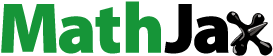
Abstract
This study seeks to analyze different energy solutions for the Kanalitojo Indigenous Community that is located 25 kilometers from the municipal seat of the municipality of Puerto Carreño, in the State of Vichada, eastern Colombia. For the development of this study, a technical performance analysis has been applied to each proposed solution considering the amount of electricity generation and demand. The first solution refers to a hydrokinetic turbine connected to a distribution network. The second solution combines the use of solar panels, hydrokinetic turbine, and portable batteries with which it is possible to transport the generated energy to the places where the community needs it. The third proposed energy solution refers to the use of micro-cogeneration units and portable batteries. With the use of the capabilities of the TRNSYS v17 energy simulation software, the comparative technical analysis between each solution for the same load demand is carried out. This analysis has shown a great advantage in the use of solar panels, hydrokinetic turbine, and portable batteries with an electricity surplus of 103.8%. However, technically the first solution based on a hydrokinetic turbine is more suitable for the community challenges due to it meets the electricity demand of the community by an excess of 12.4% and the capital and operating expenditure are less.
PUBLIC INTEREST STATEMENT
This study seeks to analyze different energy solutions for the Kanalitojo Indigenous Community located nearby Puerto Carreño (Vichada State), eastern Colombia. It is a vulnerable community that does not have access to electric power service through an interconnected network. For the development of this study, a technical performance analysis has been applied to each proposed solution considering the amount of electricity generation and demand. The proposed solutions are: 1. Hydrokinetic turbine. 2. Hydrokinetic turbine, solar panels, and portable batteries.3. Micro-cogeneration unit and portable batteries (MCHP).
1. Introduction
In Colombia, the non-interconnected zones (NIZ) refer to geographical areas that are not electrically coupled to national connected system (NCS), that is, they do not have access to electric power service through an interconnected network, but they may have access to distributed generation based on Diesel. The population density of NIZ is extremely low (3 inhab/km2), due to the dispersion of both municipalities and dwellings, factor that makes the logistics of service attention difficult, with high costs investment and operation units. This is due to poor mobilization and transport infrastructure which is aggravated by the political and socio-economic conditions of the regions. In general, the NIZ represent no less than 52% of the Colombian territory with a population of approximately 1,500,000 people. Furthermore, these areas are strategic for Colombia which offer great possibilities of economic growth for the country, by natural, tourist and cultural activities (see ).
Figure 1. NIZ areas in Colombia
The connection to electrical interconnected networks is one of the main problems for isolated indigenous communities (Zapata et al., Citation2005). The possibility of having access to electricity represents a path for their development and improvements in their quality of life, but achieving this objective requires an analysis of environmental conditions and potential resources to achieve greater coverage with quality generation and sustainable over time. The case study to evaluate the assessment framework is the indigenous community Kanalitojo which seeks to provide clean energy to all families within the community as well as to generate the necessary energy to support their production processes that will support development and itself will provide a source of income for that community. Similar studies have been carried out around the world, beginning with the identification of different renewable energy potentials of the area, then quantifying them and comparing them with each other considering the community energy demands (Searcy & Flynn, Citation2010). Colombia has numerous possibilities for the selection of renewable energies, based on solar energy, which has great potential in the north and center of the country. It also has great potential in wind energy in its northern part, more precisely in the Caribbean region (Ricardo & Venecia, 2011).
River based hydrokinetic energy sources can be found across the entire national territory as Colombia has a high density of rivers, giving it another great, renewable energy potential (Betancur, Citation2016). These conditions make Colombia a potential player in the development of renewable energies, allowing the evaluation of development capacities for the different regions (Franco et al., Citation2008), but it is necessary to exploit them and increase their implementation due to the country depends almost entirely on conventional generation making energy security more fragile (Florez et al., Citation2009). The use of electricity by communities in NIZ is intended, as mentioned before, to improve their quality of life and boost their industrial development, but this would also help to work in tourist and cultural areas that promote the desire for knowledge of the region thereby managing to cover more economic sectors and thus leading to the sustainability of the community (Jiménez, Citation2014). This analysis will help to identify and develop the best energy alternative for the Kanalitojo community, starting with its characteristics of daily life and the renewable potential resources available in their territory e.g. solar energy (monthly average solar radiation and ambient temperature are 5,32 kWh/m2/day and 30 °C respectively) and hydrokinetic energy (monthly average river velocity and depth are 0.5 m/s and 1 m depth respectively).
