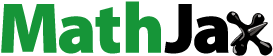
Abstract
Drying is an important process that extends the storage life and retains the quality of onion. Unfortunately, convective dyers with poor engineering designs and construction show low thermal efficiency for onion drying. This work aims to study the effects of recycled exhaust air on the thermal efficiency of onion slice drying at various temperatures and drying capacities. The exhaust air leaving the drying chamber was recirculated and mixed with fresh air to reduce the preheating load. The thermal efficiency of the proposed system was calculated and compared with the results of a standard drying process without exhaust air recycling. Findings showed that the average thermal efficiency of the modified dryer improved with increasing drying temperature, drying capacity, and recycled exhaust air-to-fresh air ratio. Optimization via a central composite design revealed an optimal thermal efficiency of 58.82% at a drying temperature of 67.94°C, drying capacity of 0.089 kg of onion slices, and recycled exhaust air-to-fresh air ratio of 4.0. The findings of this work suggest that significant cost reduction in onion slice drying may be achieved by optimizing the recycled exhaust air-to-fresh air ratio and drying capacity of the dryer.
PUBLIC INTEREST STATEMENT
This work aims to improve thermal efficiency of onion slice drying by recycling exhaust air exiting the dryer. As we know that the air leaving dryer still contains amount of heat which can be directly recovered. Here, the exhaust air was recirculated and mixed with fresh air in certain ratio to reduce the preheating load. The result showed that the average thermal efficiency of the modified dryer improved with increasing drying temperature, drying capacity, and recycled exhaust air-to-fresh air ratio. The optimal efficiency of 58.82% was achieved at a drying temperature of 67.94°C, drying capacity of 0.089 kg of onion slices, and recycled exhaust air-to-fresh air ratio of 4.0. The findings of this work imply that incorporation of recycled exhaust air could remarkably reduce energy costs during onion slice drying.
1. Introduction
Onion (Allium cepa) bulbs are commonly used as a food seasoning and rich in flavonoid compounds and vitamin C (Metrani et al., Citation2020). Postharvest processing, such as drying, strongly affects the preservation of these compounds. The drying of fresh onion aims to remove moisture on the outer surface layer of the crop to inhibit undesirable microbial activity and germination and enable the extended storage of the bulbs (Bourdoux et al., Citation2016).
In general, onion bulbs are purchased from traditional markets for direct use as a food seasoning. However, many consumers are gradually adjusting to the use of sliced or powdered onion for the flexibility these onions forms offer (Bamba et al., Citation2020). In the food industry, onion is often processed as an instant seasoning in the form of dried slices or powder (Edith et al., Citation2018). Some bioactive substances and vitamin C contents of dried onion slices or powder are lost following exposure to high air drying temperatures. Sasongko et al. (Citation2020) reported that higher drying temperatures can exacerbate phenolic compound degradation. However, when the air drying temperature and relative humidity are decreased to 50°C and <10%, respectively, phenolic compound retention may be as high as 96%. Djaeni et al. pointed that thiamine retention is very low when conventional drying is conducted at 50–70°C. For example, at a drying temperature of approximately 50°C for 5 h, only 14% thiamine retention is achieved (Djaeni & Arifin, Citation2017). Fresh onion bulbs dried at 40–60°C show degradation of their physicochemical properties, including color, quercetin content, and antioxidant activity, with increasing drying time (Djaeni et al., Citation2016). The use of dehumidified air as a drying medium could shorten the drying time of onion bulbs and preserve their bioactive compounds (Atuonwu et al., Citation2011; Sasongko et al., Citation2020).
The main drawbacks of onion drying include massive energy usage and low thermal efficiency. The thermal efficiency of conventional convective drying is less 50% (Kemp, Citation2014), which translates to only 10–20% total energy usage. Although solar drying could minimize energy costs, the technique usually requires longer drying times and is highly dependent on weather conditions (Tiwari, Citation2016). Dehumidified air drying has been reported to be a successful method to improve the energy efficiency of food drying (Djaeni et al., Citation2011). Relative air humidity close to 0% or air moisture content (MC) of up to 0.1 ppm and temperatures in the range of 10–40°C could potentially enhance the driving force for moisture transfer from the onion surface to the drying air. Hence, the drying time could be shortened, and heat could be utilized more efficiently (Sasongko et al., Citation2020). Asiah et al. (Citation2017) applied air dehumidification as a drying medium to reduce the free moisture on the surface or outer layer of fresh onion bulbs to approximately 12%. In this state, fresh onions can be preserved from germination during storage. Results showed that dehumidified air can significantly reduce the drying time at drying temperatures ranging from 40to 50°C.
