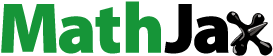
Abstract
The brittle response of concrete largely influences concrete deformation under axial load in the post-peak descending branch of the stress-strain curve. In this study, unplasticized polyvinyl chloride (uPVC) plastic tube used to confine concrete cylinders and improve the post-peak performance of specimens subjected to axial compression loads. Three experimental parameters, tube diameter, tube thickness, and H/D ratio, used to examine the mechanical behavior of eighteen uPVC tube confined short concrete columns. Test results show that when the outer diameter to thickness ratio (D/t) changed from 22 to 12.9, local buckling of the polymeric tube decreased, and the strain at peak stress was increased. So far, there is no model for predicting the strain of plastic tube confined concrete. The present test data was combined with more than 160 test data collected from the published literature to compile an experimental database. The number of test data for the strain of uPVC tube confined concrete reported in the literature is limited and less than those for strength. However, the assembled database was abundant enough to develop an ultimate condition model for the maximum axial strain accurately, based on the database. The developed model’s accuracy was verified by comparing model predictions with twenty existing FRP-confined concrete models using three statistical indices. The distinguishing feature of the strain model includes several parameters on the concrete confined by the uPVC tube.
PUBLIC INTEREST STATEMENT
The uPVC tube is a universal thermoplastic polymer commercially available for sewage and several other construction industry applications. The tube can be used for encasing concrete with durability, environmental and economic advantages. The tube exhibits acceptable behavior under applied load with a ductile response and sufficient deformations before the ultimate failure. The encasing tube is of particular interest for structural or architectural columns in several civil applications such as low rise industrial and residential buildings. Other potential uses of plastic tubes are as a pier and pile in bridge infrastructure applications found in hostile environments—the thin-walled tubular functions as a barrier to prevent the ingress of harmful materials into the encased concrete. The plastic could be strengthened externally using fiber reinforced polymers as strips or full wraps for carrying additional loads.
3. Introduction
One of the most tempting uses of commercial uPVC tubes is for structural applications for concrete encasement in compression members. The plastic tube has favorable intrinsic characteristics and offers permanent mold to the fresh concrete and considerable confinement to the hardened concrete. Several materials have reported having the potential for use as confining devices for concrete in bridge piers and foundation piles. Effective use of concrete in aggressive substructure applications could be realized constructively by an economical concrete confinement technique that uses a plastic tube to encase the concrete core in bridge piers and foundation piles, which may remarkably contribute to the ultimate strength and ductility (Abdulla, Citation2017). Encasing concrete with uPVC plastic forms has several advantages, including sealing the visible pores and concrete cover, preventing steel reinforcement from corrosion, and environmentally protecting the concrete infill.
The plastic tube’s benefit in enhancing the deformation of concrete has been recognized by several recent researches and accepted more by the engineering society. Such deformations are essential to increase the length of the falling branch of the stress-strain curve by increasing the strain at peak stress and the ultimate failure strain, which is required to increase the concrete member’s ductility and toughness.
Concrete confined by a prefabricated polypropylene (PP) jacket was tested under low-velocity impact loading using static and dynamic loads. The test results were compared with similar specimens confined by carbon fiber-reinforced polymer (CFRP) composites (Uddin & John, Citation2008). Specimens jacketed with PP reached a maximum strain, which was more than three times that for CFRP wrapped sample. The durability of high-density polyethylene (HDPE) tubes encasing Self-compacting concrete with and without steel fibers was explored after exposure to chloride environments (Kurtoglu et al., Citation2018). The increase in strain was a significant physical phenomenon observed after exposure to chemicals simulating aggressive environments.
Furthermore, a 50% enhancement in fracture energy was achieved when the tube thickness was increased by 30%. Engineering plastics were used to jacket concrete cylinders (Abdulla, Citation2020c). The two components’ deformations were monitored by introducing a small gap of 1.2 mm between the concrete and the polymeric tube. Test results show a ductile post-peak response with compression strain-softening and the formation of two peak strains. In another study (Woldemariam et al., Citation2020), Test results have illustrated that the uPVC confinement of concrete enhanced the ductility and energy absorption capacity by 1.84–15.3 and 11–24.3 times over that unconfined specimens. An artificial neural network (ANN) technique was employed to predict the axial strain of concrete-filled plastic tubular specimens tested under direct compression load (Abdulla, Citation2020e). Statistical evaluations demonstrated the soft computing technique to be more effective and accurate than several existing strength models for fiber-reinforced polymer (FRP)-confined concrete.
For mass concrete structures, the embedded water tube cooling system is considered a useful tool to monitor hydration temperature during construction (Hong et al., Citation2019). Due to its improved constructability and durability, Polymeric uPVC can be employed as an alternative to traditional steel or wood formworks (Abdulla, Citation2020e; Michel Murillo et al., Citation2019; Abdulla, Citation2020c). The seismic behavior of embedded PVC tube confined reinforced high-strength concrete columns was explored (Chen et al., Citation2020). The effects of PVC tube diameter, axial compression ratio, and concrete strength on seismic behavior indexes were analyzed.
