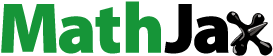
Abstract
In the present investigation, coumarin derivative 4-((4-amino-5-thio-1,2,4-triazol-3-yl)methyl)coumarin (ATTC) have synthesized as novel ecofriendly corrosion inhibitor and evaluated the corrosive inhibitive properties on mild steel in 1 M hydrochloric acid solution through weight loss, electrochemical impedance spectroscopy (EIS) and Potentiodynamic (PD) polarization techniques. The corrosion analysis results show that ATTC inhibitive corrosion by mechanism of adsorption and display inhibition performance around 96% at the concentration of 0.5 mM. From the morphology analysis by means of scanning electron microscopy (SEM) techniques of the protective film on mild steel surface, it was confirmed that adsorption of ATTC molecules appears on the mild steel surface through chemi-sorption, that additional retard the corrosion rate. The stability of ATTC molecule was investigated in the gaseous state and it has thio and mercapto forms. Based on of density functions theory (DFT) results, thio possess higher stability state compared with mercapto. From the thermodynamic parameters, it was found that the conversion of thio form to mercapto form is spontaneous and endothermic.
PUBLIC INTEREST STATEMENT
A novel ecofriendly compound, containing two biologically active moieties N-amino-2-mercapto-1,3,4-triazole and coumarin, namely “4-((4-amino-5-thio-1,2,4-triazol-3-yl)methyl)coumarin” (ATTC) was synthesized and fully characterized by spectroscopically techniques. Its inhibitive performance towards the corrosion inhibition of mild steel in 1 M HCl using an experimental approach complemented with theoretical investigation. ATTC acted as a good corrosion inhibitor for mild steel corrosion in 1 M HCl showing an efficiency of 96% at 0.5 mM and 30 °C. The adsorption model follows Langmuir adsorption isotherm. Inhibition efficiency increases with inhibitor concentration increase and decreases with temperature increase.
Conflicts of Interest
The authors declare no conflict of interest.
1. Introduction
Mild steel is significant alloy that has huge utilization in manufacture because of its superior mechanical characteristics and low expenses. Generally mild steel utilized in different industries as material of construction for reactor, heat exchanger and boiler and storage in addition to pipelines of oil and gas transport (Singh & Quraishi, Citation2012; Tao et al., Citation2009). Moreover, mild steel utilized in chemical manufactures in handling corrosive solutions. Hydrochloric acid is generally applied in industrial approaches such as acid pickling, cleaning and acidification. Hydrochloric acid has less problems and low expensive from other halogenic and mineral acids (Singh et al., Citation1995). The major feature of hydrochloric acid is its capability to form metal chlorides, that is quite soluble in aqueous environment, compared to nitrates, phosphate and sulphates. The excellent solubility of chlorides causes the minimum polarizing impact and does not impedance the corrosion rate (Emregul & Hayval, Citation2006; Hudson & Warning, Citation1970). The utilization of inhibitors is considered the extreme efficient technique foralloy protection versus corrosive solutions. The investigation of corrosion on MS in corrosive environment is of academic and industrial attention and has received a significant amount of concern of considerable researchers. In general corrosion inhibitors are natural or organic compounds having hetero-atoms such as nitrogen, oxygen, sulfur, and phosphorous in addition to double or triple bonds and aromatic ring (Bentiss et al., Citation2001; Hosseini et al., Citation2003; Singh, Citation2012). The organic molecules containing -C = N-, electron donator, and polar groups are reported to conduct as efficient inhibitors of MS in corrosive environment (Fekry & Riham, Citation2010; Hegazy et al., Citation2011; Nada et al., Citation2011). Though considerable coumarins were investigated as corrosion inhibitors of MS, most of these synthesized coumarins failed to demonstrate significant inhibition performance at higher temperatures (Badr, Citation2009; Quraishi et al., Citation2001; Shanbhag et al., Citation2008). The aim of the present work is to study the influence of 2-(3,4,5-trimethoxybenzylidene) hydrzinecarbothiamide (TMBHC), as a corrosion inhibitor for mild steel in 1 M hydrochloric acid using Tafel polarization and EIS methods. Further, the study also focuses on the inhibition mechanism based on the adsorption isotherms, activation and thermodynamic parameters obtained. Recently, interest in the development and use of low-cost and environmentally friendly compounds as corrosion inhibitors have increased (Al-Amiery et al., Citation2013; Al-Majedy et al., Citation2019; Jawad et al., Citation2019; Junaedi et al., Citation2012; Salman, Jawad et al., Citation2019). The novelty of present investigation lies in the synthesis, characterization, and applied of novel 4-aminothiosemicarbazide derivative as a corrosion inhibitor for the protection of mild steel in a 1 M HCl environment. The interest of this 4-aminothiosemicarbazide is its excellent inhibitive performance (91%), low inhibitive concentrations, long active time and potent bonds between the surface of tested coupons and inhibitor molecules. Following up of the investigations for efficient corrosion inhibitors for mild steel in acidic solution (Al-Amiery et al., Citation2013; A. A. Al-Amiery, Kadhum, Alobaidy et al., Citation2014; A. A. Al-Amiery, Kadhum, Kadihum et al., Citation2014; Ahmed et al., Citation2018; Al-Amiery et al., Citation2015, Citation2016; Al-Azawi et al., Citation2018; S. Al-Baghdadi et al., Citation2018; Al-Obaidy et al., Citation2015; ASTM G77-98, Citation1998; S. B. Al-Baghdadi et al., Citation2016; Habeeb et al., Citation2018; Jamil et al., Citation2018, Citation2018; Kadhim et al., Citation2017; Kadhum et al., Citation2014; Mohamad et al., Citation2014; Obayes et al., Citation2017, Citation2014; Rubaye et al., Citation2015; T. Salman et al., Citation2019; Yousif et al., Citation2015), the present investigation is to study the inhibition action of the green corrosion inhibitor derivative of coumarin which is 4-((4-amino-1,2,4-triazole-5 (4 H)-thione-3-yl) methyl) coumarin (ATTC), on the corrosion of mild steel in hydrochloric acid. The chemical formula of the novel ecofriendly corrosion inhibitor was elucidating according to spectroscopic techniques (FTIR, NMR) and CHN analysis. The effect of ATTC inhibition was investigated by weight lost calculations, electrochemical impedance spectroscopy (EIS), s as well as by surface analysis using scanning electron microscopy (SEM) with a different concentration of ATTC as corrosion inhibitor. The potential mechanism for ATTC absorption has been explored on the mild steel surface.