In the case of onion slice drying, both free and bound moisture must be removed to reduce the MC of the slices to approximately 20%. Free moisture can easily be evaporated by lowering the air humidity of the drying medium, but the bound moisture within the tissues of the onion layers requires more heat to eliminate. Heat is required to break the physical interactions between moisture and the tissues of the onion layers, resulting free moisture. More heat is then required to evaporate the free moisture. The heat required for these processes could be doubled and reduce the drying efficiency of the system (Strumilto et al., Citation2014). The time required to dry onion slices and reduce their MC from 86 to 88% to less than 10% by conventional drying is approximately 4 hours; however, the energy efficiency of this system is only approximately 35% (Nwakuba et al., Citation2017). A previous study showed that dehumidified air drying reduces the drying time to 0.8 hours, but no attempt to improve the energy efficiency of the system was reported (Sasongko et al., Citation2020).
Energy efficiency may be enhanced by recycling the exhaust air leaving the drying chamber; this exhaust air contains potential sensible heat and, thus, can evaporate moisture from food products (Djaeni et al., Citation2007; Krokida & Bisharat, Citation2004). The exhaust air can also be recirculated during drying to minimize heat losses from the dryer. Existing dryer designs may be upgraded by installing systems to mix the exhaust air leaving the heater with fresh air of a low relative humidity. The main benefit of such an upgraded system is that exhaust air can be directly reused; this improvement reduces the amount of fresh air entering the dryer for subsequent drying (Djaeni et al., Citation2007).
The current work aims to evaluate the effect of recycled exhaust air on the thermal efficiency of onion slice drying. Ambient air was heated to a certain temperature before feeding to the drying chamber, and then the exhaust air exiting the dryer was recirculated and mixed with incoming fresh air to minimize heat utilization. The thermal efficiency of this system was estimated under various drying conditions and compared with the results of standard drying without a recycling system.
2. Materials and methods
2.1. Materials
Onion (A. cepa; cultivar, Bima) with an initial moisture content (MC) of 86.02% ± 0.66% (wet basis) or 6.15 ± 0.33 kg water/kg dry solid (dry basis), as determined according to the gravimetric method, was used in this work (Shreve et al., Citation2006). The onions were sliced into 1.233 ± 0.029 mm-thick slices, as measured by a vernier caliper. Before slicing, the fresh onion was stored in a cool, dry, well-ventilated room at ambient conditions (27–29°C).
2.2. Methods
2.2.1. Convective dryer
The convective dryer used for onion slice drying is shown in . Ambient air with a relative humidity of 77% ± 1.53% and temperature of 30.33°C ± 1.53°C (measured by KW0600561, Krisbow®, Indonesia) was supplied by a blower through a pipe with an inside diameter (ID) of 12 cm and linear velocity of 1.78 m/s (measured by KW0600562, Krisbow®). The air was heated to a certain drying temperature (e.g., 50°C) in an electric preheater equipped with a thermo-regulator. The hot air was channeled to the tray dryer (30 cm × 50 cm) loaded with 0.025 kg of fresh onion slices. The MC of the onions was recorded every 30 minutes for 180 minutes. Three experimental temperatures were tested (i.e., 50°C, 60°C, and 70°C), and experiments were conducted in two replicates. The mean values of the experiments were obtained and reported as results.
Figure 1. Schematic of the onion slice drying system: 1) Blower, 2) Temperature–relative humidity sensor, 3) Velocity–temperature sensor, 4) Heater, 5) Drying chamber, 6) Valve
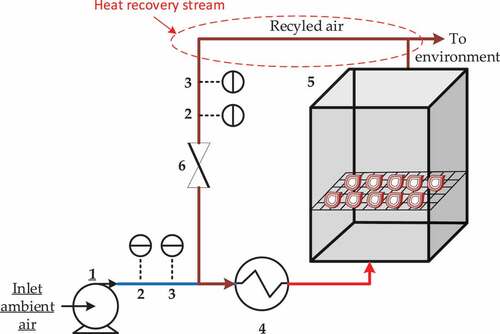
The data were used to estimate the drying rate via Newton’s model, as shown in Equationequation 1(1)
(1) (Mota et al., Citation2010). The thickness and diameter of the onion before and after drying process were observed. The thin layer of onion resulted the small difference changes of thickness and diameter after drying process, as presented in . Meanwhile, the hot air in dryer can be considered that it was homogenously distributed in cross sectional direction (). So, the moisture evaporation and heat transfer were uniform. Moreover, the volume shrinkage could be considerably neglected (Muhlbauer & Muller, Citation2020; Onwude et al., Citation2016). The model was evaluated in terms of correlation coefficient R2 and root mean square error (RMSE).
whereis the constant of drying (1/min), t is the observation time (minute), and
is the moisture ratio, which can be calculated from Equationequation 2
(2)
(2) .
where and
are the MCs (kg water/kg dry material) at the initial time (
) and observation time (t), respectively, and
is the equilibrium MC (kg water/kg dry material), which is a function of air temperature and relative humidity (Sasongko et al., Citation2020) (temperature range, 30–50°C; relative humidity (RH) range, 0–90%), as expressed by the modified Henderson model (Viswanathan et al., Citation2003) in Equationequation 3
(3)
(3) .
where is 100% of the equilibrium MC (dry basis), and
is the air temperature (°C).