PVC tube was used to encase cementitious composite materials. The compressive behavior of compound concrete-filled reinforced PVC tubes was compared to PVC tubes without steel reinforcement (Babu & Paulose, Citation2019). In marine and offshore infrastructure construction, foundation piles’ durability needs to be accounted for due to the corrosion from the salt in the seabed soil (Song et al., Citation2008). Intending to treat the corrosive foundation, stabilized soil confined by a PVC tube was used (Shiyang et al., Citation2018). The axial strain of specimens contained with the tube was 6.45–25.09 times the unconfined ones, respectively. PVC tube was employed for the beam-column joint construction. The deformation of joint core reinforced with a ring beam for connection of PVC fiber‐reinforced polymer confined concrete column, and a reinforced concrete beam was investigated (Bu et al., Citation2020).
Recent research (Jiequn et al., Citation2019) has shown that PVC tubes’ lifting effect strengthened with carbon fiber wraps not to be significant in recycled aggregate concrete (RAC). However, the CFRP wraps introduce a positive influence on incrementing the peak strain of uPVC-confined recycled aggregate concrete. The authors concluded that the plastic tube confinement could considerably enhance the strain at peak stress of RAC specimens. The effect of uPVC tube confinement on RAC’s peak strain is more significant than that of normal aggregate concrete. Compared with the elastic modulus of unconfined RAC, the PVC-RAC’s elastic modulus was improved by 12.9%. The improvement was increased to 27.3% when 50% of the aggregate was replaced by RA (Jiequn et al., Citation2019). This ratio was further increased to 29.8% when 100% RA was used. Generally, the low modulus plastic tube restrains concrete core to some extent from dilating laterally, enhancing its strength and ductility capacities (Chen et al., Citation2020; Michel Murillo et al., Citation2019; Abdulla, Citation2020c).
So far, there is no model for predicting the strain of uPVC tube confined concrete, which is one of the present study’s objectives. Another goal is to check the applicability of FRP-confined concrete strain models for predicting the strain of uPVC confined concrete specimens. Furthermore, the current work collects all the published data on a novel technique of concrete confinement using commercially available PVC or uPVC tubes with material and labor cost savings since the tube is lightweight and no skilled labor is needed. The number of test data for the strain of uPVC-concrete specimens reported in the literature is limited and is less than those for strength. However, the assembled database was abundant enough to accurately develop a maximum condition model for the peak axial strain.
4. Experimental program
4.1. Materials
Ordinary hydraulic Portland cement was used to cast concrete. The concrete was used for both the unconfined specimens and confined specimens made up of coarse river gravel with a 9.5 mm maximum size. The fine aggregate was river sand with a maximum size of 4.75 mm. All the specimens were cast from the same concrete, which was proportioned by weight. The mixture proportions were 1 (cement): 2 (sand): 2.75 (gravel). The water-cement ratio (w/c) was 0.5. The 28 days targeted cylindrical compressive strength was 40MPa.
4.2. Plastic tube
Grey color uPVC was cut to the required heights, and the ends ground and cleaned. Two average thicknesses of polymeric tubes were used in the present study, 5 and 7 mm, respectively. The plastic tubes were assembled on a stable timber base and used as a temporary mold for casting unconfined concrete specimens and as permanent formwork for casting the composite specimens. For tensile testing of uPVC, rods were cut from the tubes in the longitudinal direction, and the edges were finished for testing. The geometric details of the coupons are shown in .
5. Testing procedure
5.1. uPVC coupons
The coupons were assembled in the testing machine and hold vertically through the upper and lower grips . The coupons tested at a speed of 5 mm/min (as per ISO 6259–2PVC), and the average fracture strain was 42%. The specimen underwent linear elastic deformations up to the proportional limit, where the yield stage was initiated, resulting in the test specimen’s necking under direct tensile load due to the material yielding. The coupon continued to resist load until the peak load was reached. Beyond maximum load, the specimen underwent softening with a smooth plateau until it was split into two parts .
5.2. uPVC hollow tubes
Hollow uPVC tubes were tested under axial compression load at a speed of 1 mm/min. Hollow uPVC tubes were vulnerable to local buckling under direct axial load, which reduced their global stiffness. Only when the tube was loaded continued to shrink and fold in the axial direction and expand in the lateral direction, . Unlike in the tensile testing of uPVC coupons, where the split of the coupon terminated the test at the point of necking, the hollow tubes continued to resist load with substantial deformation reaching large ultimate strains exhibiting considerable buckling near the upper end. The test results for yield strength of coupons and hollows tubes were close.
5.3. uPVC-confined concrete
The uPVC tube confined concrete specimens were tested under axial compression in a universal testing machine, . Three unconfined specimens were tested to failure for each corresponding H/D ratio. A displacement-controlled procedure at a loading rate of 0.3 mm/min was used to test the confined and unconfined short columns. The test continued beyond the ultimate load to capture the falling branch of the stress-strain curve. The testing machine was switched off following PVC’s failure confined concrete or destruction of the unconfined specimens. Typical failure modes of specimens confined with a uPVC tube with a thickness of 5 mm were shown in . Two modes of failure observed; drum type and shear-type failure . The failure of uPVC tube confined columns with 7 mm thickness shown in before the test; (b) and (c) after the test.