2. Experimental
2.1. Materials and chemical analyses
The solvents and chemicals were purchased from Sigma-Aldrich, KL, Malaysia and were utilized as received without further purification. Carbon Hydrogen Nitrogen (CHN) analysis were done through 5500-Carlo Erba analyzer. Sectrophototechniques were done through Nuclear magnetic resonance (AVANCE III 600 MHz spectrometer) and Fourier-transform infrared spectroscopy (Thermo Scientific Model Nicolate 6700).
2.2. Synthesis of corrosion inhibitors
4-Coumarinacetic acid (6.125 gm, 30.0 mmol) and 4-aminothiosemicarbazide (3.180 gm, 30.0 mmol) in POCl3 (15.0 gm) have beeen stirred at (0–5 °C). Reflux for and add dry pyridine (5.0 ml). Poured onto ice and the precipitated was filtered and recrystallized from ethanol. Brown powder 57.0 % was yielded and the melting point was 258.0 °C. The ATTC is displayed in Scheme . The ATTC was fully characterized using FTIR, NMR and CHN analysis. FT-IR: amino groups (3287.5 cm−1, 3172.7 cm−1 and 3161.3 cm−1), methylene group (2911.6 cm−1), carbonyl group (1713.8 cm−1). 1H-NMR (DMSOd6): 1H, for benzene ring, d, 7.31 ppm, d, 7.39 ppm, d, 7.67 ppm, d, 7.51 ppm; for C = C-H, 1H, s, 5.93 ppm; for CH2; for NH2 group, 2 H, s, 5.16 ppm. 13C-NMR in DMSO-d6: for benzene ring (115.3 ppm, 118.9 ppm, 121.9 ppm, 127.3 ppm, 132.8 ppm, 153.6 ppm); for carbonyl group (159.4 ppm); for vinyl group (109.5 ppm and 150 ppm); for methylene group 39.4 ppm and for N = C-N 176.2 ppm. CHN, analytical calculation (found) for the ATTC: C, 52.54(52.31), H, 3.67(3.29), N, 20.43(20.17).
2.3. Electrode and electrolytes
Mild steel specimens obtained from the Metal Samples Company were used as the working electrodes throughout this study. The chemical compositions of tested coupons were of iron, 99.210; Carbon 0.210; Silicon 0.380; Phosphorus 0.090; Sulphur 0.050; Manganese 0.050; and Aluminium 0.010. 4.5 cm2 was the active surface area tested coupons. The first experimental step was to polish the coupon surface then washed with distilled water, acetone and dry ether (Ahmed et al., Citation2018; ASTM G77-98, Citation1998; Jamil et al., Citation2018). The measurements were conducted in aerated, non-stirred 1.0 M hydrochloric acid solutions at 303, 313, 323 and 333 K at the corrosion inhibitor concentrations: 0 mM, 0.01 mM, 0.2 mM, 0.3 mM, 0.4 mM and 0.5 mM. The solutions were freshly prepared using distilled water. Each measurement was repeated three times, and only the average values were reported to verify the reproducibility of the experiments.
2.4. Weight loss method
Weight loss technique was done according to the reference (ASTM G77-98, Citation1998). The results have been used to calculate the corrosion rate (CR mmpy) and Inhibition efficiency (IE%) in addition to surface coverage (θ) as in Equationequations 11
1 –Equation3
3
3 .
where W, A, d and t are the weight loss, area, density and immersion time of coupon respectively. A similar procedure was applied for various temperature for 5 hours.
2.5. EIS electrochemical experiments
Electrochemical experiments were carried out using the traditional three-electrode system by Reference 600 (Gamry Instrument Potentiostat/Galvanostat/ZRA). A graphite electrode (counter), a reference (saturated calomel electrode (SCE)) and mild steel electrode (working) with an exposed area of 1.0 cm2 was used. The ethanol (anhydrous) and acetone were used to clean the polished working electrode and then immersed in the solution for 30 min to stabilization of the steady-state potential and the measurements have been done at the frequency range of 100 KHz to 0.1 Hz with using the AC signals of the 5 mV peak-to-peak an amplitude of 5 mV at open circuit potential (OCP).
2.6. Surface analysis
A scanning electron microscope test was conducted at the UKM Electron Microscopy Unit. The scanning electron microscope was used to investigate the coupon surface in absence and presence of the corrosion inhibitor in the corrosive environment.