The indigenous community of Kanalitojo is located 25 km from the municipality of Puerto Carreño, in the State of Vichada in Colombia. This community includes the population belonging to the three indigenous clans as follows: Sáliva, Amorúa and Sikuani. They are in total 163 people grouped in 33 families located in a settlement approximately 573 hectares. This population is made up of 93 women and 70 men. The problem facing this community is the limited availability of technological alternatives for energy generation to support the daily life in this indigenous community.
The Kanalitojo indigenous community is a nomadic and semi-nomadic community that presents requirements for energy supply to moving residences or living quarters and its surroundings. The main causes of this difficulty are the nomadic and semi-nomadic condition of the community, as they move their living space depending on the productive period of the main foods that they sow and consume. This makes difficult to think about traditional alternatives for the generation or supply of energy. Also, displacement and land disputes that currently affect this indigenous community has prevented construction projects and/or infrastructure development, among them, the supply of energy.
2. Materials and methods
This section will analyze the different energy technologies that could be applied in the case study community based on the potential of available renewable energy in their territory.
One of the technologies are solar panels whose principle of operation is the photovoltaic effect which produces an electrical voltage when semiconductor materials meet the sun rays. The solar panels are composed of solar cells arranged together (). These elements, together with other transmission and energy containment devices, make up the solar energy solution.
Another possible solution can be micro-cogeneration units (MCHP), which are composed of plants that commonly work with natural gas and, on starting, instantly generate electricity and useful heat in the same process. The heat produced is used to meet the needs of the Air Conditioning System (ACS) and Domestic Hot Water (DHW), and electric power is used for common demands as can be seen in . Some of its characteristics or advantages are as follows:
• Contribution to the environment with the reduction of CO2 emissions of up to 70%.
• Local energy generation.
• Decrease in electricity costs.
• Independence of the conventional electricity network.
• It is easily coupled to the energy systems previously established in buildings.
• Its working capacity is generally high, which ensures a great coverage in the energy requirements.
Another of the proposed solutions involves the use of wind energy, which refers to the transformation of the wind to convert it into electrical energy. Its operating principle consists of an inverse motor, that is, an engine to which rotational movement is applied so that it delivers energy (refer to ). All this is achieved using generators that consist of the rotation between a rotor and a stator whose interaction leads to a magnetic flux that is converted into electricity.
The magnetic flux (Φ) that passes through the armature has the value of the product of the field strength (B), the surface of the turn (s) and the cosine of the angle formed by the plane that contains it and the direction of the magnetic field (cos φ).
Whenever there is a variation of the magnetic flux that crosses a loop, an induced electromotive force (EMF) is produced, represented by ε whose value varies according to the speed of variation of the flow, for both we will have that ´n´ the number of turns of the armature:
The electromotive force induced in a coil that rotates in a uniform magnetic field varies with time in a sinusoidal way and its maximum value depends on the number of turns, the field strength, the coil section, and the rotation speed. The expression of the induced electromotive force is given by:
Hydrokinetic turbines operate under the same principles as wind turbines and share a similar philosophy of design (Güney & Kaygusuz, Citation2010; Yuce & Muratoglu, Citation2015).
The first step in the design of hydrokinetic turbines is the rotor sizing. For this, the turbine output power (Ƥ) needs to be considered, which in turn is given in terms of the fluid density (ρ), fluid velocity (V, directly proportional to V3), cross sectional area (A), power coefficient (Cp) and the train’s transmission efficiency (ɳ), where Cpɳ must be equal to 21 % (Anyi & Kirke, Citation2011).
The variation in the flow velocity profile serves to determine the location with the highest efficiency in which the system should be installed. shows schematically the relation between flow speed and deepness. The flow speed is at its lowest value at the bottom of the river due to the high friction that experiences the flow against the surface of the riverbed. As the flow separates from the bottom, the speed increases to a maximum. Close to the surface, the friction exert by the air causes the speed to slow down again.