6. Test results
6.1. Failure mode
Most of the confinement pressure that the tube can exert on concrete reached when the radial strain in the plastic tube reaches yield. The maximum confinement pressure is achieved when the compression stress reaches its ultimate or peak value. Beyond the peak stress, the tube continues to apply confinement pressure but on a smaller scale due to the low modulus of the tube. The maximum top strain in the tube is attained when the plastic tube’s circumferential strain reaches its burst pressure and, ultimately, the tube rupture resulting in the failure of the damaged concrete cylinder. Low stiffness is one of the main disadvantages of the tube. This limits the use of plastic tubes in structural applications into lightly loaded industrial and residential buildings. Other more convenient uses for the plastic tube are for piers and underground piles in infrastructure applications. The significance and the vital role of confinement in improving the lateral buckling of concrete columns is an essential pivoting factor when considering structural members’ safety against collapse. To avoid brittle catastrophic failure, the strength, and deformation capacity of confining material influence concrete members’ behavior and ensure a ductile flexural response, which was the case in the present study, and a further improvement in the function of the uPVC tube can be achieved using FRP wraps or strips.
6.2. uPVC coupons
The uPVC coupon exhibit no clear yielding, and the yield stress has to be approximated from 0.2% strain, a parallel line that offsets the abscissa at 0.2% strain and cuts the stress-strain curve.
6.3. uPVC tube confined concrete
The thin-walled plastic tube was subjected to biaxial stress due to the applied direct compression load and the transfer of the axial loads from the core concrete to the external confining device. This was evident in plastic tubes having a thickness of 5 mm, where the tube underwent considerable outward buckling deformations, resulting in a bowel type failure. When the tube’s thickness was increased to 7 mm, less axial load was transferred from the concrete core. The tube exhibited less deformations due to the improvement in its geometrical properties, . Generally, simultaneous axial loading of both concrete core and confinement tube yields enhancement in the strength of PVC-confined concrete due to interfacial bonding between the two components, which improves the global stiffness of test specimens. When the hollow tube is under direct load, it undergoes deformations in axial and lateral directions resulting in local buckling. When the uPVC—confined concrete is tested, the encasing tube’s inward deformations were ceased . In the stress-strain curve of PVC-confined concrete, , an initial linear portion of the stress-strain curve is maintained up to about 70–80% of the ultimate load. With further increase in the maximum load, the curve becomes non-linear, and a non-sharp peak is reached. Beyond the peak, the stress-strain relationship undergoes compression strain-softening with a noticeable drop in stress (25–35% of peak load) and an increase in the corresponding strain. After that, the curve stabilizes, and small declines in stress being registered with corresponding large increments in strain. All the test results for the ultimate stress and strain of uPVC-confined concrete columns were summarized in . The ability of uPVC-confined concrete to absorb mechanical energy continues with a large increase in strain and a continuous marginal drop in stress up to the point of ultimate strain—the toughness increases due to the rise in the area under the stress-strain curve. The load capacity of confined specimens showed a slightly increasing trend with the increase in tube thickness. When the outer diameter to thickness ratio (D/t) changed from 22 to 12.9, local buckling of the polymeric tube decreased, and the strain at peak stress was increased. The increment in H/D ratio had adversely influenced the strength.
Table 1. Ultimate stress and strain of uPVC-confined concrete
Engineers must seek alternative confining materials to improve structural members’ performance in displaying sufficient deformations and strain softening past peak load. The confinement device was efficient in dissipating the internal strain energy and develops adequate ductility. However, for additional load capacity achievement, fiber-reinforced polymers (FRP) could be externally applied to the tubular shell for further lateral confinement, leading to improved overall tube toughness. Recent research (Jiequn et al., Citation2019) has shown that the PVC tube confinement can enhance the elastic modulus of plastic tube-confined recycled concrete.
6.4. Experimental database
An extensive review of published test data on PVC-confined concrete was conducted. Only the studies that report the stress and strain values were considered. The compiled database covers the test results of PVC-confined concrete columns of circular cross-sections under direct axial compression load. The final database covered the following details for each specimen: geometric properties of the uPVC tube, diameter (D), thickness (t), and height (H); material properties of the uPVC tube, tensile yield strength (fyp), elastic modulus (E), confined strength of uPVC-confined concrete at the peak of the stress-strain relationship (f’cc) and the strain (Ԑ’cu) at the peak stress; unconfined concrete cylinder strength (f’co) and strain (Ԑ’co). The final database meets the following criteria’s:
1- Confinement methods; full jacketing, where only the confined specimens with no gaps at top and bottom were included.
2- The specimens included in the database failed due to uPVC tube yielding.
3- To consider the size effect, small-sized and large-sized specimens were considered.
4- Specimens with internal steel reinforcement were excluded.
All the details of the compiled database are summarized in . One hundred- sixty test data were gathered from 15 different studies (Abdulla, Citation2014, Citation2019, Citation2020a, Citation2020b, Citation2020d, Citation2020f; Askari et al., Citation2020; Azeez et al., Citation2018; Fakharifar & Chen, Citation2016; Fakharifar et al., Citation2014; Jiang et al., Citation2012; Marzouck & Sennah, Citation2002; Woldemariam et al., Citation2019; Saadoon, Citation2010; Wang & Yang, Citation2012), published between 2002 and 2020. One relevant detail of the tube when it was not given was assumed as follows: fyp = 35MPa (Abdulla, Citation2020f).