Scanning electron microscopy (SEM, TM1000 Hitachi Tabletop Microscope, was used to examine the mild steel samples that had been immersed in HCl, both with and without the corrosion inhibitor.
3. Results and discussion
3.1. Chemistry of ATTC
The reaction sequences for the synthesis of ATTC starting from 4-coumarinacetic acid and 4-aminothiosemicarbazide are outlined in Scheme . ATTC was synthesized by the reaction of 4-coumarinacetic acid and 4-aminothiosemicarbazide in a presence of POCl3.
3.2. Gravimetric analysis
3.2.1. Effect of ATTC concentration
Figure , demonstrates the corrosion rates of the tested coupons after exposure to the hydrochloric acid environment. Mild steel, for the exposure time of corrosive solution in absence of ATTC molecules has higher corrosion rate than in presence of ATTC molecules. An unexpected reduce in corrosion rate at 0.5 mM and 10 h was noted. Likewise, CR was reduced for all tested ATTC concentrations but at the highest concentration 0.5 mM, the mild steel surface is entirely protected by the ATTC molecules forming a layer on the surface of mild steel that resulted in best inhibitive performance in corrosive environment. Furthermore, 5 h and 10 h of immersion time of tested coupons at 0.5 mM as ATTC concentration have approximately the same CR although the CR displed more significant at 10 h, exposure time and 0.5 mM of inhibitor concentration.
Figure , demonstrates the inhibition efficiency (IE) of the mild steel in hydrochloric acid environment HCl solution in absence and presence of ATTC molecules as corrosion inhibitor. There is a significant increase in the IE of tested inhibitor after the first 5.0 h. Formation of thin film, probably formed because of the coordination bonds between mild steel surface and ATTC molecules from efficient dissolution to inefficient state improve inhibition efficiency. This demonstrates that the ATTC molecules decrease the dissolution and enough to protect the surface of the mild steel from the corrosive environment. The effects of exposure time is not important due to presence of ATTC molecules as a protected layer between the mild steel and hydrochloric acid solution (Jamil et al., Citation2018).
In general, the CR and IE of ATTC molecules depend on the ability of adsorption on the MS. The highest inhibition efficiency of 96.5% have been achieved at the concentration of 0.5 mM attributed to the availability of heterogeneous atoms in the chemical structure of ATTC molecules that participates as active functional groups and electrostatic bonds between ATTC with surface of MS. Nitrogen, sulphur and oxygen atoms interact with the mild steel surface through adsorption and that increase the inhibition efficiency. The active centres in the ATTC molecule that are amine, thionyl, lactone and carbonyl groups interact with the mild steel surface via electron donation to the empty d-orbital of iron thereby enabling the adsorption of the ATTC molecule to the surface of mild steel.
3.2.2. Temperature effect
The inhibition efficiency for mild steel in 1 M hydrochloric acid environment at 303, 313, 323 and 333 K in the absence and presence of ATTC is shown in Figure . The presence of ATTC indicated that inhibition efficiency of mild steel increases with the increase of ATTC concentration to 96.36% at 303 K. As the temperature increases, the inhibition efficiency decreases. At 303, 313, 323 and 333 K maximum inhibition efficiencies of 96. 36%, 87.47%, 78.56%, 71.2% and 36.73% were obtained in 1 M hydrochloric acid medium containing 0.5, 0.4, 0.3, 0.2 and 0.1 mM ATTC, respectively. The results demonstrate that the addition of ATTC decreases the dissolution of mild steel in hydrochloric acid solution. This impedance to dissolution probably because of the formation of the Fe–ATTC complex layer.
3.3. Adsorption isotherm analysis
For investigated the adsorption isotherm followed the relation between the ATTC concentration Cinh and Cinh/θ at the investigated various temperature, is demonstrated in Figure . Straight line with slope and correlation coefficient were obtained referring to that adsorption isotherm obeyed Langmuir adsorption isotherm (as in Equationequation 4)4
4 at all the tested temperature. This refers to formation of mono adsorbed layer of the ATTC molecules mild steel surface.
were is the adsorption constant
3.4. Thermodynamic and kinetic parameters
The activation energy (Ea) was calculated from Arrhenius plots for mild steel corrosion rate demonstrated in Figure according to Equationequation 55
5 .
where R is a constant of molar gas, T is absolute temperature and a is Arrhenius pre-exponential constant.
It is clear from Table that, activation energy of the inhibited environment in this investigation increases by increasing the concentration of ATTC referring point adsorption of the ATTC molecules at the mild steel surface (Gao & Liang, Citation2007). The activation energy value for the highest studied ATTC concentration was (90.2 kJ·mol−1) means that the adsorption is chemical adsorption. On the other hand, the lowest ATTC studied concentration was (55.9 kJ·mol−1) and this value below 80 kJ/mol, required for chemical adsorption, indicating that the adsorption is physical adsorption. It is obvious from EquationEquation 55
5 , that CR is determined by both activation energy and Arrhenius constant. It is obvious from Table that the activation energy value increased on increasing of ATTC concentration. Corrosion rate decrease with increasing ATTC concentration. The transition state equation (Equationequation 6
6
6 ), was derived from Arrhenius equation:
Table 1. Kinetic parameters for MS in HCl in the presence and absence of ATTC
where h is the Planck’s constant and N is the Avogadro’s number.
