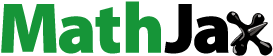
Abstract
The purpose of this study is to investigate the corrosion behavior of the ferrite-bainite phase of AISI1040 steel and its corrosion inhibition using pectin in 0.5 M sulphuric acid medium. The corrosion study was conducted through weight loss, potentiodynamic polarization, and electrochemical impedance spectroscopy (EIS) measurements. The study was conducted within the temperature range of 313–343 K and immersion time of 1 h— 7 h. The inhibition performance of pectin has boosted with an increase in the concentration of pectin and decreased with the temperature and time of exposure. From the weight loss study, the highest inhibition efficiency of 85% was achieved at 5.0 g/L at 1 h of exposure at 40 °C. The activation, thermodynamic, and adsorption isotherm were adapted for the experimental results. The energy and enthalpy of activation emphasized on energy barrier for the corrosion process. The entropy of activation values is negative, indicating the association of molecules. The physical adsorption of pectin on the metal surface was confirmed by the free energy of adsorption (close to-20kj mol−1). The enthalpy of adsorption indicated the exothermic process and, arrangement of the molecules on the surface of the metal expressed by the entropy of adsorption. The optimum efficiency of 74.9% is achieved for the addition of 5.0 g/L of pectin at 313 K by the EIS technique. Surface analyses involving a scanning electron microscope and atomic force microscopy were carried out to understand the nature of the surface in the presence and absence of pectin.
PUBLIC INTEREST STATEMENT
Corrosion is a phenomenon that produces a less desirable material due to the deterioration of the component/system in a corrosive medium. The purpose of the investigation was to control the corrosion of heat-treated applicable material in a corrosive medium (0.5 M sulphuric acid) using a green inhibitor (pectin). To understand the nature of inhibitor and optimization, the study was carried out at various conditions of inhibitor concentration, range of temperature, and time of exposure. The measurements were done using weight loss, potentiodynamic polarization, and electrochemical impedance spectroscopy. The surface analysis was carried out by scanning electron microscope and atomic force microscopy. From the output achieved from the experiment, the pectin inhibitor was effective at the lowest time of exposure and temperature considered in the experiment at the optimum concentration of inhibitor. Thus, pectin is an efficient inhibitor for the corrosion inhibition of the ferrite-bainite phase of AISI1040 steel.
1. Introduction
Corrosion inhibitors remain an important way of protecting metals and their alloys from corrosion processes in a corrosive environment (Nathan, Citation1973; T. Olugbade & Lu, Citation2019b; T. O. Olugbade, Citation2021; Shinato et al., Citation2020; Yu. M. Panchenkoa, A. I. Marshakova, L. A. Nikolaevaa, 2020). Carbon steel finds numerous applications in many industries due to its excellent mechanical properties, low cost, and easy workability (T. O. Olugbade, Citation2021). However, they exhibit poor corrosion resistance, which results in a metal loss. The use of corrosion inhibitors is a very effective and economic way of protecting metals in industrial processes, such as pickling, industrial acid cleaning, and oil and gas well acidizing (T. Olugbade et al., Citation2019; T. Olugbade & Lu, Citation2019a). The heat treatment methods are applied to increase the mechanical properties thereby increasing the applicability of the metal. Different materials like steel, aluminum, titanium, etc. undergo corrosion when it comes in contact with corrosive environments (Ali, Citation2019; Deepa & Padmalatha, Citation2017). AISI1040 steel (also known as EN 8 steel) is a plain medium carbon steel with moderate strength. The material properties can be reinforced by the thermal treatment process, where the metal is heated and then cooled at the required temperature, to obtain the desired microstructure. This steel can be utilized in the manufacturing of automobile parts. Dual-phase (DP) steels have received massive consideration in the past few decades because of increased application in the automotive industry (Abedini et al., Citation2019; Salamci & S. Candan, Citation2017). The dual-phase structure of steels has a place with a gathering of present-day multi-phase steels described by ferrite matrix containing different phases (martensite, bainite, and leftover austenite) (Abedini et al., Citation2019; Gerengi et al., Citation2020; Keleştemur et al., Citation2009; Song et al., Citation2019). The concurrence of delicate/hard phases and their connection in working conditions permit both high strength and malleability to be accomplished. DP steels comprising of delicate ferrite and hard martensite show better mechanical properties compared to regular HSLA (High Strength Low Alloy) steels (Abedini et al., Citation2019; song et al., Citation2020). That has driven the experts to investigate their appropriateness for various structural and constructional purposes (Katiyar et al., Citation2019). Although a lot of research articles have come out concerning the excellent blend of mechanical properties in DP steels (A. Karimi, S. Kheirandish, Citation2017; Basantia et al., Citation2021; Bilal et al., Citation2019; Fereiduni & Ghasemi Banadkouki, Citation2014; Kumar et al., Citation2019; Shahzad et al., Citation2018; Xiong et al., Citation2019; Zhao et al., Citation2019; Zhu, Citation2019), further examinations are important to discover the influence of phase constituents on the corrosion resistance properties (Bignozzi et al., Citation2020; Gürkan Aydın, Citation2019; Ma et al., Citation2020; P. R. Prabhu, Prabhu, Sharma et al., Citation2020; Zhang et al., Citation2020).