Table 2. Experimental database of uPVC-confined concrete under direct load
6.5. Methods of evaluation
Three statistical indexes were employed to evaluate the applicability of the existing twenty strain models of FRP-confined concrete for predicting the strain of PVC-confined concrete. The statistical indexes refer to important additional details of the predicted data.
1- The average absolute error (AAE) given by the following equation:
AAE = (1)
The AAE close to zero gives the best model with the highest precision. The second index was the average ratio (AR), which is equal to:
AR = (2)
The perfect AR value, which equals one, displays the boundary between the underestimation (AR<1), where the predicted value is less than the experimental value, and overestimation (AR>1), where the predicted value is more than the observed value. A third statistical indicator used to assess the performance of the models given by:
3- Coefficient of correlation (R2)
R2 = (3)
Where the model prediction is expressed by (model.i), the experimental value represented by (exp.i), N is the total number of data.
6.6. Strain models
Twenty existing FRP-confined concrete strain models (Al-Tersawy et al., Citation2007; Benzaid et al., Citation2010; Binici, Citation2008; Ciupala et al., Citation2007; Fardis & Khalili, Citation1982; Gao et al., Citation2019; Ilki et al., Citation2002, Citation2004; Jiang & Teng, Citation2007; Mandal et al., Citation2005; Miyauchi et al., Citation1997, Citation1999; Saadatmanesh et al., Citation1994; Saafi & Li, Citation1999; Samaan et al., Citation1998; Shehata et al., Citation2002; Toutanji, Citation1999; Wu et al., Citation2009; Yan & Pantelides, Citation2007; Youssef et al., Citation2007) were used to examine these models’ applicability to predict the strain of PVC tube-confined concrete short columns subjected to axial compression loads. Although the FRP-confined concrete displays a bilinear response with the ascending branch, a descending branch is also possible depending on the confinement level, which is the case with PVC-confined concrete, which usually exhibit compression softening with a descending branch of the stress-strain curve. Several test parameters used in the strain models of FRP-confined concrete can evaluate the strain of the uPVC tube confined concrete. However, one parameter is different: the fiber rupture strain εh,rup, and it was replaced with the strain at peak stress Ԑ’cu.
7. Discussions
7.1. Data range
A 160 test data database for strain results of uPVC tube-confined concrete was compiled from the published literature combined with the eighteen test data from the present study. The range of geometric properties of uPVC tube used in the database was as follows; thickness of uPVC tube varies from 2.5 mm (Woldemariam et al., Citation2019) to 8.5 mm (Wang & Yang, Citation2012), external tube diameter ranged from 68 mm (A. M Woldemariam et al., Citation2019) to 168 mm (Fakharifar & Chen, Citation2016), height from 38 mm (Abdulla, Citation2014) to 600 mm (Jiang et al., Citation2012). The ratio of εcc/εco ranged from 1.28(Abdulla, Citation2014) to 8.27 (A. M Woldemariam et al., Citation2019), except one data was below one, 0.97 (Jiang et al., Citation2012). The strengthening ratio of columns in the database ranged from 0.87 (Abdulla, Citation2014) to 2.36 (A. M Woldemariam et al., Citation2019), and the corresponding confinement ratio (fl/f’co) of ranged from 0.05 (Askari et al., Citation2020) to 0.45 (Wang & Yang, Citation2012). To not have a post-peak descending branch, the ACI code recommends the confinement effectiveness ratio (fl/f’co) to be larger than 0.08, adopted from the studies (Lam & Teng, Citation2003a, Citation2003b).
One significant difference between the FRP and PVC tube is in the low modulus of the latter. Another difference is in the maximum axial stress (Ԑ’cu), where the ACI 440.2 R (ACI Citation2008) (ACI 440-2R (ACI2008 American Concrete Institute (ACI), Citation2008) restricts this to a maximum value of 0.01 to avoid excessive cracking and maintain structural integrity (Yazici & Muhammad, Citation2012). This limit was exceeded in the assembled database in several cases due to the uPVC tube’s elastic-plastic behavior, unlike FRP’s only-elastic behavior (Jiang et al., Citation2012).