3.5. Electrochemical impedance spectroscopy measurements
Figure illustrates the characteristics of the corrosion inhibitor at various concentrations of ATTC in a 1 M hydrochloric acid environment at 303 K, as examined using electrochemical impedance spectroscopy (EIS). The equivalent circuit model is shown in Figure by the impedance data synthesis, containing solution resistance (Rs), polarization resistance (Rp) and fixed phase element (CPE), and is presented in Equationequation (7)7
7 :
where the CPE is the steady phase element to substitute a double layer capacitance Cdl to acquire a additional, accurate fit of the methodological data set. n corresponds to the phase shift, that is concerning with the inhomogeneities of the double layer. For n = 0, CPE illustrates an impedance, for n = 1, a capacitance, and for n = −1 an inductance. The dimension of Yo is sn Ω-1
While that of a capacitance (C) is Ω-1 or F. The “double layer capacitance” values (Cdl) are measured from Equationequation8(8)
(8) :
where ω” is the angular frequency corresponding to the maximum imaginary part of the impedance spectrum. The impedance parameters are demonstrated in Table and the inhibitive efficiencies (h) are measured via Equationequation 9(9)
(9) :
Table 2. The EIS parameters for MS in corrosive environment in absence and presence of varaious concentrations of ATTC at 303 K
where Rp and are the resistance (Ω cm2) in presence and absence of ATTC, respectively. From Figure , it is obvious that the Nyquist Figure demonstrates a depressing capacitive loop, but at higher concentration of ATTC, the capacitive loop radius will increase, that means that the charge transfer between the environment and MS is dramatically hindered through the addition of ATTC (Al-Amiery et al., Citation2020; Salman, Al-Amiery et al., Citation2019). It is obvious from Table that the increase in the concentration of ATTC,
increases, that imply that the ATTC molecules have clear inhibition impact after adsorption on the surface of MS. when the increase of ATTC concentration the electric double layer capacitance value decreased, that is due to the ATTC molecules replacing the water molecules adsorbed on the surface of MS.
The results of EIS were obtained, the fitting of the circuit was done through using the parabolic circuit model. The CPE circle was selected as an equivalent model for matching performance. In Figure , the solution resistance was represented by Rs, where the charge transfer resistance and the constant phase element were represented by Rct and CPE, respectively.
Nyquist plot, Bode plot data (EIS) were analyzed and specified as a corrosion mechanism that carried out on the mild steel surface. The data of EIS are generally explained in expression of equivalent electrical circuits which are utilized to describe the electrical features of electrochemical interfaces. Generally, the EIS obtained for mild steel in 1 M hydrochloric acid solution consists of one time/constant in Bode-phase representation, that agreed to the charge-transfer process. The plots mild steel coupon Bode in hydrochloric acid solution without and with ATTC molecules is displayed in Figure .
3.6. Polarization
Potentiodynamic (PD) polarization plots for mild steel coupons in corrosive environment in absence and presence various concentrations of ATTC are demonstrated in Figure . In general, a PD polarization investigation gives significant proof regarding the kinetic participatory in the reaction occurring at cathode/anode areas and which the kind inhibitive action is acceptable by corrosive inhibitor on the basis of corrosive possibility. Recently, in published papers the inhibitors impedance the anodic and cathodic reactions occurring on the surface of mild steel, that concludes in reducing of the corrosion current icorr. The diminish in icorr proposed the corrosive inhibitive phenomenon (Saxena et al., Citation2018; Srivastava et al., Citation2017). Table shows the Tafel parameter Ecorr (corrosion potential), icorr (current density), βa (anodic Tafel constant) and βc (cathodic Tafel constant). From these results, it is obviously clear that the increase of ATTC shifts the corrosion potency at the high negative direction, compared to that in absence of ATTC molecules, indicating a mixed-type inhibitor (Kosari et al., Citation2014; Umoren et al., Citation2014; Yadav & Quraishi, Citation2012). The anodic Tafel constant and cathodic values varied in a comparable style on adding ATTC concentrations, support the inhibition action of mixed type. The increase of ATTC into corrosive environment proceeds a diminution in the value of icorr (42.7 μA/cm2 at 0.5 mM). The decrease of icorr with adding of ATTC compares absence of ATTC, i.e., in acidic solution (661 μA/cm2) for mild steel, obviously specified impedance in the anodic and cathodic reactions, resulting in corrosion retardation. The inhibitive efficiencies are determined according to Equationequation 10(10)
(10) .
Table 3. Potentiodynamic parameters for mild steel coupon without and with ATTC in 1 M hydrochloric acid solution
where is the current density in absence ATTC and
is the corrosion current density in presence ATTC.
3.7. Morphological studies
The mild steel surface was investigated with SEM in absence of ATTC molecules in corrosive environment and in presence of ATTC at the concentration of 0.5 mM for 5 h as immersion time. Figure ), demonstrates the picture of surface of MS in the absence of ATTC molecules. It is clear that the surface of mild steel was corroded. On the other hand, Figure ), demonstrates the picture of surface of MS in presence of ATTC molecules and it was smooth and clear.
3.8. Thermodynamic studies on ATTC tautomers
ATTC and AMTC are formed as demonstrated in Figure . The stability of these isomers from the density functions theory calculations indicated that the ATTC isomer is more stable than AMTC (Table ).
