Nanoparticles: Properties, applications and toxicities. Arabian Journal of Chemistry, 12(7), 145–151. https://doi.org/10.1016/j.arabjc.2017.05.011
- John, S. (2015). Enhancement of corrosion protection of mild steel by chitosan/ZnO nanoparticle composite membranes. Progress in Organic Coatings, 84, 28–34. https://doi.org/10.1016/j.porgcoat.2015.02.005
- Kolhatkar, A. (2013). Tuning the Magnetic Properties of Nanoparticles. International Journal of Molecular Sciences, 14(8), 15977–16009. https://doi.org/10.3390/ijms140815977
- Kumashiro, R. (1999). New analysis of oxidation state and coordination environment of copper ion-exchanged in ZSM-5 zeolite. The Journal of Physical Chemistry B, 103(1), 89–96. https://doi.org/10.1021/jp981935t
- LI, H. (2013). Adsorption behavior and adsorption mechanism of Cu(II) ions on amino-functionalized magnetic nanoparticles. Transactions of Nonferrous Metals Society of China, 23(9), 2657–2665. https://doi.org/10.1016/S1003-6326(13)62782-X
- Li, R. (2018). Tannase immobilisation by amino-functionalised magnetic Fe3O4-chitosan nanoparticles and its application in tea infusion. International Journal of Biological Macromolecules, 114, 1134–1143. https://doi.org/10.1016/j.ijbiomac.2018.03.077
- Lin, S. W. (1999). An innovative method for removing Hg2+ and Pb2+ in ppm concentrations from aqueous media. Chemosphere, 39(11), 1809–1817. https://doi.org/10.1016/S0045-6535(99)00074-0
- Magdalena, A. G. (2018). EDTA-functionalized Fe3O4 nanoparticles. Journal of Physics and Chemistry of Solids, 113, 5–10. https://doi.org/10.1016/j.jpcs.2017.10.002
- Mishra, J., Jha, M., Kaur, N., & Ganguli, A. K. (2018). Room temperature synthesis of urea based imidazole functionalised ZnO nanorods and their photocatalytic application. Materials Research Bulletin, 102, 311–318. https://doi.org/10.1016/j.materresbull.2018.02.045
- Moret, S. (2016). Functionalised silicon oxide nanoparticles for fingermark detection. Forensic Science International, 259, 10–18. https://doi.org/10.1016/j.forsciint.2015.11.015
- Mwafy, A. (2019). Multi walled carbon nanotube decorated cadmium oxide nanoparticles via pulsed laser ablation in liquid media. Optics and Laser Technology, 111, 249–254. https://doi.org/10.1016/j.optlastec.2018.09.055
- Phan, N. T. S. (2006). Highly accessible catalytic sites on recyclable organosilane-functionalised magnetic nanoparticles: An alternative to functionalised porous silica catalysts. Journal of Molecular Catalysis A-chemical, 253, 123–131. https://doi.org/10.1016/j.molcata.2006.03.019
- Phiwdang, K. (2013). Synthesis of CuO nanoparticles by precipitation method using different precursors. Energy Procedia, 34, 740–745. https://doi.org/10.1016/j.egypro.2013.06.808
- Ren, G. (2009). Characterisation of copper oxide nanoparticles for antimicrobial applications. International Journal of Antimicrobial Agents, 33(6), 587–590. https://doi.org/10.1016/j.ijantimicag.2008.12.004
- Rohilla, D. (2020). Dopamine functionalized CuO nanoparticles: A high valued “turn on” colorimetric biosensor for detecting cysteine in human serum and urine samples. Materials Science and Engineering: C, 110, 110724. https://doi.org/10.1016/j.msec.2020.110724
- Sang, Y. M. (2008). Filtration by a novel nanofiber membrane and alumina adsorption to remove copper(II) from groundwater. Journal of Hazardous Materials, 153(1–2), 860–866. https://doi.org/10.1016/j.jhazmat.2007.09.035
- Shashikala, M., et al. (2013). Studies on the removal of methylene blue dye from water using chitosan. International Journal of Development Research, 3, 40–44.
- Shen, H. Y. (2012). A new insight on the adsorption mechanism of amino-functionalized nano-Fe3O4 magnetic polymers in Cu(II), Cr(VI) co-existing water system. Chemical Engineering Journal, 183, 180–191. https://doi.org/10.1016/j.cej.2011.12.055
- Syame, S. M., Mohamed, W. S., Mahmoud, R. K., & Omara, S. T. (2017). Synthesis of copper-chitosan nanocomposites and its application in treatment of local pathogenic isolates bacteria. Oriental Journal of Chemistry, 33(6), 2959–2969. https://doi.org/10.13005/ojc/330632
- Tofighy, M. A. (2011). Adsorption of divalent heavy metal ions from water using carbon nanotube sheets. Journal of Hazardous Materials, 185(1), 140–147. https://doi.org/10.1016/j.jhazmat.2010.09.008
- Usai, V. (2019). Synthesis and characterisation of cobalt oxide nanoparticles decorated graphene oxide and its electrocatalytic behaviour. Polyhedron, 157, 192–199. https://doi.org/10.1016/j.poly.2018.10.002
- Vithanage, A. H., Madhushanka, A. K. D. N., Ariyadasa, T., & Gunavardena, S. H. P. (2015). Textile dye removal in wastewater using chitosan. Annual Sessions of IESL (pp. 283–289). The Institution of Engineers, Srilanka.
- Volanti, D. P. (2008). Synthesis and characterization of CuO flower-nanostructure processing by a domestic hydrothermal microwave. Journal of Alloys and Compounds, 459(1–2), 537–542. https://doi.org/10.1016/j.jallcom.2007.05.023
- Wan, W. S. (2012). Synthesis and characterisation of magnetic β-cyclodextrin-chitosan nanoparticles as nano-adsorbents for removal of methylene blue. International Journal of Biological Macromolecules, 50, 444–450. https://doi.org/10.1016/j.ijbiomac.2011.12.016
- Xu, M. (2018). Highly dispersed CuCo nanoparticles supported on reduced graphene oxide as high-activity catalysts for hydrogen evolution from ammonia borane hydrolysis. Journal of Nanoparticle Research, 20(12), 329. https://doi.org/10.1007/s11051-018-4429-6
- Zhang, Y. X., et al. (2013). Controlled synthesis of hierarchical CuO nanostructures for electrochemical capacitor electrodes. International Journal of Electrochemical Science, 8, 8645–8661. http://www.electrochemsci.org/papers/vol8/80608645.pdf