7.2. Strain predictions
The strain predictions using the existing twenty FRP-confined Concrete models were plotted versus the experimental strain ratios, . The accurate prediction line is reached by a straight line that yields a 45° angle to the test results axis. For some model predictions, the data was largely scattered, Models (Binici, Citation2008; Ciupala et al., Citation2007; Fardis & Khalili, Citation1982; Ilki et al., Citation2002; Mandal et al., Citation2005; Miyauchi et al., Citation1999; Saadatmanesh et al., Citation1994; Saafi & Li, Citation1999; Samaan et al., Citation1998; Toutanji, Citation1999). For other model predictions, the data were clustered into a narrow strip, (, , , ). For a couple of models, it was also observed that a few predictions were even negative. For experimental fcc/fco results greater than 2, several models (Al-Tersawy et al., Citation2007; Benzaid et al., Citation2010; Binici, Citation2008; Ciupala et al., Citation2007; Fardis & Khalili, Citation1982; Ilki et al., Citation2002; Jiang & Teng, Citation2007; Mandal et al., Citation2005; Miyauchi et al., Citation1997, Citation1999; Saadatmanesh et al., Citation1994; Saafi & Li, Citation1999; Samaan et al., Citation1998; Toutanji, Citation1999; Wu et al., Citation2009) yielded unconservative estimates of experimental εcc/εco values, whereas Models (Ilki et al., Citation2004; Shehata et al., Citation2002; Yan & Pantelides, Citation2007) produced conservative estimation. Two models (Gao et al., Citation2019; Youssef et al., Citation2007) showed reasonable predictions. For confinement effectiveness fl/fco ratio 0.3 (A. M Woldemariam et al., Citation2019; Wang & Yang, Citation2012) or > (0.45) (Wang & Yang, Citation2012), several models (Al-Tersawy et al., Citation2007; Binici, Citation2008; Ciupala et al., Citation2007; Fardis & Khalili, Citation1982; Ilki et al., Citation2002; Mandal et al., Citation2005; Miyauchi et al., Citation1997, Citation1999; Saafi & Li, Citation1999; Toutanji, Citation1999) overestimated the experimental Ԑ’cu/Ԑ’co values, whereas Models (Gao et al., Citation2019; Ilki et al., Citation2004; Saadatmanesh et al., Citation1994; Yan & Pantelides, Citation2007; Youssef et al., Citation2007) underestimated the experimental εcu/εco values. This could be ascribed to variation in the confinement effectiveness factor or constant slope of the equation. The accuracy of the models was evaluated using statistical indexes, which were also used for comparison purposes. The following information was assessed; average or mean value, standard deviations, AAE, AR, and the regression coefficient R2. The predicted value was computed, and the difference with the test value gives the error for each data point. The model providing a smaller sum of errors was accepted as a better model than the other models. The model (Miyauchi et al., Citation1997) could result in larger average absolute errors.
The percentage of AA error produced by the twenty models was below 100%. The only model (Miyauchi et al., Citation1997) with 127.07% exceeding this limit, and model (Miyauchi et al., Citation1997) has two expressions valid for concrete strength of fco = 30MPa and fco = 50MPa, respectively.
The model that yielded the least AA error was model (Wu et al., Citation2009) with an AAE value of 27.23%. For most of the model predictions the margin of error lies in the range 30 to 50% (Benzaid et al., Citation2010; Ciupala et al., Citation2007; Fardis & Khalili, Citation1982; Gao et al., Citation2019; Jiang & Teng, Citation2007; Saadatmanesh et al., Citation1994; Saafi & Li, Citation1999; Toutanji, Citation1999) and between 50 to 80% (Binici, Citation2008; Ilki et al., Citation2002, Citation2004; Mandal et al., Citation2005; Samaan et al., Citation1998; Shehata et al., Citation2002; Yan & Pantelides, Citation2007; Youssef et al., Citation2007). The magnitude of the second index AR varied from 0.305 (Ilki et al., Citation2004) to 2.25 (Miyauchi et al., Citation1997). When AR is 0.305, all the data fall below the 45° best fitting line and below the lower 20% error line (. As AR’s value increased to 2.25, almost all the data points shifted up . it’s worth mentioning that the performance of simple models (Wu et al., Citation2009) was better compared with some existing, more complicated models (Binici, Citation2008; Yan & Pantelides, Citation2007). For the third index, for most FRP model predictions, the values of R2 lay below 0.5 and give a poor indication of the error for each data point.
7.3. Predictive expression for strain at peak axial strength of PVC-confined concrete
The analysis shows that a more suitable strain model is needed, considering more experimental parameters. Several studies have pointed out the difficulty in modeling and predicting confined concrete strain (Yazici & Muhammad, Citation2012). Based on the comprehensive database, most of the FRP strain models were not suitable for predicting the strain of uPVC tube-confined concrete specimens. Only one model (Wu et al., Citation2009) , showed reasonable predictions, with AAE and AR values of 27.23% and 0.84, respectively. Therefore, an attempt was made in the present work to develop a model that includes most of the parameters given in the database as follows:
from multi-linear regression analysis, the values of constants were evaluated and inserted in EquationEq. (4(4)
(4) ), yielding the following strain model:
The strain predictions using the proposed model were plotted versus the experimental values, . Compared with the FRP models, the proposed model’s strain predictions were considerably discrete, with AAE and AR equal to 26.7% and 0.9, as shown in . The developed model captured the test data of the database with acceptable accuracy. Several studies pointed out the fact that the computed average absolute error values for Ԑ’cu /’co were more significant than that of fcc/fco estimates. An average absolute error of 30% is not unfamiliar for εcu/εco, and a number of studies on FRP-confined concrete studies have reported similar values (Yazici & Muhammad, Citation2012). In a similar finding, four existing FRP strain models were used to predicate the strain of FRP-confined concrete columns under cyclic axial loading based on a comprehensive experimental database (Fanaradelli & Rousakis, Citation2020), yielding predictions with AAE values close to or higher than 50%.