The drying time of onion slices from an MC of 86.02% (wet basis) or 6.15 kg water/kg dry solid (dry basis) to an MC of 30% (wet basis) or 0.42 kg water/kg dry solid (dry basis) can be estimated using Equationequations 1(1)
(1) –Equation3
(3)
(3) with the Newton model.
2.2.2. Thermal efficiency
Thermal efficiency was estimated on the basis of the laws of conservation of mass and energy. The following assumptions were taken into account for the calculations.
The heater, dryer, and pipe system were operated adiabatically.
No heat loss to the environment occurred during drying.
The hot air was homogenously distributed in the dryer ()
The process was well mixed system
The physical properties (e.g., density and specific heat) of dry and wet air were identical.
The dimension of onion did not change during the drying or the onion shrinkage was neglected ()
Pressure drops were not considered in the calculations.
The operating pressure was 1 bar.
The exhaust air of the dryer had a relative humidity of 50%.
The enthalpy was referenced to a temperature of 0°C.
EquationEquation 4(4)
(4) shows the expression used to calculate the thermal efficiency of the dryer (Kudra, Citation2012):
where is the temperature of ambient air (°C),
is the temperature of the exhaust air leaving the dryer (°C), and
is the temperature of air entering the dryer (°C).
The temperature of exhaust air can be estimated from the energy balance of the heat generated by the dryer and the heat of water evaporation, as shown in Equationequations 5(5)
(5) and Equation6
(6)
(6) .
The energy balance in the adiabatic drying system, where the heat used to evaporate moisture from the onion equals the sensible heat released by the hot air, could be used to estimate the temperature change of air () as follows:
where is the mass of dry solid (kg),
is the latent heat of water vaporization (kJ/kg) (Green & Southard, Citation2019),
is the air flow rate (kg/minute), and
is the heat capacity of air (kJ/kg K) (Green & Southard, Citation2019). The thermal efficiency calculations were conducted at different drying capacities (i.e., 0.05, 0.075, 0.1, 0.125, and 0.15 kg of onion slices) and drying times. The average thermal efficiency was calculated from the two sets of experiments and reported as the result.
2.2.3. Recycled exhaust air
The exhaust air leaving the drying chamber contains a large amount of heat that could be potentially reused. In the present study, some of the exhaust air exiting the dryer was recycled and mixed with fresh ambient air before feeding to the dryer. In this way, the total heat load in the heater could be reduced, resulting in improved thermal efficiency. Partial, rather than total, recycling of the exhaust air is recommended because total recycling can lead to saturation of the drying air. In this case, the capacity of air to evaporate water from a wet product is limited. The thermal efficiency calculations were slightly modified to consider partial air recycling, as shown in Equationequation 7(7)
(7) .
where is the temperature of mixed air (fresh air + recycled air), which can be estimated by Equationequation 8
(8)
(8) .
where and
respectively refer to the flowrates of fresh ambient air (m3/minutes) and recycled air (m3/minutes).
Because the total air entering the dryer is considered constant, addition of recycled air could reduce the amount of fresh air entering the dryer. EquationEquation 8(8)
(8) was rearranged to obtain Equationequations 9
(9)
(9) and Equation10
(10)
(10) :
where represents the recycled exhaust air to fresh air ratio. Here the
values were varied from 1 to 4 to determine the effect of recycled exhaust air on thermal efficiency enhancement.
2.2.4. Optimization using response surface methodology (RSM)
Response surface methodology was used to explore correlations between independent and dependent variables in the drying process. Here, sequential values of the independent variables were applied to determine the optimal response of the dependent variable. A central composite design (CCD) was used in the optimization studies. The design consisted of 10 runs, including 4 star, 4 cube, and 2 center points. The drying temperature and drying capacity
were selected as independent variables, and the percentage of thermal efficiency (E) was selected as the response. The correlation of the factors and responses was expressed as a second-order polynomial equation (Equationequation 11
(11)
(11) ) (Sasongko et al., Citation2020)
where is the predicted response,
is the constant coefficient,
and
are linear coefficients,
are quadratic coefficients, and
is a two-factor interaction coefficient. Statistical analyses and optimization were performed using Statistica software (version 10.0, USA).