Figure 5. Schematic representation of the relation between deepness and flow speed variation (Gunawan et al., Citation2012)
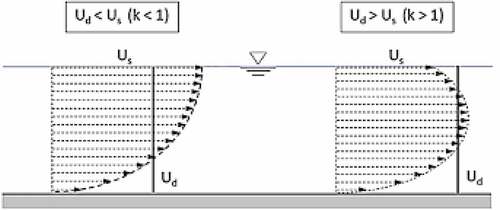
The flow velocity also varies breadthwise across the river and reaches a maximum near the center of the current (Gunawan et al., Citation2012). illustrates a typical flow speed profile and turbulence intensity in open canals. A variation in the input flow of the turbine can directly affect its efficiency. The turbulence may increase the hydraulic turbine’s efficiency in approximately 12%. To benefit from this additional energy the structural system needs to exploit the effect of the turbulence on its design (V. S. Neary et al., Citation2013).
Figure 6. Typical profiles of flow speed and turbulence intensity in an open canal for two different hydrokinetic turbines: a) vertical axis and b) axial flow (V. S. Neary et al., Citation2013)
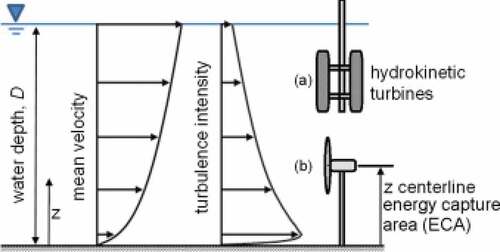
3. Experimental setup description and results
3.1. Description of the experimental setup
To carry out this analysis, a profile of energy consumption of the entire community was determined based on the geographical, social, and cultural conditions of the indigenous community of Kanalitojo. show the electrical demands by houses and community centers, respectively. These electrical demands were taken in 6-minute intervals leading to a total of 87,600 measures of electricity demand. For each 6minute demand registered, inductive loads are considered for the demands coming from appliances and general energy usage required.
Table 1 Electrical demand by the houses
Table 2 Electrical demand by the community centers
The next step was the definition of 3 possible solutions where different energy technologies were used: 1. Hydrokinetic turbine. 2. Hydrokinetic turbine, solar panels, and portable batteries.3. Micro-cogeneration unit and portable batteries.
The software used to simulate these 3 scenarios was TRNSYS v17, which is a simulation program primarily used in the fields of renewable energy engineering and building simulation for passive as well as active solar design.
To carry out the simulation of the energy system in TRNSYS v17, the Type that would be used operationally and its location or source within the software were defined. The entire system is governed by controllers that turn on and off each device according to the need of the system or solution. In each subsystem or set of associated Types in the simulation and the controllers were integrated with the subsequent subsystem. In this way, errors of handling or passage in flows of information from part of the system to another were avoided, which is a general rule for the determination of their functions through calculation controls offered by the software. The whole set was conditioned for use or control for one year making the software adjustments to avoid errors in each of the components and their inter-relationships with each other.
The first solution includes the use of a hydrokinetic turbine located in the Meta river with a distribution network for each of the 18 houses, the refrigeration, industrial and educational centers (see ).
The second proposed solution refers to the use of a single hydrokinetic turbine located in the Meta river and solar panels as a hybrid system, which will supply the necessary electricity for its use in the 18 houses via portable batteries, and centers mentioned previously ().
The third solution evaluated uses a micro-cogeneration unit linked to a bio-digester, which uses the organic waste generated from different activities within the community to produce biogas to feed the electric generation plant. This electricity produced will go to a reception plant, which in turn will send electricity to the refrigeration, educational and industrial centers, and it will also be the recharge point for the portable batteries for each family ().
Each of these solutions was simulated using the energy software TRNSYS V17, for an electricity generation period of one year based on the community’s total electricity demand.
The specifications of the solar photovoltaic module (SPM), hydrokinetic turbine (HKT), and micro-cogeneration unit (MCHP) used in this simulation are given as follows:
SPM: solar photovoltaic panels with a power of 8 kWp, distribution system (4.5 kW, 100 kg weight, hybrid), MOLL OPzV solar stationary batteries (20 kWh), and Yeti150 single portable batteries (3 kg weight, 250 Wh, for 33 families).