Table 4. Calculated parameters for ATTC and AMTC
This is proved by the total energy value which calculated from each of them, that the total energy of ATTC isomer is grater than AMTC by 5.5222 Kcal/mol. Thermodynamical results demonstrated that reaction (1) is spontaneous due to variation entropy that has positive value (∆S = +) so the ATTC molecule is more stable than AMTC molecule. The variation enthalpy with negative (∆H = -) suggests that (1) is exothermic reaction. The stability of ATTC isomer is as shown in Figure . The band gap values of ATTC and AMTC, are 3.558 eV and 2.942 eV, respectively; this signals that thin molecule in gas phase is insulators for the electrical conductivity.
Figure shows the HOMO and the LUMO electronic distributions for ATTC and AMTC. Other observations account for absorption maximum wavelength (λmax) of both isomers to be 345.5500 nm and 421.4300 nm, respectively. The ATTC and AMTC have no centre of symmetry at all symmetry element hence they are classified as the identity.
3.9. Inhibition mechanism
All the results of the used techniques (weight loss, absorption and morphology) in this study indicate that the new corrosion inhibitor ATTC has played a key role in inhibiting corrosion of mild steel in the acidic environment. It is possible to propose a schematic mechanism of inhibition, the corrosion of the mild steel in the acidic environment by relying the study of the chemical structure and the electronic structure with the results of this study as shown in Figure . Since the ATTC molecules have many functional groups and heteroatoms in addition to electronic pairs as well as pi bonds and aromatic ring. The iron atoms have an unoccupied d-orbital, so the iron atoms have the ability to adsorbthe ATTC molecules, unassociated electrons and thus form strong bonds (coordination bonds) with the mild steel surface. The electrons of the ATTC molecules may accumulate in the atomic orbitals of the mild steel surface and may lead to electron repulsion on the surface of the mild steel and thus reverse transfer occurs. Electrons move from the atoms of the mild steel to the molecular orbitals of the new anti-corrosion molecules known as retrodonation. In this study, the corrosion of iron in an acidic environment produces a surface charged with positive charges, attracting negatively charged ions.
4. Conclusions
The synthesized ecofriendly coumarin ATTC showed good inhibitive performance of 91 at 0.5 mM. ATTC having a triazole substituent demonstrated an excellent inhibitive performance. Electrochemical impedance spectroscopy (EIS) techniques displayed that an increase in the ATTC concentration increases Rp on the mild steel surface and decreases Cdl. Weight loss measurements suggest that the studied ATTC acted as mixed-type inhibitor. The absorption of ATTC obeys Langmuir isotherm. Gibb’s free energy, enthalpy and entropy of adsorption indicate that the adsorption process is spontaneous and endothermic and the ATTC molecules adsorbed on the mild steel surface through chemisorption. EIS, Weight loss and SEM techniques corroborated the formed inhibitor film on the mild steel surface. SEM studies confirmed the presence of a thin film of inhibitor on the MS surface, which is formed ATTC molecules by electrons sharing between d-orbital of mild steel surface and unpaired of electrons of ATTC molecules. Density Function Theory (DFT) showed that the isomer ATTC is more stable AMTC.
Acknowledgements
This study was supported by the University of Technology, Baghdad, Iraq.
Additional information
Funding
Notes on contributors
Ahmed A. Al-Amiery
Ahmed Al-Amiery (SCOPUS h-index= 31), is a Professor at University of Technology, Iraq. He has over 190 scientific papers and projects either presented or published and 21 patents. He is an international expert in many areas of applied chemistry (Synthesis of Organic Molecules and applied these molecules as corrosion inhibitors, antioxidants, scavengers, antimicrobial and anti-cancer). Dr. Al-Amiery is the Editor for many journals. He is Visitor professor at Universiti Kebangsaan Malaysia. Al-Amiery has Post-doctoral from Department of Chemical and Process Engineering Universiti Kebangsaan Malaysia. Dr. Al-Amiery has been awarded the Medal of scientific excellence (2014), and also Hold Science Day Awards from the Ministry of Higher Education and Scientific Research for four consecutive years (2010, 2011, 2019). Al-Amiery selected for the Who’s Who for International Executives 2015. Al-Amiery selected for the one of the best mentalities of the world for 2016 by the University of Cambridge. Al-Amiery is a TWAS- Young Affiliates.