The organic molecules containing heteroatoms such as sulfur, oxygen, nitrogen, etc. have an inhibitory effect that is mostly attributed to their adsorption on the metallic surface (Galo et al., Citation2020; Mahgoub et al., Citation2010; Negm et al., Citation2011; Şahin et al., Citation2020; Tabatabaei majd et al., Citation2020). Nowadays, the focus of corrosion inhibition research is on biodegradable, environmental-friendly green inhibitors.
Pectin molecules satisfy the above conditions and they contain a large amount of α(1,4)-glycosidic-bound poly(D-galacturonic acid) (Charitha & Rao, Citation2020). The advantages of pectin are non-toxic, low cost for production, and soluble in acids. As a part of our research work with green and sustainable inhibitors (Deepa Deepa Prabhu & Rao, Citation2019; P. R. Prabhu, Prabhu, Rao et al., Citation2020; Salman et al., Citation2020), in this study, the applicability of pectin as a biopolymer to corrosion control of AISI1040 dual-phase steel with ferrite-bainite (F-B) structure in 0.5 M sulphuric acid was done. Furthermore, the temperature is generally reported to be one of the important factors that can influence metal corrosion and metal-inhibitor interaction (Bentiss et al., Citation2005; Mert et al., Citation2011). Numerous studies have examined the effect of steel corrosion inhibitors in aggressive environments where green inhibitors are present (Loto & Loto, Citation2020; Lowmunkhong et al., Citation2010; Obi-Egbedi et al., Citation2012; D; D Prabhu & Rao, Citation2013; Qiang et al., Citation2018; Solomon et al., Citation2010). The corrosion study of heat-treated applicable material using green inhibitors are limited. Therefore, the present study was done on the heat-treated AISI1040 steel to arrive at ferrite bainite phase followed by its corrosion behavior in 0.5 M sulphuric acid using weight loss, potentiodynamic polarization, and electrochemical impedance spectroscopy measurements. The corrosion inhibition studies were performed using pectin biopolymer varying time, temperatures, and inhibitor concentrations. The parameters of activation and thermodynamics of the material in the absence and presence of pectin have been calculated and discussed. The confirmation of the adsorption of pectin on the metal surface was carried out using a scanning electron microscope and atomic force microscopy.
2. Methodology
2.1. Material, medium, & inhibitor
2.1.1. Material & specimen preparation
The AISI1040 steel is an unalloyed medium carbon, moderate strength steel usually used in normalized condition. The chemical composition of AISI1040 steel used in the study are elements of iron (98.7 %), manganese (0.54 %) carbon (0.247 %), silicon (0.199 %), chromium (0.095 %), copper (0.059 %), nickel (0.044 %), sulphur (0.037 %), phosphorus (0.027), and molybdenum (0.027 %).