HKT: a Smart Hydro Power hydrokinetic turbine unit with a maximum power of 5 kW.
MCHP: a Senertec Dachs micro-CHP (microcombined heat and power) unit with a maximum power of 5.5 kW.
The validation process of the TRNSYS simulation model is presented in Colmenares-Quintero et al. (Citation2018) and Colmenares-Quintero et al. (Citation2021) available in open access. The mathematical modelling of the power output of each system is based on the following equations:
SPM: E = A * r * H * PR, where E (kWh)—Energy, A (m2)—Total Solar Panel Area, r (%)—Solar Panel Yield or Efficiency, H—Annual Average Solar Radiation on Tilted Panels (shadings not included) and PR—Performance Ratio, Coefficient for Losses (range between 0.5 and 0.9, default value = 0.75).
HKT: P = 0.5*Cp*ρ*A*V3*10−3 where P (kW)—Power, Cp—Coefficient of Turbine Performance, ρ (kg/m3)—Water Density, A (m2)—Blade-swept Area, V (m/s)—Water Velocity
MCHP: Power in kW = (Pm LAN/n 100)/60 in bar where Pm - mean effective pressure, L - length of the stroke in m A - area of the piston in m2, N - Rotational speed of engine RPM, n - number of revolutions required to complete one engine cycle: n = 1 (for two stroke engine), n = 2 (for four stroke engine).
3.2. Experimental results and scenarios development
For the simulation of solution 1, the system was assembled with a complete electricity demand on the hydrokinetic turbine (), and then was compared with the energy requirements ().
Table 3. Result simulation: comparison of demand and generation for Solution 1
In the simulation of solution 2, the assembly of the solar panels and the hydrokinetic turbine was carried out; both under the total electricity demand of the whole system (), and then the comparison with estimated total energy generated given in .
Table 4. Result simulation: comparison of demand and generation for Solution 2
The third solution was simulated with the total electricity demand of the system supported in the micro-cogeneration unit. This unit is defined as a modified internal combustion engine based on Diesel (refer to and ).
Table 5. Result simulation: comparison of demand and generation for solution 3
4. Conclusions
This study seeks to analyze different energy solutions for the Kanalitojo Indigenous Community located in the non-interconnected zone of Colombia. A simulation framework based on TRNSYS V17 was used. Data available from community and in the public domain was used to validate the model. As a result, the following conclusions were reached:
Understanding the lifestyle and the cultural behaviours of the communities, and the likely capabilities of alternative renewable energy resources available in their territory are of great importance in the selection of the most appropriate energy solution for indigenous communities.
3 scenarios were simulated: 1. Hydrokinetic turbine (HKT); 2. Hydrokinetic turbine, solar panels, and portable batteries (SPM and HKT); 3. Micro-cogeneration unit and portable batteries (MCHP).
The first (HKT) and second (SPM and HKT) technical solutions produce a power surplus of 12.4% and 103.8%, respectively, while the third solution (MCHP) produces less power than required by 19.1%.
Technically the first solution based on a hydrokinetic turbine is more suitable for the community challenges due to its ability to exceed the electricity demand of the community by 12.4%.
5. Recommendations
As a future work, the paper can be extended with the application of advanced intelligent algorithms with optimization methods to be coupled to the computational framework. Also, the capital expenditures (CAPEX) and operating expenses (OPEX) need to be considered so that better decisions can be made.
Acknowledgements
This project is funded through Engineering X, an international collaboration founded by the Royal Academy of Engineering and Lloyd´s Register Foundation in the United Kingdom. This award is part of the Engineering X “Engineering Skills Where They are Most Needed Mission”, which seeks to support the delivery of skills and education programmes that will lead to the development of much needed engineering skills capacity, enhanced safety standards, and infrastructure that remains safe and fit for purpose. Project reference # ESMN1921\1\177 “SDGs-challenges based learning in engineering curricula at the Universidad Cooperativa de Colombia: Enhancing the engineering skills in a developing country” and the UCC ID is INV2850. Also, the authors would like to thank the Institute for Planning and Promotion of Energy Solutions for Non-Interconnected Zones (IPSE) of the Colombian Ministry of Mines and Energy for fruitful discussions and provision of data in non-interconnected zones.