References
- Ahmed, M., Al-Amiery, A., Al-Majedy, Y., Kadhum, A., Mohamad, A., & Gaaz, T. (2018). Synthesis and characterization of a novel organic corrosion inhibitor for mild steel in 1 M hydrochloric acid. Results in Physics, 8(3), 728–17. https://doi.org/10.1016/j.rinp.2017.12.039
- Al-Amiery, A., Kadhum, A., Mohamad, A., Musa, A., & Li, C. (2013). Electrochemical study on newly synthesized chlorocurcumin as an inhibitor for mild steel corrosion in hy-drochloric acid. Materials (Basel), 6(12), 5466–5477. https://doi.org/10.3390/ma6125466
- Al-Amiery, A., Shaker, L., Kadhum, A., & Takriff, M. (2020). Synthesis, characterization and gravimetric studies of novel triazole-based compound. International Journal of Low-Carbon Technologies, 15(2), 164–170. https://doi.org/10.1093/ijlct/ctz067
- Al-Amiery, A. A., Al-Majedy, Y. K., Kadhum, A. A. H., & Mohamad, A. B. (2015). New coumarin derivative as an eco-friendly inhibitor of corrosion of mild steel in acid medium. Molecules, 20(1), 366–383. https://doi.org/10.3390/molecules20010366
- Al-Amiery, A. A., Kadhum, A. A. H., Alobaidy, A. H. M., Mohamad, A. B., & Hoon, P. S. (2014). Novel corrosion inhibitor for mild steel in HCl. Materials (Basel), 7(2), 662–672. https://doi.org/10.3390/ma7020662
- Al-Amiery, A. A., Kadhum, A. A. H., Kadihum, A., Mohamad, A. B., How, C. K., & Junaedi, S. (2014). Inhibition of mild steel corrosion in sulfuric acid solution by new schiff base. Materials (Basel), 7(2), 787–804. https://doi.org/10.3390/ma7020787
- Al-Amiery, A. A., Kadhum, A. A. H., Mohamad, A. B., & Junaedi, S. (2013). A novel hydrazinecarbothioamide as a potential corrosion inhibitor for mild steel in HCl. Materials (Basel), 6(4), 1420–1431. https://doi.org/10.3390/ma6041420
- Al-Amiery, A. A., Kassim, F. A. B., Kadhum, A. A. H., & Mohamad, A. B. (2016). Synthesis and characterization of a novel eco-friendly corrosion inhibition for mild steel in 1 M hydrochloric acid. Scientific Reports, 6(1), 1–13. https://doi.org/10.1038/srep19890
- Al-Azawi, K. F., Mohammed, I. M., Al-Baghdadi, S. B., Salman, T. A., Issa, H. A., Al-Amiery, A. A., Gaaz, T. S., & Kadhum, A. A. H. (2018). Experimental and quantum chemical simulations on the corrosion inhibition of mild steel by 3-((5-(3,5-dinitrophenyl)-1,3,4-thiadiazol-2-yl)imino)indolin-2-one. Results in Physics, 9(6), 278–283. https://doi.org/10.1016/j.rinp.2018.02.055
- Al-Baghdadi, S., Hashim, F. G., Salam, A. Q., Abed, T. K., Gaaz, T. S., Al-Amiery, A. A., Kadhum, A. A. H., Reda, K. S., & Ahmed, W. K. (2018). Synthesis and corrosion inhibition application of NATN on mild steel surface in acidic media complemented with DFT studies. Results in Physics, 8(3), 1178–1184. https://doi.org/10.1016/j.rinp.2018.02.007
- Al-Baghdadi, S. B., Noori, F. T. M., Ahmed, W. K., & Al-Amiery, A. A. (2016). Thiadiazole as a potential corrosion inhibitor for mild steel in 1 M HCl. Journal of Advanced Electrochemistry, 2(3), 67–69.
- Al-Majedy, Y. K., Ibraheem, H. H., Falih, M. S., & Al-Amiery, A. A. (2019). New coumain derivatives as corrosion inhibitor. IOP Conference Series: Materials Science and Engineering, 579(1–10), 012051. https://doi.org/10.1088/1757-899X/579/1/012051
- Al-Obaidy, A., Kadhum, A., Al-Baghdadi, S., Al-Amiery, A., Kadhum, A., & Yousif, E. (2015). Eco-friendly corrosion inhibitor: Experimental studies on the corrosion inhibition performance of creatinine for mild steel in HCl complemented with quantum chemical calculations. International Journal of Electrochemical Science, 10(3), 3961–12972.
- ASTM G77-98. (1998). Standard test method for ranking resistance of materials to sliding wear using block-on-ring wear test. West Conshohocken, USA : ASTM International.
- Badr, G. E. (2009). The role of some thiosemicarbazide derivatives as corrosion inhibitors for C-steel in acid medium. Corrosion Science, 51(11), 2529–2536. https://doi.org/10.1016/j.corsci.2009.06.017
- Bentiss, F., Traisnel, M., & Lagrenee, M. (2001). Influence of 2,5-bis (4-imethylaminophenyl)-1,3,4-thiadiazole on corrosion inhibition of mild steel in acidic media. Journal of Applied Electrochemistry, 31(1), 41–48. https://doi.org/10.1023/A:1004141309795
- Emregul, K. C., & Hayval, M. (2006). Studies on the effect of a newly synthesized Schiff base compound from phenazone and vanillin on the corrosion of steel in 2 M HCl. Corrosion Science, 48(4), 797–812. https://doi.org/10.1016/j.corsci.2005.03.001
- Fekry, A. M., & Riham, R. M. (2010). Acetyl thiourea chitosan as an eco-friendly inhibitor for mild steel in sulphuric acid medium. Electrochimica Acta, 55(6), 1933–1939. https://doi.org/10.1016/j.electacta.2009.11.011