The heat-treated test samples were prepared in a cylindrical rod shape of 19 mm diameter and 8 mm height. The specimen was abraded using different grades of emery paper (80–1200) and finally on the polishing disk with different grades (3 microns to 0.25 micron) of diamond paste. The polished sample was then washed with double distilled water, cleaned with acetone, and eventually dried before being immersed in the acidic environment.
2.1.2. Preparation of medium
The medium used in the investigation is standard sulphuric acid. A large quantity, solution of sulphuric acid was made ready by diluting an analytical reagent grade sulphuric acid to an appropriate volume. The solution was standardized by the volumetric method. The solution of concentration (0.5 M) was made and prepared from the stock solution. The experiments were conducted at temperatures ranging from 313 K, 323 K, 333 K to 343 K.
2.1.3. Inhibitor used
The inhibitor used in our experiments is commercially available pectin. It is a structural acid heteropolysaccharide that is found in the medium and primary lamellar cell walls of terrestrial plants. The main constituent is galacturonic acid, which is a sweet acid (a derivative of galactose).
2.2. Heat treatment
The samples were heated in a muffle furnace to the predetermined austenitization temperature (900 °C). The process PQ () represents the austenitization process. The samples were held at the austenitization temperature for a fixed duration of 2 h. After isothermal holding for 2 h, specimens are quickly taken out from the furnace for air cooling to get the room temperature structure.
The normalized specimens are used for the ferrite-bainite heat treatment. Here, the austenitizing is carried out at 750°C for 2 h followed by quenching in a salt bath containing an equal proportion of molten sodium nitrate and sodium nitrite mixture held at a specific temperature of 350°C isothermally. After isothermal holding for about 30 min, specimens are cooled to room temperature in still air. shows the dual-phase heat treatment procedure to obtain an F-B structure.
shows the microstructure of AISI1040 steel in as-received condition. The steel shows ferrite and lamellar pearlite grains. The steel rods might have undergone air cooling, resulting in the formation of fine grains. shows the microstructure of steel in the normalized condition. The microstructure displays fine and lamellar pearlite and proeutectoid ferrite. The interlamellar distance in normalized pearlite is smaller when compared to the as-bought steel as a result of normalizing heat treatment. Fine pearlite enhances the mechanical properties of the steel. shows the duplex structure in the steel containing ferrite and feathery bainite. The steel is heated in between lower and upper critical temperatures of the iron carbide phase diagram where homogeneous austenite is not formed. During this process, the pearlite of as-received steel converts into austenite first and proeutectoid ferrite remains unchanged. When the steel is quenched from this temperature, the austenite converts to lower temperature structures like bainite. When the steel is quenched in subcritical temperatures (350°C), the cooling curve enters the bainite zone. When austenite is cooled in this temperature range, carbon atoms redistribute in austenite. Low carbon regions transform to ferrite by diffusionless processes and result in fine needles of ferrite. As time passes, carbon diffuses out and precipitates in the form of fine carbides, the arrangement of carbides here is not in the form of lamellar structure. In this study, the steel displays upper or feathery bainite along with proeutectoid ferrite.
2.3. Electrochemical impedance spectroscopy (EIS) method
For the studies of EIS and Potentiodynamic Polarization (PDP), the working electrode was made of DP AISI1040 steel (the molded specimen has been exposed to corrosive media with 0.7 cm2 surface area), a platinum electrode as a counter electrode, and a calomel electrode as a reference electrode. CH600E potentiostat was the workstation used for obtaining the EIS and PDP plots. The electrochemical experiments were carried out at 313 ± 1 K. All electrochemical measurements were performed in an aerated solution in unstirred conditions. The test samples were able to attain stable and consistent open-circuit potential (OCP) in 30 min. EIS measurements were done over a frequency range of 100 kHz to 10 mHz; small amplitude (10 mV) sinusoidal ac voltage was used. Experiments are repeated three times and the average of the best three agreeing values was reported.
The polarization resistance (Rp) values were derived from the EIS by adding the charge transfer resistance (Rct) and resistance of the solution (Rs). The inhibition efficiency (IEEIS %) of the inhibitor was derived from Rp values using the following Equationequation 1(1)
(1) :
where and
are the polarization resistance in the presence and absence of pectin, respectively.