3. Results and discussion
3.1. Parameter estimation
presents the moisture ratios of dried onion slices without recycled exhaust air and recycled exhaust air to fresh air ratio of 1 at drying temperatures of 50°C, 60°C, and 70°C. In all drying experiments, the moisture ratio decreased exponentially, which is a typical observation in food products during drying. The decrease in moisture ratio was more pronounced in the first 60 minutes of drying than at later times because of the high initial concentration of free moisture on the surface of the samples. Thus, moisture could easily be diffused to and evaporated by the drying air. Approximately midway through the drying process, the availability of free moisture on the surface of the samples is limited, and the removal of bound moisture within the inner tissue matrix of the onion begins to take place (Asiah et al., Citation2017). This process requires more heat to break moisture–onion layer linkages and a longer time for moisture to diffuse to the onion surface before evaporating, as observed in some other food products during drying (Kudra, Citation2012; Viswanathan et al., Citation2003).
Figure 4. Experimental and modeled moisture ratios as a function of drying time at a drying capacity of 0.1 kg of onion slices (a) without recycled exhaust air, R = 0 and (b) recycled exhaust air to fresh air ratio of 1
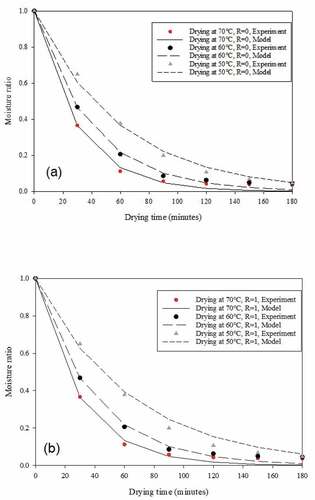
also demonstrates that the rate of moisture ratio reduction is faster at higher drying temperatures than at lower ones. For example, the moisture ratio observed after drying (180 minutes) at a drying temperature of 70°C is 1.21 times lower than that obtained when the drying temperature is 60°C. Higher drying temperatures promote the driving force for mass transfer, which increases the moisture evaporation rate and reduces the drying time (Djaeni et al., Citation2011).
The different change of onion slice volume during the drying process was observed by measuring the thickness and diameter of onion slice, as previously presented in . The difference of thickness was about 13% for total moisture content 86.02% and 30.76%. So that, the shrinkage factor can be neglected. Hence, the Newton Model can be suitable for this case (Muhlbauer & Muller, Citation2020; Onwude et al., Citation2016).
The Thus, the moisture ratio profiles could be fitted using the Newton model, which has been employed to describe the drying processes of food such as strawberry (El-Beltagy et al., Citation2007), red chili (Hossain & Bala, Citation2007), and onion (Kadam et al., Citation2011). According to the R2 and RMSE values calculated in the present study, the Newton model fits the data well (). The R2 values were very close to 1.00, and the RMSE values were lower than 0.008. These findings imply that the Newton model can be used to describe the phenomenon of onion slice drying. The validated model was applied to estimate the drying time from an initial MC of 86.02% (wet basis) or 6.15 kg water/kg dry solid (dry basis) to a final water content of 30% (wet basis) or 0.429 kg water/kg dry solid (dry basis), as shown in .
Table 1. Parameter estimations of the Newton model for onion slice drying
As the drying temperature increased, the drying rate increased and the drying time decreased (). Higher drying temperatures enhance the driving force for mass transfer by increasing the temperature difference, which leads to lower moisture viscosity and higher moisture diffusivity. These phenomena result in faster mass transfer rates from the moist product to the drying air (Djaeni et al., Citation2011). According to the results of onion slice drying, increasing the drying temperature by 20°C would reduce the total drying time by nearly half. The drying process with and without exhaust air recycle were comparable. The exhaust air recycle did not affect the drying rate and drying time, since the temperature of hot air entering the dryer is same. However, the way can reduce the heat load of the preheater.
3.2. Thermal efficiency estimation
exhibits the thermal efficiencies estimated for various drying times under different temperatures and drying capacities. Earlier experiments indicated that higher temperatures improve thermal efficiency by providing stronger driving forces for mass transfer; thus, more moisture could be evaporated from the onion. However, midway through drying, the residual moisture on the onion slice surface decreases and the driving force for mass transfer declines. Thus, more heat is required to break the bound moisture within the tissue matrix of the onion layer (Minea, Citation2016), and poorer thermal efficiency is observed.
Figure 5. Thermal efficiency as a function of drying time under different drying temperatures and drying capacities without recycled exhaust air (R = 0)
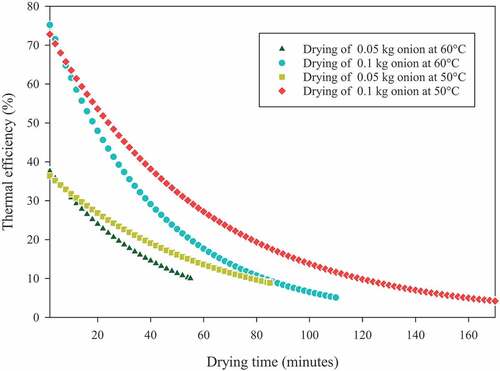
An increase in drying capacity enhances thermal efficiency, as shown in . The amount of moisture increases as the drying capacity increases. The sensible heat transferred from the drying medium and could be fully utilized in the presence of more free moisture that could be easily evaporated. However, longer drying times eventually reduce the average thermal efficiency of the drying process. In all cases studied, drying without recycled exhaust air resulted in poor thermal efficiency.