Additional information
Funding
Notes on contributors
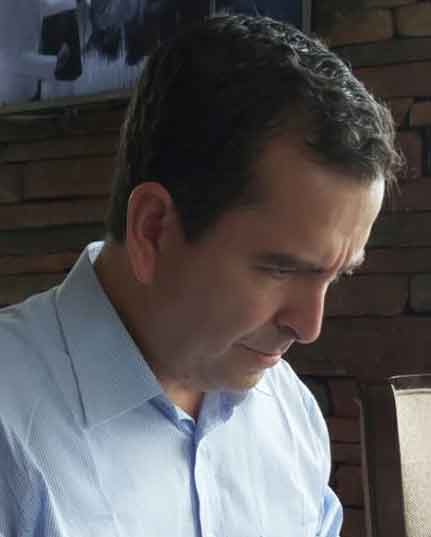
Ramón Fernando Colmenares-Quintero
Ramón Fernando Colmenares-Quintero is the currently national head of research in engineering and Prof.Dr. at UCC with research focus on energy simulation and modelling in the energy sector, and vulnerable communities.
Luis Fernando Latorre-Noguera is a researcher in energy system simulation at UCC. His research interests are in sustainable energy.
Natalia Rojas has a degree in Civil Engineering and an MSc in Marine Renewables, works for Aquatera bringing knowledge and expertise in energy engineering.
Prof.Dr. Karl Kolmsee founded Smart Hydro Power a company commercializing kinetic hydropower plants for rural electrification, and lectures energy management at Kufstein.
Kim E. Stansfield got a PhD in Composites from Kingston University. He was sustainable energy systems transformation planner at the UK ETI, and at Warwick WMG.
Prof.Dr. Juan Carlos Colmenares-Quintero is the leader of the research group CatSEE from the IPC/PAS in Poland. His interests range from materials science/nanotechnology to photocatalysis and water/air purification.
References
- Anyi, M., & Kirke, B. (2011). Hydrokinetic turbine blades: Design and local construction techniques for remote communities. Energy for Sustainable Development, 15(3), 223–13. https://doi.org/10.1016/j.esd.2011.06.003
- Betancur, J. A. (2016). Análisis de una solución energética para pequeñas comunidades aisladas, mediante la utilización de generación hidro-c)inetica. Pre Degree, Universidad Tecnológica de Pereira.
- Colmenares-Quintero, R. F., Góez-Sánchez, G. D., Colmenares-Quintero, J. C., Latorre-Noguera, L. F., & Kasperczyk, D. (2021). Application of a simulation tool based on a bio-inspired algorithm for optimisation of distributed power generation systems. Cogent Engineering, 8, 1. https://doi.org/10.1080/23311916.2021.1909791
- Colmenares-Quintero, R. F., Latorre-Noguera, L. F., Colmenares-Quintero, J. C., & Dibdiakova, J. (2018). Techno-environmental assessment of a micro-cogeneration system based on natural gas for residential application. CT&F - Ciencia, Tecnología Y Futuro, 8(1), 101–112. https://doi.org/10.29047/01225383.97
- Florez, J. H., Tobon, D., & Castillo, G. A. (2009). ¿Ha sido efectiva la promoción de soluciones energéticas en las zonas no interconectadas (ZNI) en Colombia?: Un análisis de la estructura institucional. Cuadernos de Administración, 22, 219–245. https://www.redalyc.org/pdf/205/20511730011.pdf
- Franco, C., Dyner, I., & Hoyos, S. (2008). A Contribution of the Energy at Development of Islated communities in not Interconnected Zones: A Case of application of the Systems Dynamics and Sustainable Livelihoods in the Colombian Southwest. Dyna, 75, 199–214. https://www.researchgate.net/publication/262520721_Contribution_of_the_energy_at_development_of_islated_communities_in_not_interconnected_zones_A_case_of_application_of_the_systems_dynamics_and_sustainable_livelihoods_in_the_Colombian_Southwest
- Gunawan, B., Sun, X., Sterling, M., Shiono, K., Tsubaki, R., Rameshwaran, P., Knight, D. W., Chandler, J. H., Tang, X., & Fujita, I. (2012). The Application of LS-PIV to a Small Irregular River for Inbank and Overbank Flows. Flow Measurement and Instrumentation, 24, 1–12. https://doi.org/10.1016/j.flowmeasinst.2012.02.001