2.4. Potentiodynamic polarization technique
The samples were polarized relative to the OCP with a potential of −250 mV cathodic and +250 mV anodically at a scan level of 1.0 mVs−1. The anode and cathodic curves were extrapolated to obtain the corrosion potential (Ecorr) as well as corrosion current densities (icorr). The inhibition efficiency (IEPDP %) was assessed using icorr values using Equationequation 2(2)
(2) :
where and
are the corrosion current densities in the absence and the presence of pectin, respectively.
2.5. Weight-loss method
Weight-loss corrosion measurements are very simple because they do not require specialized instruments apart from an accurate balance. Experiments are generally performed as per the standard method (A. G1-90, 1999). Weight-loss methods are predominantly slower than other techniques, but a good number of samples can be run at the same time. This method obtains the average corrosion rate over extended periods, according to the following Equationequation 3(3)
(3) :
where K = a constant (3.45 x 106), W = difference in weight of the sample in gms, D = density of the sample in g/cm3, A = sample surface area exposed to a corrosive medium in cm2, t = exposed time in hours.
From the weight-loss method, the inhibition efficiency (IEw) is calculated by using the following Equationequation 4(4)
(4) :
where W1 = the difference between the initial and final sample weights in a corrosive environment without an inhibitor (gms), W2 = the difference between the initial and final weight of the sample in the presence of inhibitor (gms)
2.6. Effect of temperature and kinetic factors
The CRs measured at various temperatures (313–343 K) by the weight loss method were applied for the calculation of activation energy Ea, activation enthalpy ΔH#, and activation entropy ΔS#. The determination of Ea is done using Equationequation 5(5)
(5) (Deepa Deepa Prabhu & Rao, Citation2019).
where R = 8.314 J mol−1 K−1, T = absolute temperature (in Kelvin), and B = a constant which differs on metal type
The ΔH# and ΔS# were calculated using Equationequation 6(6)
(6) :
where h = Plank’s constant (6.626 × 10−34 J.s), N = Avogadro’s number (6.022 × 1023 mol−1), and T = absolute temperature (in Kelvin)
2.7. Adsorption and thermodynamic factors
The isotherm is presented according to the best fit and correlation factor (R2) to obtain adsorption equilibrium constant (K) from Equationequation 7(7)
(7) .
where Cinh = inhibitor concentrations (in g/L) and θ = surface coverage
With the value of K, the standard free energy of adsorption (∆Goads) was calculated using equation 8 (D D Prabhu & Rao, Citation2013).
where R = 8.314 J mol−1 K−1, T = absolute temperature
The plot of ∆G°ads vs T was then used to obtain the standard enthalpy of adsorption (ΔH°ads) standard entropy of adsorption (ΔS°ads) using Equationequation 9(9)
(9) .
2.8. Scanning electron microscopy (SEM) and atomic force microscopy (AFM) analysis
The SEM of DP AISI1040 F-B steel immersed in 0.5 M H2SO4 solution with and without 5.0 g/L pectin inhibitor was investigated by capturing the images with the magnification of 1 K X using EVO MA18. The AFM spectral images were taken using IB342 Innova model for the surface of corroded and inhibited surfaces of DP AISI1040 F-B steel in the corrosive medium of 0.5 M H2SO4 with and without 5.0 g/L pectin.
The summary of methodology adopted during the experimental section has been presented in the form of flow chart as shown in .
3. Result and discussion
3.1. EIS method
The EIS measurements for the corrosion of DP AISI1040 F-B steel were performed in 0.5 M H2SO4 and with different amounts of pectin at 313 K is given in . The Nyquist plots exhibited a depressive capacitive loop in the high frequency (HF) range indicating the Faradic process at the electrode locations (Deepa & Padmalatha, Citation2017). Due to the non-uniform nature of the surface and surface inhomogeneity, the constant phase element (CPE) was used as a replacement for ideal capacitance/double-layer capacitance (C dl) (Majd et al., Citation2019). The HF capacitive loop can also be attributed to the charge transfer reaction and the time constant of the dual electrical layer. The equivalent circuit is made up of the constant phase element (CPE) in parallel with the charge transfer resistance (Rct). is obtained by adjusting the experimental data to the equivalent circuit model through ZSimpWin version 3.1 software. Comparing the experimental and calculated spectra confirms the relevance of the equivalent circuit adopted. The impedance parameters obtained are calculated and listed in .