This low efficiency can be explained by analyzing the temperature of the exhaust air exiting the dryer. In all drying experiments, the temperature of the exhaust air leaving the drying chamber was higher than that of ambient air. After 60 min of drying, for example, the temperature of the exhaust air leaving the dryer remained close to the inlet air temperature (see ). Thus, heat usage for water evaporation in the system without recycled exhaust air may be considered inefficient.
Figure 6. Temperatures of exhaust air at a drying capacity of 0.1 kg of onion slices under different inlet air temperatures without recycled exhaust air (R = 0)
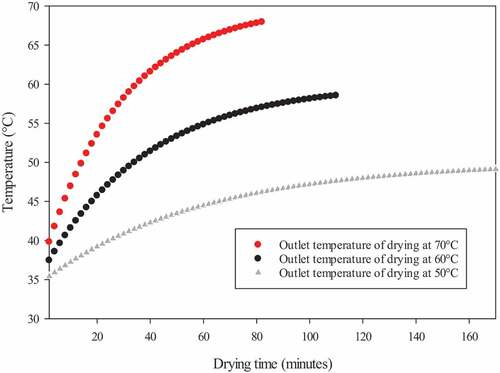
Because exhaust air contains a considerable amount of sensible heat, some of the exhaust air leaving the drying chamber could be recirculated and mixed with fresh ambient air prior to feeding into the heater. In this way, heat is directly recovered, the air temperature entering the heater increases significantly, and the heat load of the preheater could be reduced.
The average thermal efficiency of onion slice drying from an initial MC of 86.02% (wet basis) or 6.15 kg water/kg dry solid (dry basis) to a final MC of 30% (wet basis) or 0.429 kg water/kg dry solid (dry basis) was estimated. The results in showed that thermal efficiency improves with increasing air temperature and drying capacity (Borah et al., Citation2015; Djaeni et al., Citation2011). For example, increasing the air temperature by 10°C could increase the thermal efficiency of drying by 10%. The thermal efficiency of drying improved with increasing drying capacity. However, at a capacity of 0.12 kg, the improvement in thermal efficiency was not significant because the ability of the air to evaporate moisture from the samples is limited. In general, onion drying without recycled exhaust air resulted in poor average thermal efficiencies. This finding supports the results of previous studies on apple slice drying (Beigi, Citation2016), greenhouse drying (Kadam et al., Citation2011), and onion drying with a convective dryer (EL-Mesery & Mwithiga, Citation2012).
3.3. Thermal efficiency improvement with recycled exhaust air
The moderately hot exhaust air exiting the dryer contains potential sensible heat that could be reused for other purposes. Unfortunately, exhaust air is also characterized by a higher absolute humidity, which could reduce the driving force for mass transfer. Therefore, total exhaust air recycling is not recommended. Instead, some of the exhaust air could be recycled to recover heat directly and reduce the preheater load required to heat the air to the desired drying temperature (Djaeni et al., Citation2007; Krokida & Bisharat, Citation2004). shows that the thermal efficiency for drying in all test cases could be significantly enhanced by employing recycled exhaust air. A recycled exhaust air to fresh air ratio of 1 (50% fresh air + 50% exhaust air) improved the thermal efficiency of drying by 1.5 times compared the drying process without exhaust air recycling (R = 0), especially in the first 30 min. However, after certain time (suppose 80 minutes), the thermal efficiency improvement was limited because the water content in product was low, which inhibits the driving force for mass transfer.
Figure 8. Effect of recycled exhaust air-to-fresh air ratio on the thermal efficiency of onion slice drying at different drying capacities and a drying temperature of 70°C
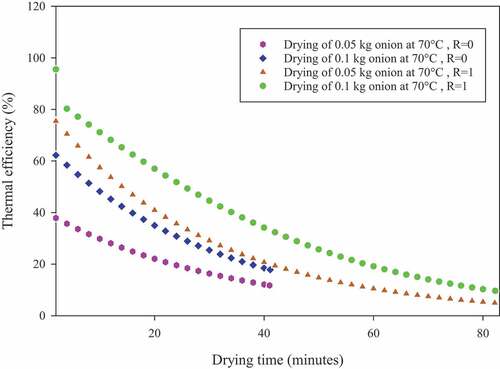
The average thermal efficiency of the system represents the performance of onion slice drying with recycled exhaust air. The average thermal efficiency was calculated on the basis of the heat required to reduce the MC of onion from 86.02to 30% (wet basis). shows the thermal efficiency of onion slice drying at a drying temperature of 70°C under various recycled exhaust air-to-fresh air ratios and drying capacities. In all cases, thermal efficiency improved with increasing drying capacity and recycled exhaust air-to-fresh air ratio. For example, without recycled exhaust air, the maximum thermal efficiency calculated was 39%, only, as depicted in . By exhaust air recycle, the average thermal efficiency was able to reach approximately 57% (). Improvements in thermal efficiency by incorporating recycled exhaust air could help remarkably reduce the overall energy requirement for drying, similar to the findings of a previous study (Djaeni et al., Citation2007).