8. Conclusions
Three experimental parameters, tube diameter, tube thickness, and H/D ratio, were used to examine the mechanical behavior of eighteen short PVC tube confined concrete. Based on an experimental database of 160 test data combined with the 18 test data of the present study, the following conclusion can be drawn:
1- The thin-walled plastic tube was subjected to biaxial stress due to the applied direct compression load and the transfer of the axial loads from the core concrete to the external confining device. This was evident in plastic tubes having a thickness of 5 mm, where the tube underwent considerable outward buckling deformations resulting in a bowel type failure. When the tube’s thickness was increased to 7 mm, less axial load was transferred from the concrete core. The tube exhibited less deformations due to the improvement in its geometrical properties.
2- The three test variables influenced the performance of uPVC-confined concrete, where the increase in tube thickness reduced buckling in the thin-walled plastic tube and increased the strain at peak stress of uPVC-confined concrete. The increase in the H/D ratio had adversely influenced the strength.
3- The PVC tube confinement device enhanced the strain at peak stress of concrete by 1.62–3.29 times compared with unconfined specimens.
4- Twenty of the existing strain models for FRP-confined concrete were used to predict the strain ratio εcu/εco for uPVC-confined concrete. All the models yielded AAE values above 30%, with only one model with an AAE value of 27.23%.
4- Based on a comprehensive database of 178 test data, a strain model was developed for predicting the strain at peak stress of PVC tube-confined concrete. The developed model’s accuracy was verified by comparing model predictions with twenty existing FRP-confined concrete models using three statistical indexes. The distinguishing feature of the proposed strain model is that it includes the influence of several parameters on the concrete confined by the uPVC tube.
5- The plastic tube’s significance and vital role in improving the lateral buckling of concrete columns is a crucial pivoting factor when considering structural members’ safety against the non-ductile brittle collapse of the concrete core.
A database of confined columns that undergo strain softening in the stress-strain curve’s descending branch is more critical than the columns with second ascending branch FRP-confined columns. Therefore, it is imperative to predict PVC columns’ deformations with reasonable precision at failure for structural applications. Hence more research and effort are needed to improve the developed models’ performance, which requires more design variables, such as length effect and different loading conditions. Research is needed to establish an analytical technique to predict the stress-strain of PVC tube-confined concrete under axial load.
Acknowledgements
This research did not receive any specific grant from funding agencies in the public, commercial, or not-for-profit sectors.
Additional information
Funding
Notes on contributors
Nwzad Abduljabar Abdulla
Dr. Abdulla NA is an Assistant Professor at the Department of Civil Engineering, College of Engineering, University of Salahaddin, Erbil. Iraq. His research interest includes composite structures, uPVC-confined concrete, and structural recycled concrete elements.
References
- Abdulla, N. A. (2014). concrete filled thermoplastic tube under compression first international engineering conference (IEC2014). p(60–27)
- Abdulla, N. A. (2017). Concrete filled PVC tube: A review. Construction and Building Materials, 156, 321–329. https://doi.org/10.1016/j.conbuildmat.2017.08.156
- Abdulla, N. A. (2019). Influence of plastic pour-in form on the mechanical behavior of concrete. Structures, 19, 193–202. https://doi.org/10.1016/j.istruc.2019.01.007
- Abdulla, N. A. (2020a). Concrete encased with engineering plastics. Journal of Civil Engineering and Construction, 9(1), 31–41. https://doi.org/10.32732/jcec.2020.9.1.31
- Abdulla, N. A. (2020b). Mechanical behavior of slender composite columns under axial compression load. KSCE Journal of Civil Engineering, 24(1), 208–218. https://doi.org/10.1007/s12205-020-0669-y
- Abdulla, N. A. (2020c). Concrete with an outer plastic protective shell. SN Applied Sciences, 2, 1093. https://doi.org/10.1007/s42452-020-2901–2
- Abdulla, N. A. (2020d). The Behavior of concrete-filled Plastic Tube Specimens under Axial Load, Jordan. Journal of Civil Engineering, 14(1), 69–81
- Abdulla, N. A. (2020e). Using the Artificial Neural Network to Predict the Axial Strength and Strain of Concrete-filled Plastic Tube. Journal of Soft Computing in Civil Engineering. https://doi.org/10.22115/scce.2020.225161.1198 27 doi:10.1016/j.istruc.2020.07.061
- Abdulla, N. A. (2020f). Axial strength of short concrete-filled plastic tubes. Structures, 27, 1786–1800. https://doi.org/10.1016/j.istruc.2020.07.061
- ACI 440-2R (ACI2008 American Concrete Institute (ACI). (2008). “Guide for the design and construction of externally bonded FRP systems for strengthening concrete structures.”. MI. 440.2R-08.
- Al-Tersawy, S. H., Hodhod, O. A., & Hefnawy, A. A. (2007). Reliability and code calibration of RC short columns confined with CFRP wraps. In: Proc. 8th int. symp. on fiber-reinforced polymer reinforcement for concrete structures. Patras, Greece: Univ. of Patras; 2007
- Askari, S. M., Khaloo, A., Borhani, M. H., & Tale Masoule, M. S. (2020). Performance of polypropylene fiber reinforced concrete-filled UPVC tube columns under axial compression. Construction and Building Materials, P(1–16).