- Güney, M. S., & Kaygusuz, K. (2010). Hydrokinetic Energy Conversion Systems: A Technology Status Review, Renewable and Sustainable Energy Reviews, Vol. 14(9), 2996–3004. https://doi.org/10.1016/j.rser.2010.06.016
- Jiménez, T. (2014). Alternative energy and community tourism: A joint commitment to sustainable human development in rural communities. Energética, 44, 93–105. https://repositorio.unal.edu.co/bitstream/handle/unal/52586/45487-236917-1-PB.pdf?sequence=1&isAllowed=y
- Neary, V. S., Gunawan, B., & Sale, D. C. (2013). Turbulent Inflow Characteristics for Hydrokinetic Energy Conversion in Rivers. Renewable and Sustainable Energy Reviews, 26, 437–445. https://doi.org/10.1016/j.rser.2013.05.033
- Priyadarshi, N., Anand, A., Sharma, A. K., Azam, F., Singh, V. K., & Sinha, R. K. (2017). An Experimental Implementation and Testing of GA based Maximum Power Point Tracking for PV System under Varying Ambient Conditions Using dSPACE DS 1104 Controller. Int. J. Renew. Energy Res, 7, 255–265. https://www.ijrer.org/ijrer/index.php/ijrer/article/viewFile/5379/pdf
- Priyadarshi, N., Kumar, V., Yadav, K., & Vardia, M. (2017). An Experimental Study on Zeta buck-boost converter for Application in PV system. In Handbook of Distributed Generation (pp. 393–406). Cham, Switzerland.
- Priyadarshi, N., Padmanaban, S., Bhaskar, M. S., Blaabjerg, F., & Sharma, A. A. (2018). Fuzzy SVPWM Based Inverter Control Realization of Grid Integrated PV-Wind System with FPSO MPPT Algorithm for a Grid-Connected PV/Wind Power Generation System: Hardware Implementation. IET Electr. Power Appl, 12(7), 962–971. https://doi.org/10.1049/iet-epa.2017.0804
- Priyadarshi, N., Padmanaban, S., Maroti, P. K., & Sharma, A. (2018). An Extensive Practical Investigation of FPSO-Based MPPT for Grid Integrated PV System Under Variable Operating Conditions with Anti-Islanding Protection. IEEE Syst. J., 13(2), 1–11. doi: 10.1109/JSYST.2018.2817584.
- Energies, 11(5), 1067. https://doi.org/10.3390/en11051067
- Priyadarshi, N., Sharma, A. K., & Azam, F. (2017). A Hybrid Firefly-Asymmetrical Fuzzy Logic Controller based MPPT for PV-Wind-Fuel Grid Integration. Int. J. Renew. Energy Res, 7, 1546–1560.
- Searcy, E., & Flynn, P. C. (2010). A criterion for selecting renewable energy processes. Biomass and Bioenergy, 34(5), 798–804. https://doi.org/10.1016/j.biombioe.2010.01.023
- Shastry, A., Suresh, K. V., & Vinayaka, K. U. (2015). Hybrid Wind-Solar Systems using Cuk-Sepic Fused Converter with Quasi-Z-Source Inverter. In Proceedings of the IEEE Power, Communication and Information Technology Conference (PCITC), Bhubaneswar, India, 15–17 October; pp. 856–861.
- Yuce, M. I., & Muratoglu, A. (2015). Hydrokinetic energy conversion systems: A technology status review. Renewable and Sustainable Energy Reviews, 43, 72–82. https://doi.org/10.1016/j.rser.2014.10.037
- Zapata, C. M., Singh, B., Zuluaga, M. M., & Dyner, I. (2005). Fuentes alternativas de generación de energía, incentivos y mandatos regulatorios: Una aproximación teórica al caso colombiano. Energética, 34, 55–63.