3.4. Optimization of thermal efficiency in onion slice drying
The objective of the present experiment is to reduce the MC of onion slices from 86% to the 30% (wet basis) with optimum thermal efficiency. Ten experimental runs were conducted to obtain the optimal drying conditions that could maximize the thermal efficiency of drying. Low and high levels of independent variables were selected on the basis of the data presented in . The factors selected for optimization included drying temperature (60–70°C) and drying capacity (0.025–0.125 kg of onion slices), and a recycled exhaust air-to-fresh air ratio of 4.0 was applied. shows the low and high levels of independent variables, and shows the experimental design using orthogonal CCD.
Table 2. Process variables and levels of the orthogonal central composite design
Table 3. Experimental variables used for optimization via the orthogonal central composite design and observed responses of thermal efficiency at a recycled exhaust air-to-fresh air ratio of 4.0
Optimization using orthogonal CCD revealed the relationship between two independent variables (i.e., drying temperature and onion slice weight) and one response variable (i.e., thermal efficiency) as expressed in a second-order polynomial equation (Equationequation 12(12)
(12) ). This equation was evaluated using R2, as shown in . The model was successfully fitted to the data with R2 close to 0.9.
Table 4. Results of the quadratic model of the orthogonal central composite design
The coefficients of and
in equation 13 were positive, which indicates that thermal efficiency increases at higher drying temperatures and drying capacities. Three- and two-dimensional plots of the thermal efficiency of onion slice drying determined from equation 13 and are illustrated in , respectively. According to ,
significantly affects the thermal efficiency of drying with a p value of <0.05. The maximum thermal efficiency, at 58.82%, was obtained at a drying temperature of 67.94°C and drying capacity of 0.089 kg of onion slices. Therefore, improving thermal efficiency by heat recovery may be a sensible option to reduce the total energy consumption of a drying system.
3.5. Comparison with other drying methods
The thermal efficiency of several drying technologies for fruits and vegetables have recently been evaluated. Kadam et al. (Citation2011) observed the thermal efficiency of a greenhouse solar dryer for onion slice drying at midday for 10 days and obtained a thermal efficiency of approximately 20.82%. A hybrid dryer combining two heat sources, namely, solar energy and microwave energy, revealed a thermal efficiency of approximately 24.3% (Çelen & Karataser, Citation2019). A solar collector equipped with a modified solar tracking system to move the collector showed enhanced thermal efficiency of up to 75.7% (Das & Akpinar, Citation2020).
The thermal efficiency of convective dryers used for apple slice and potato drying is generally below 15% (El-Beltagy et al., Citation2007; Kadam et al., Citation2011). Such poor efficiency can be explained from the perspective of the temperature of the exhaust air leaving the dryer. In the present study, some of the exhaust air was recycled by mixing with fresh air entering the heater so that the sensible heat of the exhaust air could be directly recovered and reduce the heater load. A recycled exhaust air-to-fresh air ratio of 4.0 resulted in a thermal efficiency of 58.82% at a drying temperature of 67.94°C. This improvement is superior to the thermal efficiencies produced by a solar dryer (Kadam et al., Citation2011), hybrid dryer (Çelen & Karataser, Citation2019), convective dryer (Beigi, Citation2016), and vacuum infrared dryer (Hafezi et al., Citation2015).
4. Conclusion
The thermal efficiency of onion slice drying was studied at various temperatures and drying capacities, and the drying rate and effective drying time of 0.025 kg of onion slices at temperatures ranging from 50°C to 70°C were evaluated using the Newton model. The Newton model could satisfactorily predict the drying characteristics of onion slices. Drying rates increased with increasing drying temperature, and the drying time could be shortened by approximately 50% by increasing the drying temperature by 20°C. The combination of mass and heat balances could help predict the temperature of the air exiting the dryer and be used in thermal efficiency calculations. Results showed that the average thermal efficiency of the conventional dryer is relatively poor. However, introduction of recycled exhaust air could reduce the heat load of the heater and significantly improve the average thermal efficiency of the dryer. Drying efficiencies improved with increasing drying temperature, recycled exhaust air-to-fresh air ratio, and drying capacity.