Figure 5. Nyquist plots in 0.5 M H2SO4 comprising various concentrations of pectin at 313 K for the corrosion of DP AISI1040 F-B steel
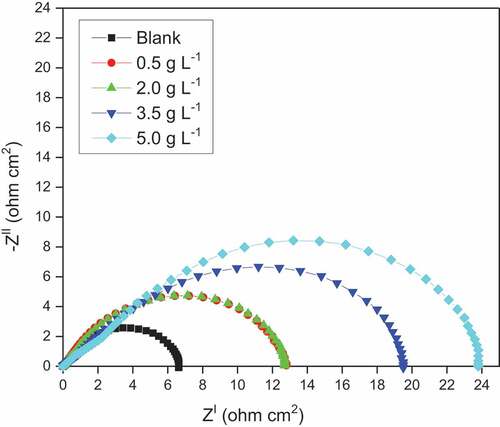
Table 1. Results of EIS measurements for the corrosion of DP AISI1040 F-B steel in 0.5 M H2SO4 comprising various concentrations of pectin at 313 K
in 0.5 M H2SO4 at 313 K
A double-layer capacitance (Cdl) is replaced by a CPE in the circuit used is to take into account the depressed capacitive nature of Nyquist plotted and the C dl can be computed using the equation. 10 (Machnikova et al., Citation2008; Toppo et al., Citation2013).
where Q = CPE, ωmax = maximum impedance rate and n = CPE exponent. If the value of n is 1, then CPE acts as a capacitor. The average value of n is 0.82. The slight change in capacity relative to its actual value was calculated using EquationEquation 11(11)
(11) .
fmax = is the frequency at the top of the semicircle (where-Z” is maximum). The polarization resistance (RP) was calculated using Equationequation 12(12)
(12) .
By adding pectin compound to the sulphuric acid solution, there is a decrease in the corrosion rate indicated by the increase in the Rp values of DP AISI1040 F-B steel through (i) pectin molecules adsorption onto the surface of the metal, (ii) decreasing in the rate of corrosion reaction, (iii) reduction in the rate of corrosive species diffusion into the metal surface and (iv) decreasing the electrical resistance of the metal surface (Izadi et al., Citation2017).
The value of Rp increased and Cdl declined with increased pectin concentration. Inhibitor molecule’s adsorption on the surface forming a physical barrier between the metal and corrosive. The increased thickness of the double layer at the metal-electrolyte interface caused a decrease in Cdl values. The Cdl reduction is attributed to the local dielectric constant reduction. Water molecules can be replaced by pectin molecules on the metal surface, leading to the local dielectric constant reduction (Izadi et al., Citation2017).
3.2. Potentiodynamic polarization (PDP) technique
PDP measurements for the corrosion of DP AISI1040 F-B steel were made in 0.5 M H2SO4 and with different quantities of pectin. PDP plots of corrosion are shown in ; it represents the corrosion of DP AISI1040 F-B steel in 0.5 M H2SO4 having various concentrations of pectin at 313 K. For the anodic Tafel slopes obtained (βa) and the cathodic slope of Tafel (βc), no substantial variation in values occurred, suggesting that there has not been a change in the mechanism of the anodic and cathodic process with pectin (Li & Deng, Citation2012). The corrosion potential value (Ecorr) was on average below ± 85 mV (Li et al., Citation2011; Nathan, Citation1973; Deepa Deepa Prabhu & Rao, Citation2019) suggesting that pectin act as a mixed type of inhibitor. The PDP results for DP AISI1040 F-B steel containing pectin at 313 K are shown in . The icorr values decreased with increased pectin concentration indicating increased effectiveness of pectin inhibition. The inhibition efficiency achieved from the PDP measurements was consistent with that achieved from the EIS studies.