- Azeez, A. A., Jamaluddin, N., Rahman, N. A., Hassen, D. R., & Attiyah, A. N. (2018). Experimental and analytical study of PVC confined concrete cylinders. Journal of Engineering Applied Sciences, 13(8), 2145–2151.
- Babu, B., & Paulose, H. (2019). Behavior of Compound Concrete Filled Reinforced PVC Tubes Under Compression. International Research Journal of Engineering and Technology(IRJET)e- 2395–0056, 06(04).
- Benzaid, R., Mesbah, H., & Chikh, N. (2010). FRP-confined concrete cylinders: Axial compression experiments and strength model. J ReinfPlas Compos, 29(16), 2469–2488.
- Binici, B. (2008). Design of FRPs in circular bridge column retrofits for ductility enhancement. Eng Struct, 30(3), 766–776. https://doi.org/10.1016/j.engstruct.2007.05.012
- Bu, S., Yu, F., Feng, C., Liu, Q., song, J., & Fang, Y. (2020). Local compressive behavior of joint core reinforced with ring beam for connection of polyvinyl chloride fiber‐reinforced polymer confined concrete column and reinforced concrete beam. Structural Concrete., 21, 1523–1543. https://doi.org/10.1002/suco.201900312
- Chen, Z., Liang, Y., & Zhao, X. (2020). Investigation on Hysteretic Behavior of Embedded PVC Pipe Confined Reinforced High Strength Concrete Columns. Materials, 13(3), 737. https://doi.org/10.3390/ma13030737
- Ciupala, M. A., Pilakoutas, K., & Mortazavi, A. A. Effectiveness of FRP composites in confined concrete. In: Proc. 8th int. symp. on fiber reinforced polymer reinforcement for concrete structures. Patras, Greece Univ. of Patras; 2007.
- Fakharifar, M., & Chen, G. (2016). Compressive behavior of FRP-confined concrete-filled PVC tubular columns. Composite Structures, 141, p 1–19. https://doi.org/10.1016/j.compstruct.2016.01.004
- Fakharifar, M., Chen, G., Lin, Z., & Woolsey, Z. (2014). Behavior and strength of passively confined concrete-filled tubes. In: The 10th US national conference on earthquake engineering: July. Anchorage, AK..
- Fanaradelli, T., & Rousakis, T. (2020). Assessment of analytical stress and strain at peak and ultimate conditions for fiber-reinforcement polymer confined reinforced concrete columns of rectangular sections under axial cyclic loading. Structural Concrete, 1–14.
- Fardis, M. N., & Khalili, H. (1982). FRP-encased concrete as a structural material. Mag Concr Res, 34(122), 191–202. https://doi.org/10.1680/macr.1982.34.121.191
- Gao, C., Huang, L., & Yan, L. (2019). Strength and ductility improvement of recycled aggregate concrete by polyester FRP-PVC tube confinement. Composites Part B, 162, 178–197. https://doi.org/10.1016/j.compositesb.2018.10.102
- Hong, Y., Lin, J., & Chen, W. (2019, February). Simulation of thermal field in mass concrete structures with cooling pipes by the localized radial basis function collocation method. International Journal of Heat and Mass Transfer, Volume 129, 449–459. https://doi.org/10.1016/j.ijheatmasstransfer.2018.09.037
- Ilki, A., Kumbasar, N., & Koc, V. (2002). Strength and deformability of low strength concrete confined by carbon fibre composite sheets. In: Proc. 15th eng. mech. Conference. New York:Columbia Univ.; 2002
- Ilki, A., Kumbasar, N., & Koc, V. (2004). Low strength concrete members externally confined with FRP sheets. Struct Eng Mech, 18(2), 167–194. https://doi.org/10.12989/sem.2004.18.2.167
- Jiang, S., Dai, T., Fu, D., Wu, Z., & Li, N. (2012). Experimental study on concrete columns confined by BFRP-PVC tubes under uniaxial loading. Journal of Shenyang Jianzhu University (Natural Science), 28(1), 23–29.
- Jiang, T., & Teng, J. G. (2007). Analysis-oriented stress-strain models for FRP-confined concrete. ASCE J Eng Struct, 29(11), 2968–2986. https://doi.org/10.1016/j.engstruct.2007.01.010
- Jiequn, L., Tian, Y., Chen, J. G., & Zhu, C. Y. (2019). Experimental Study on CFRP-PVC Confined RAC under Axial Compression. Solid State Phenomena, 294, pp 143–149
- Kurtoglu, A. E., Hussein, A. K., & Gulsan, M. E. (2018). Mechanical investigation and durability of HDPE-confined SCC columns exposed to severe environment. KSCE J Civil Eng, 22, 5046. https://doi.org/10.1007/s12205-017-1533-6
- Lam, L., & Teng, J. G. (2003a). Design-oriented stress-strain model for FRP-confined concrete. Construction and Building Materials, 17(6–7), 471–489. https://doi.org/10.1016/S0950-0618(03)00045–X
- Lam, L., & Teng, J. G. (2003b). “Design-oriented stress-strain model for FRP-confined concrete in rectangular columns.”. J. Reinf. Plast. Compos., 22(13), 1149–1186. https://doi.org/10.1177/0731684403035429
- Mandal, S., Hoskin, A., & Fam, A. (2005). Influence of concrete strength on confinement effectiveness of fiber-reinforced polymer circular jackets. ACI Struct J, 102(3), 383–392.