Finally, process optimization via CCD was conducted to obtain the best conditions with which to dry onion slices from an initial MC of 86% to a final MC of 30% (wet basis) with optimum thermal efficiency. Three input variables, namely, drying temperature, drying capacity, and recycled exhaust air-to-fresh air ratio, were selected. Results showed that the maximum thermal efficiency (58.82%) could be achieved at a drying temperature of 67.94°C, drying capacity of 0.089 kg onion slices, and recycled exhaust air-to-fresh air ratio of 4.0. These results demonstrate that incorporation of recycled exhaust air could remarkably reduce energy costs during onion slice drying.
Declaration of conflict of interests
The authors declare no conflict of interest related to the work presented in this paper.
Additional information
Funding
Notes on contributors

Mohamad Djaeni
Mohamad Djaeni is a professor at Department of Chemical Engineering, Faculty of Engineering, Diponegoro University. His research interest is development of drying technology for food product.
Hadiyanto Hadiyanto
H. Hadiyanto is a professor with research interest on renewable energy conversion at Department of Chemical Engineering Diponegoro University.
Andri Cahyo Kumoro
Andri Cahyo Kumoro is a professor at Department of Chemical Engineering Diponegoro University with the subject of food science and technology.
Febiani Dwi Utari
Febiani Dwi Utari is a doctoral student at Department of Chemical Engineering Diponegoro University with the subject of drying and food processing.
Ching-Lik Hii
Ching-Lik Hii is a senior lecturer on Department of Chemical and Environmental Engineering, Faculty of Science and Engineering, University of Nottingham Malaysia with the expertise in drying & processing of food, vegetables and fruits.
References
- Asiah, N., Djaeni, M., & Hii, C. L. (2017). Moisture transport mechanism and drying kinetic of fresh harvested red onion bulbs under dehumidified air. International Journal of Food Engineering, 13(9). https://doi.org/10.1515/ijfe-2016-0401
- Atuonwu, J. C., Jin, X., van Straten, G., van Deventer Antonius, H. C., & van Boxtel, A. J. (2011). Reducing energy consumption in food drying: Opportunities in desiccant adsorption and other dehumidification strategies. Procedia Food Science, 1, 1799–17. https://doi.org/10.1016/j.profoo.2011.09.264
- Bamba, B. S. B., Komenan, A. C. A., Kouassi, K. K. P., & Soro, D. (2020). Effects of onion bulb processing conditions on drying characteristics, physicochemical and functional properties profile of onion (Allium cepaL.) powder. Journal of Food Science, 85(10), 3345–3354. https://doi.org/10.1111/1750-3841.15415
- Beigi, M. (2016). Energy efficiency and moisture diffusivity of apple slices during convective drying. Food Science and Technology, 36(1), 145–150. https://doi.org/10.1590/1678-457X.0068
- Borah, A., Hazarika, K., & Khayer, S. M. (2015). Drying kinetics of whole and sliced turmeric rhizomes (Curcuma longa L.) in a solar conduction dryer. Information Processing in Agriculture, 2(2), 85–92. https://doi.org/10.1016/j.inpa.2015.06.002
- Bourdoux, S., Li, D., Rajkovic, A., Devlieghere, F., & Uyttendaele, M. (2016). Performance of drying technologies to ensure microbial safety of dried fruits and vegetables. Comprehensive Reviews in Food Science and Food Safety, 15(6), 1056–1066. https://doi.org/10.1111/1541-4337.12224
- Çelen, S., & Karataser, M. A. (2019). Investigation of the performance of a hybrid dryer designed for the food industry. Foods, 8(2), 1–15. https://doi.org/10.3390/foods8020081
- Das, M., & Akpinar, E. K. (2020). Determination of thermal and drying performances of the solar air dryer with solar tracking system: Apple drying test. Case Studies in Thermal Engineering, 21(August), 100731. https://doi.org/10.1016/j.csite.2020.100731
- Djaeni, M., & Arifin, U. F. (2017). Kinetics of thiamine and color degradation in onion drying under various temperatures. Advanced Science Letters, 23(6), 5772–5774. https://doi.org/10.1166/asl.2017.8828
- Djaeni, M., Asiah, N., Wibowo, Y. P., & Yusron, D. A. A. (2016). Quality evaluation of onion bulbs during low temperature drying. American Institute of Physics, 060010. https://doi.org/10.1063/1.4949317
- Djaeni, M., Bartels, P., Sanders, J., van Straten, G., & van Boxtel, A. J. (2007). Process integration for food drying with Air Dehumidified by Zeolites. Drying Technology, 25(1), 225–239. https://doi.org/10.1080/07373930601161096
- Djaeni, M., Bartels, P. V., Sanders, J. P. M., & van Boxtel, A. J. (2011). Low temperature drying with Air Dehumidified by Zeolite for food products: Energy efficiency aspect analysis. International Journal of Food Engineering, 7(6). https://doi.org/10.2202/1556-3758.1930
- Edith, D. M. J., Dimitry, M. Y., Richard, N. M., Armand, A. B., Léopold, T. N., & Nicolas, N. Y. (2018). Effect of drying treatment on nutritional, functional and sensory properties of three varieties of onion powders. Journal of Food Measurement and Characterization, 12(4), 2905–2915. https://doi.org/10.1007/s11694-018-9906-1
- El-Beltagy, A., Gamea, G. R., & Essa, A. H. A. (2007). Solar drying characteristics of strawberry. Journal of Food Engineering, 78(2), 456–464. https://doi.org/10.1016/j.jfoodeng.2005.10.015
- EL-Mesery, H. S., & Mwithiga, G. (2012). Comparison of a gas fired hot-air dryer with an electrically heated hot-air dryer in terms of drying process, energy consumption and quality of dried onion slices. African Journal of Agricultural Reseearch, 7(31), 4440–4452. https://doi.org/10.5897/ajar12.614
- Green, D. W., & Southard, M. Z. (2019). Perry’s chemical engineers’ handbook. McGraw-Hill Education.