Figure 7. PDP plots in 0.5 M H2SO4 comprising various concentrations of pectin at 313 K for the corrosion of DP AISI1040 F-B steel
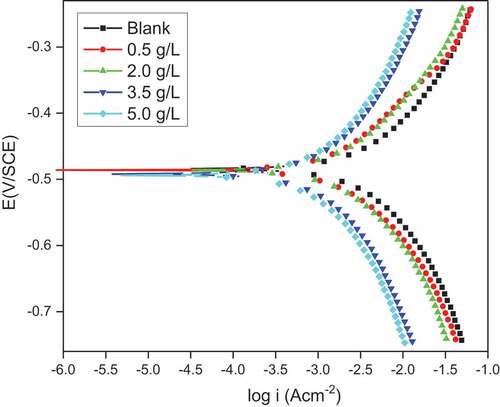
Table 2. Results of PDP measurements for the corrosion of DP AISI1040 F-B steel in 0.5 M H2SO4 comprising various concentrations of pectin at 313 K
3.3. Weight loss method
represents the effect of weight loss of DP AISI1040 F-B steel in 0.5 M H2SO4 at different immersion times and different temperatures. It is observed that weight loss increased with increasing time of exposure as well with increased temperature suggesting the enhanced corrosiveness to the metal on exposure. reflects the inhibition efficiency for pectin with the selected metal and medium. There was an increase in efficiency with the increase in pectin concentrations. Increased pectin concentration increases inhibition effectiveness to approximately 85% at 5.0 g/L for 1 h exposure time. The inhibitory action of the natural compound relative to the sample may be attributed to the adsorption of the pectin component onto the metallic surface. This adsorption confines the disintegration of the material by blocking the corrosion process. The inhibition efficiency decreased with time of exposure could be due to the degradation of pectin when exposed for a longer time. Thus from , it is clear that the plots have shown lesser inhibition efficiency (%) from 1 h to 7 h exposure time as well as with temperature from 313 K to 348 K.
3.4. Activation parameters and effect of temperature and immersion time
Arrhenius plot and ln (CR/T) vs 1/T in 0.5 M H2SO4 comprising pectin on DP AISI1040 F-B steel is shown in . Activation parameters for degradation of DP AISI1040 F-B steel in 0.5 M H2SO4 comprising pectin are depicted in . The value of Ea increased with increasing concentrations of pectin at each immersion time, showing that the energy barrier for the corrosion process (Amin et al., Citation2011). The values of Ea were larger for lesser exposure time, which could be due to lesser corrosion of metal at lesser immersion time. The ∆H# values varied correspondingly to that of Ea, matching the suggested mechanism (Deepa Deepa Prabhu et al., Citation2021). The ∆S# values were negative, characterizing the molecular association and decrease in disorder during the formation of the activated complex. (Oguzie et al., Citation2008).
Figure 10. (a) Arrhenius plots (b) Plots of ln (CR/T) versus 1/T in 0.5 M H2SO4 comprising various concentrations of pectin for the corrosion of DP AISI1040 F-B steel at 1 h, 3 h, 5 h, and 7 h immersion time
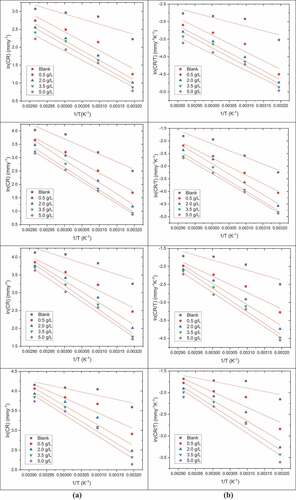
Table 3. Activation parameters for the corrosion of DP AISI1040 F-B steel in 0.5 M H2SO4 comprising various concentrations of Pectin at 1 h, 3 h, 5 h, and 7 h immersion time
shows the three-level effect, different immersion times, weight loss, and inhibition efficiency for different concentrations of pectin. Increased weight loss and reduced inhibition efficiency were observed with higher exposure time and temperature due to an increased corrosion process at a higher exposure time and physical adsorption of the inhibitor. There is an electrostatic interaction between pectin molecules and the metal surface. These electrostatic interactions between the inhibitor and the metal surface reduce their attractive force with raise in temperatures.