- Gao, G., & Liang, C. (2007). Electrochemical and DFT studies of -amino-alcohols as corrosion inhibitors for brass. Electrochimica Acta, 52(13), 4554–4559. https://doi.org/10.1016/j.electacta.2006.12.058. [CrossRef] [Google Scholar]
- Habeeb, H., Luaibi, H., Dakhil, R., Kadhum, A., Al-Amiery, A., & Gaaz, T. (2018). Development of new corrosion inhibitor tested on mild steel supported by electrochemical study. Results in Physics, 8(3), 1260–1267. https://doi.org/10.1016/j.rinp.2018.02.015
- Hegazy, M. A., Ahmed, H. M., & El-Tabei, A. S. (2011). Investigation of the inhibitive effect of p-substituted 4-(N,N, N-dimethyldodecylammonium bromide)benzylidene-benzene-2-yl-amine on corrosion of carbon steel pipelines in acidic medium. Corrosion Science, 53(2), 671–678. https://doi.org/10.1016/j.corsci.2010.10.004
- Hosseini, M., Mertens, S. F. L., Ghorbani, M., & ArshadiAsymmetrical, M. R. (2003). Schiff bases as inhibitors of mild steel corrosion in sulphuric acid media. Materials Chemistry and Physics, 78(3), 800–808. https://doi.org/10.1016/S0254-0584(02)00390-5
- Hudson, R. M., & Warning, C. J. (1970). Influence of halide mixtures with organic compounds on dissolution and hydrogen absorption by low C-steel in H2SO4. Corrosion Science, 10(3), 121–134. DOI:10.1016/S0010-938X(70)80009-9
- Jamil, D. M., Al-Okbi, A. K., Hanon, M. M., Rida, K. S., Alkaim, A. F., Al-Amiery, A. A., Kadhim, A., & Kadhum, A. A. H., (2018). Carbethoxythiazole corrosion inhibitor: As an experimentally model and DFT theory. Journal of Engineering and Applied Sciences, 13(11), 3952–3959. DOI:10.36478/jeasci.2018.3952.3959
- Jamil, D. M., Al-Okbi, A. K., Al-Baghdadi, S. B., Al-Amiery, A. A., Kadhim, A., Gaaz, T. S., Kadhum, A. A. H., & Mohamad, A. B. (2018). Experimental and theoretical studies of Schiff bases as corrosion inhibitors. Chemistry Central Journal, 12(1), 1–7. https://doi.org/10.1186/s13065-018-0376-7
- Jawad, Q. A., Zinad, D. S., Salim, R. D., Al-Amiery, A. A., Gaaz, T., Takriff, M. S., & Kadhum, A. H. (2019). Synthesis, characterization, and corrosion inhibition potential of novel thiosemicarbazone on mild steel in sulfuric acid environment. Coatings, 9(11), 729. https://doi.org/10.3390/coatings9110729
- Junaedi, S., Kadhum, A., Al-Amiery, A., Mohamad, A., & Takriff, M. (2012). Synthesis and characterization of novel corrosion inhibitor derived from oleic acid: 2-Amino5-Oleyl-1,3,4-Thiadiazol (AOT). International Journal of Electrochemical Science, 7(4), 3543–3554. www.electrochemsci.org/papers/vol7/7043543.pdf
- Kadhim, A., Al-Okbi, A., Jamil, D. M., Qussay, A., & Al-Amiery, A. A. (2017). Experimental and theoretical studies of benzoxazines corrosion inhibitors. Results in Physics, 7(10), 4013–4019. https://doi.org/10.1016/j.rinp.2017.10.027
- Kadhum, A. A. H., Mohamad, A. B., Hammed, L. A., Al-Amiery, A. A., San, N. H., & Musa, A. Y. (2014). Inhibition of mild steel corrosion in hydrochloric acid solution by new coumarin. Materials (Basel), 7(6), 4335–4348. https://doi.org/10.3390/ma7064335
- Kosari, A., Moayed, M. H., Davoodi, A., Parvizi, R., Momeni, M., Eshghi, H., & Moradi, H. (2014). Electrochemical and quantum chemical assessment of two organic compounds from pyridine derivatives as corrosion inhibitors for mild steel in hcl solution under stagnant condition and hydrodynamic flow. Corrosion Science, 78(10), 138–150. https://doi.org/10.1016/j.corsci.2013.09.009. [CrossRef] [Google Scholar]
- Mohamad, A., Kadhum, A., Al-Amiery, A., Ying, L., & Musa, A. (2014). Synergistic of a coumarin derivative with potassium iodide on the corrosion inhibition of aluminum alloy in 1.0M H2SO4. Metals and Materials International, 20(3), 459–467. https://doi.org/10.1007/s12540-014-3008-3
- Nada, F. A., Fekry, A. M., & Hamdi, M. H. (2011). Corrosion inhibition, hydrogen evolution and antibacterial properties of newly synthesized organic inhibitors on 316L stainless steel alloy in acid medium. International Journal of Hydrogen Energy, 36(11), 6462–6471. https://doi.org/10.1016/j.ijhydene.2011.02.134
- Obayes, R., Al-Amiery, A., Alwan, G., Abdullah, T., Kadhum, A., & Mohamad, A. (2017). Sulphonamides as corrosion inhibitor: Experimental and DFT studies. Journal of Molecular Structure, (6),1138, 27–34. https://doi.org/10.1016/j.molstruc.2017.02.100
- Obayes, R., Alwan, G. H., Alobaidy, A. H. M., Al-Amiery, A. A., Kadhum, A. A. H., & Mohamad, A. B. (2014). Quantum chemical assessment of benzimidazole derivatives as corrosion Inhibitors. Chemistry Central Journal, 8(1), 1–8. https://doi.org/10.1186/1752-153X-8-21
- Quraishi, M. A., Sardar, R., & Jamal, D. (2001). Corrosion inhibition of mild steel in hydrochloric acid by some aromatic hydrazides. Materials Chemistry and Physics, 71(3), 309–313. DOI: 10.1016/S0254-0584(01)00295-4