- Hafezi, N., Sheikhdavoodi, M. J., & Sajadiye, S. M. (2015). Study of energy consumption of potato slices during drying process. Acta Technologica Agriculturae, 18(2), 36–41. https://doi.org/10.1515/ata-2015-0008
- Hossain, M. A., & Bala, B. K. (2007). Thin-Layer drying characteristics for green chilli. Drying Technology, 20(2), 489–505. https://doi.org/10.1081/DRT-120002553
- Kadam, D. M., Nangare, D. D., Singh, R., & Kumar, S. (2011). Low-cost greenhouse technology for drying onion (Allium Cepa L.) Slices. Journal of Food Process Engineering, 34(1), 67–82. https://doi.org/10.1111/j.1745-4530.2008.00337.x
- Kemp, I. C. (2014).Fundamentals of energy analysis of dryers. In Modern drying technology (Vols. 4–4, pp. 1–45). John Wiley & Sons, Inc. https://doi.org/10.1002/9783527631728.ch21.
- Krokida, M. K., & Bisharat, G. I. (2004). Heat recovery from dryer exhaust air. Drying Technology, 22(7), 1661–1674. https://doi.org/10.1081/DRT-200025626
- Kudra, T. (2012). Energy performance of convective dryers. Drying Technology, 30(11–12), 1190–1198. https://doi.org/10.1080/07373937.2012.690803
- Metrani, R., Singh, J., Acharya, P., Jayaprakasha, G. K., & Patil, B. S. (2020). Comparative metabolomics profiling of polyphenols, nutrients and antioxidant activities of two red onion (Allium cepa L.) Cultivars. Plants, 9(1077), 1–18. https://doi.org/10.3390/plants9091077
- Minea, V. (2016). Advances in heat pump-assisted drying technology. CRC Press.
- Mota, C. L., Luciano, C., Dias, A., Barroca, M. J., & Guiné, R. P. F. (2010). Convective drying of onion: Kinetics and nutritional evaluation. Food and Bioproducts Processing, 88(2–3), 115–123. https://doi.org/10.1016/j.fbp.2009.09.004
- Muhlbauer, W., & Muller, J. (2020). Drying atlas: Drying kinetics and quality of agricultural products. Woodhead Publishing.
- Nwakuba, N. R., Chukwuezie, O. C., & Osuchukwu, L. C. (2017). Modeling of drying process and energy consumption of Onion (Ex -gidankwanoSpp.) Slices in a hybrid crop dryer. American Journal of Engineering Research(AJER), 6(1), 44–55.
- Onwude, D. I., Hashim, N., Janius, R. B., Nawi, N. M., & Abdan, K. (2016). Modeling the thin-layer drying of fruits and vegetables: A review. Comprehensive Reviews in Food Science and Food Safety, 15(3), 599–618. https://doi.org/10.1111/1541-4337.12196
- Sasongko, S. B., Hadiyanto, H., Djaeni, M., Perdanianti, A. M., & Utari, F. D. (2020). Effects of drying temperature and relative humidity on the quality of dried onion slice. Heliyon, 6(7), e04338. https://doi.org/10.1016/j.heliyon.2020.e04338
- Shreve, B., Thiex, N., & Wolf, M. (2006). NFTA reference methods. National Forage Testing Association.
- Strumilto, C., Jones, P. L., & Zylla, R. (2014). Energy aspects in drying. In Handbook of industrial drying, fourth edition (Fourth ed., Issue July 2014, pp. 1077–1100). CRC Press. https://doi.org/10.1201/b17208-59
- Tiwari, A. (2016). A review on solar drying of agricultural produce. Journal of Food Processing & Technology, 7(9), 1000623. https://doi.org/10.4172/2157-7110.1000623
- Viswanathan, R., Jayas, D. S., & Hulasare, R. B. (2003). Sorption isotherms of tomato slices and onion shreds. Biosystems Engineering, 86(4), 465–472. https://doi.org/10.1016/j.biosystemseng.2003.08.013