3.5. Adsorption and thermodynamic factors
Langmuir adsorption isotherm (Akinbulumo et al., Citation2020) observed with pectin for all the studied temperatures and the time of exposure is illustrated in (a). A plot of ∆G°ads versus T for pectin on DP AISI1040 F-B steel in 0.5 M H2SO4 for the above-mentioned conditions is given in . Thermodynamic values obtained for the pectin adsorption are given in .
Figure 12. (a) Langmuir adsorption isotherms (b) Plot of free energy of adsorption versus T for Pectin in 0.5 M H2SO4 for the corrosion of DP AISI1040 F-B steel for 1 h, 3 h, 5 h, and 7 h immersion time
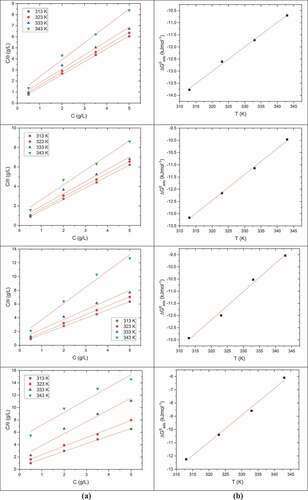
Table 4. Thermodynamic parameters for pectin adsorption on DP AISI1040 F-B steel at 0.5 M H2SO4 at various temperatures at 1 h, 3 h, 5 h, and 7 h immersion time
The obtained values of ΔG°ads at every immersion time were less than to(Galo et al., Citation2020) and the value went up with the increase in temperature, which suggested the physical adsorption of pectin on the metal surface (Fouda et al., Citation2009). The ΔH°ads values were negative, which is the indication of the exothermic process, which in addition supports the physisorption. ∆S°ads values were significant and negative, indicating the orderly placement of the inhibiting molecules on the metal surface. (Tang et al., Citation2003).
3.6. SEM and AFM analysis
The metal AISI1040 dual-phase F-B steel undergoes severe corrosion in the 0.5 M H2SO4 medium, which is observed in the two-dimensional SEM image. SEM is operated at a temperature below 298 K and humidity below 60 gm−3, the current is varied during scan and voltage of 10–15 kV under vacuum environment. In the metal surface seems to be even and smooth for the polished sample. shows the DP AISI1040 F-B steel in 0.5 M H2SO4 for 3 h, depict the uneven surface as a result of the corrosion of the metal and the deposition of the products of corrosion. is the image of the surface of the metal in presence of 5.0 g/L of pectin. The addition of pectin produced a uniform layer covering the surface and forming a barrier between the metal and the medium preventing deterioration.
Figure 13. SEM images of dual phase AISI1040 F-B steel (a) Polished surface (b) In 0.5 M H2SO4 (c) In 0.5 M H2SO4 and 5.0 g/L pectin
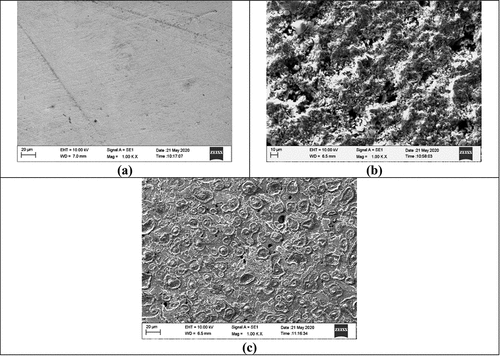
The AFM was operated at similar conditions of temperature and humidity as that of SEM, the current is varied at the probe and voltage varies between 1.6 and 1.8 V in a normal air environment. The AFM represents the three-dimensional images of DP AISI1040 F-B steel for the freshly polished, sample in represents the smooth uniform surface before the corrosion. represents the metal immersed in 0.5 M H2SO4. The rough surface is observed in the 3D representation of corroded metal. is for inhibited metal surface in the presence of 5.0 g/L pectin. The values of maximum roughness Rmax were 197 nm for polished, 5025 nm for corroded, and 1990 nm for the inhibited surface. This is a clear indication of the adsorbed pectin on the surface of the AISI1040 steel (Pais & Rao, Citation2020), due to a decrease in the Rmax value for the inhibited sample surface when compared with the corroded sample roughness value.