- Marzouck, M., & Sennah, K. (2002), “concrete-filled PVC tubes as compression members: Composite Materials in Concrete Construction”, Proceedings of the international congress “challenges of concrete construction, 31–38.
- Michel Murillo, A., Tutikian, B. F., Ortolan, V., Marcos, L. S., & Oliveira, C. H. (2019). Fire resistance performance of concrete-PVC panels with polyvinyl chloride (PVC) stay in place (SIP) formwork. Journal of Materials Research and Technology, 8(Issue 5), 4094–4107.
- Miyauchi, K. and et al. (1997) “Estimation of strengthening effects with carbon fiber sheet for concrete column”. Proceedings of the third international symposium (FRPRCS-3) on Non-Metallic (FRP) Reinforcement for concrete structures, Sapporo, Japan, pp. 217–224
- Miyauchi, K., Inoue, S., Kuroda, T., & Kobayashi, A. (1999). Strengthening effects with carbon fiber sheet for concrete column. Japan Concr. Inst.
- Saadatmanesh, H., Ehsani, M. R., & Li, M. W. (1994). Strength and ductility of concrete columns externally reinforced with fiber composite straps. ACI Struct J, 91(4), 434–447.
- Saadoon, A. S. Experimental and Theoretical Investigation of PVC-Concrete Composite Columns. Ph.D. Thesis 2010: 23–134
- Saafi, H., & Li, T. Z. (1999). Behavior of concrete columns confined with fiber-reinforced polymer tubes. ACI Material Journal, 96(4), 500–509.
- Samaan, M., Mirmiran, A., & Shaway, M. (1998). Model of concrete confined by fiber composites. Journal of Structure Engineering, ASCE, 124(9), 1025–1031. https://doi.org/10.1061/(ASCE)0733-9445(1998)124:9(1025)
- Shehata, I. A. E. M., Carneiro, L. A. V., & Shehata, L. C. D. (2002). Strength of short concrete columns confined with CFRP sheets. Mater Struct, 35, 50–58.
- Shiyang, L., Huang, X., Shuaishuai, W., Tian, J., & Zhang, W. (2018). Bearing capacity of stabilized soil with expansive component confined by polyvinyl chloride pipe. Construction and Building Materials, 175, 307–320. https://doi.org/10.1016/j.conbuildmat.2018.04.188
- Song, H. W., Lee, C. H., & Ann, K. Y. (2008). Factors influencing chloride transport in concrete structures exposed to marine environments. Cem. Concr. Compos, 30(2), 113–121. https://doi.org/10.1016/j.cemconcomp.2007.09.005
- Toutanji, H. A. (1999). Stress-strain characteristics of concrete columns externally confined with advanced fiber composite sheet. ACI Structural Journal, 96(3), 397–405.
- Uddin, N., & John, D. (2008). Purdue; and Uday Vaidya, Feasibility of Thermoplastic Composite Jackets for Bridge Impact Protection. Journal of Aerospace Engineering, 21, 259–265. https://doi.org/10.1061/(ASCE)0893-1321(2008)21:4(259)
- Wang, J. Y., & Yang, Q. (2012). Investigation on compressive behaviors of thermoplastic pipe confined concrete. Construct Build Mater, 2012(p35), 578–585
- Woldemariam, A. M., Oyawa, W. O., & Nyomboi, T. (2019). Structural performance of UPVC confined concrete equivalent cylinders under axial compression loads. Buildings, 2019(P), 1–25.
- Woldemariam, A. M., Oyawa, W. O., & Nyomboi, T. (2020). Experimental studies on the behavior of concrete-filled uPVC tubular columns under axial compression loads. Cogent Engineering, 7, 1768649. https://doi.org/10.1080/23311916.2020.1768649
- Wu, H., Wang, Y., Yu, L., & Li, X. (2009). Experimental and computational studies on high strength concrete circular columns confined by aramid fiber-reinforced polymer sheets. ASCE J Compos Constr, 13(2), 125–134. https://doi.org/10.1061/(ASCE)1090-0268(2009)13:2(125)
- Yan, Z., & Pantelides, C. P. Design-oriented model for concrete columns confined with bonded FRP jackets or post-tensioned FRP shells. In Proc. 8th int. symp. on fiber-reinforced polymer reinforcement for concrete structures. Patras, Greece: Univ. of Patras; 2007.
- Yazici, V., & Muhammad, N. S. (2012). Hadi, Normalized Confinement Stiffness Approach for Modeling FRP-Confined Concrete, J. Compos. Constr., 16, 520–528. https://doi.org/10.1061/(ASCE)CC.1943-5614.0000283
- Youssef, M. N., Feng, M. Q., & Mosallam, A. S. (2007). Stress-strain model for concrete confined by FRP composites. Compos Part B: Eng, 38(5–6), 614–628. https://doi.org/10.1016/j.compositesb.2006.07.020