- Rubaye, A., Abdulwahid, A., Al-Baghdadi, S., Al-Amiery, A., Kadhum, A., & Mohamad, A. (2015). Cheery sticks plant extract as a green corrosion inhibitor complemented with LC-EIS/MS spectroscopy. International Journal of Electrochemical Science, 10(10), 8200–8209. http://www.electrochemsci.org/papers/vol10/101008200.pdf
- Salman, T., Zinad, D., Jaber, S., Al-Ghezi, M., Mahal, A., Takrif, M., & Al-Amiery, A. (2019). Effect of 1,3,4-thiadiazole scafold on the corrosion inhibition of mild steel in acidic medium: an experimental and computational study. Journal of Bio- and Tribo-Corrosion, 5(4), 1–11. https://doi.org/10.1007/s40735-019-0243-7
- Salman, T. A., Al-Amiery, A. A., Shaker, L. M., Kadhum, A. A. H., & Takriff, M. S. (2019). A study on the inhibition of mild steel corrosion in hydrochloric acid environment by 4-methyl-2-(pyridin-3-yl)thiazole-5-carbohydrazide. International Journal of Corrosion and Scale Inhibition, 8(4), 1035–1059. https://doi.org/10.17675/2305-6894-2019-8-4-14
- Salman, T. A., Jawad, Q. A., Hussain, M. A. M., Al-Amiery, A. A., Shaker, L. M., Kadhum, A. A. H., & Takriff, M. S. (2019). Novel ecofriendly corrosion inhibition of mild steel in strong acid environment: Adsorption studies and thermal effects. International Journal of Corrosion and Scale Inhibition, 8(4), 1123–1137. https://doi.org/10.17675/2305-6894-2019-8-4-19
- Saxena, A., Prasad, D., Haldhar, R., Singh, G., & Kumar, A. (2018). Use of Sida cordifolia extract as green corrosion inhibitor for mild steel in 0.5 M H2SO. Journal of Environmental Chemical Engineering, 6(1), 694–700. https://doi.org/10.1016/j.jece.2017.12.064. [CrossRef] [Google Scholar]
- Shanbhag, A. V., Venkatesha, T. V., Prabhu, R., Kalkhambkar, R. G., & Kulkarni, G. M. (2008). Corrosion inhibition of mild steel in acidic medium using hydrazide derivatives. Journal of Applied Electrochemistry, 38(3), 279. https://doi.org/10.1007/s10800-007-9436-8
- Singh, A. K. (2012). Inhibition of mild steel corrosion in hydrochloric acid solution by 3-(4-((Z). Indolin-3-ylideneamino)phenylimino)indolin-2-one. Industrial & Engineering Chemistry Research, 51(8), 3215–3223. https://doi.org/10.1021/ie2020476
- Singh, A. K., & Quraishi, M. A. (2012). Study of some bidentate schiff bases of isatin as corrosion inhibitors for mild steel in hydrochloric acid solution . International Journal of Electrochemical Science, 7(4), 3222–3241. http://www.electrochemsci.org/papers/vol7/7043222.pdf
- Singh, D. D. N., Singh, T. B., & Gaur, B. (1995). GaurThe role of metal cations in improving the inhibitive performance of hexamine on the corrosion of steel in hydrochloric acid solution. Corrosion Science, 37(6), 1005–1019. https://doi.org/10.1016/0010-938X(95)00010-H
- Srivastava, V., Haque, J., Verma, C., Singh, P., Lgaz, H., Salghi, R., & Quraishi, M. A. (2017). Amino acid based imidazolium zwitterions as novel and green corrosion inhibitors for mild steel: Experimental, DFT and MD studies. Journal of Molecular Liquids, 244(10), 340–352. https://doi.org/10.1016/j.molliq.2017.08.049. [CrossRef] [Google Scholar]
- Tao, Z., Zhang, S., Li, W., & Hou, B. (2009). The role of metal cations in improving the inhibitive performance of hexamine on the corrosion of steel in hydrochloric acid solution. Corrosion Science, 51(11), 2588–2595. https://doi.org/10.1016/j.corsci.2009.06.042
- Umoren, S. A., Obot, I. B., Israel, A. U., Asuquo, P. O., Solomon, M. M., Eduok, U. M., & Udoh, A. P. (2014). Inhibition of mild steel corrosion in acidic medium using coconut coir dust extracted from water and methanol as solvents. Journal of Industrial and Engineering Chemistry, 20(9), 3612–3622. https://doi.org/10.1016/j.jiec.2013.12.056. [CrossRef] [Google Scholar]
- Yadav, D. K., & Quraishi, M. A. (2012). Application of some condensed uracils as corrosion inhibitors for mild steel: Gravimetric, electrochemical, surface morphological, UV–visible, and theoretical investigations. Industrial & Engineering Chemistry Research, 51 (46), 14966–14979. https://doi.org/10.1021/ie301840y. [CrossRef] [Google Scholar]
- Yousif, E., Win, Y., Al-Hamadani, A., Al-Amiery, A., Kadhum, A., & Mohamad, A. (2015). Furosemi as an environmental-friendly inhibitor of corrosion of zinc metal in acid medium experimental and theoretical studies. International Journal of Electrochemical Science, 10(2), 1708–1718. http://www.electrochemsci.org/papers/vol10/100201708.pdf
- Zarrouk, A., Hammouti, B., Lakhlifi, T., Traisnel, M., Vezin, H., & Bentiss, F. (2015). New 1H-pyrrole-2,5-dione derivatives as efficient organic inhibitors of carbon steel corrosion in hydrochloric acid medium: Electrochemical, XPS and DFT studies. Corrosion Science, 90(1), 572–584. https://doi.org/10.1016/j.corsci.2014.10.052. [CrossRef] [Google Scholar]