4. Conclusions
In this investigation, corrosion behavior and adsorption ability of pectin on heat-treated dual-phase AISI1040 steel with ferrite-bainite structure in 0.5 M sulphuric acid solution were done using weight loss and electrochemical techniques. Based on the data obtained, it is possible, to sum up, the following important points:
The pectin acts as a worthy eco-friendly inhibitor for the control of corrosion of DP AISI1040 F-B steel in 0.5 M H2SO4 solution at 313 K.
The optimum efficiency of 74.9 % is achieved for the addition of 5.0 g/L of pectin at 313 K by the EIS technique
PDP studies showed that pectin reduces both anodic and cathodic reactions thus acting as a mixed inhibitor on the surface of DP AISI1040 F-B steel.
In the weight-loss study, the highest inhibition efficiency of 85 % was achieved at 5.0 g/L at 1 h of exposure at 40 °C.
The IE of pectin increases with the increase in concentration and decreases with temperature, due to physical adsorption to the surface of the DP AISI1040 F-B steel and obeys Langmuir adsorption isotherm.
The energy and enthalpy of activation confirmed the formation of a protective barrier for the corrosion process.
The entropy of activation values was negative, indicating the association of molecules.
The free energy of adsorption values was close to
, which suggested spontaneous physical adsorption of pectin on the metal surface.
The enthalpy of adsorption indicated the exothermic process, and entropy of adsorption, indicating the arrangement of the molecules on the surface of the metal.
SEM and AFM studies have shown that the surface inhibitor film reduces the corrosion rate of DP AISI1040 F-B steel in 0.5 M sulphuric acid solution.
Nomenclature
EIS – Electrochemical Impedance Spectroscopy
PDP – Potentiodynamic Polarization
DP – Dual Phase
HSLA – High Strength Low Alloy
F-B – Ferrite Bainite
H2SO4 – Sulphuric Acid
OCP – open circuit potential
Rp – Polarisation resistance
Rct – Charge transfer resistance
Rs – Resistance of the solution
– Polarization resistance in the presence of pectin
– Polarization resistance in the absence of pectin
IE – Inhibition Efficiency
– Corrosion current density in the absence of pectin
– Corrosion current density in the presence of pectin
K – Constant
W – Difference in weight of the sample
D – Density of the sample
A – Sample surface area exposed to a corrosive medium
T – Exposed time
W1 – Difference between the initial and final sample weights in a corrosive environment without an inhibitor
W2 – Difference between the initial and final weight of the sample in an inhibitory corrosive environment
Ea – Activation energy
ΔH# – Activation enthalpy
ΔS# – Activation entropy
R2 – Correlation factor
K – Adsorption equilibrium constant
Cinh – Inhibitor concentration
θ – Surface coverage
CR – Corrosion Rate
T - Absolute temperature
∆Goads – Standard free energy of adsorption
ΔH°ads – Standard enthalpy of adsorption
ΔS°ads – Standard entropy of adsorption
SEM – Scanning Electron Microscopy
AFM – Atomic Force Microscopy
HF - High frequency
CPE – Constant phase element
Cdl – Double layer capacitance
ωmax – Maximum impedance rate
βa – Anodic Tafel slope
βc – Cathodic slope of Tafel
Ecorr – Corrosion potential
Rmax – Maximum roughness
Acknowledgements
The authors are thankful to the Department of Chemistry, Department of Mechanical Engineering, and Central Instrumentation Facilities MIT MAHE for lab and instrumental facilities.
Disclosure statement
The authors state that there is no conflict of interest in publishing this document.
Additional information
Funding
Notes on contributors
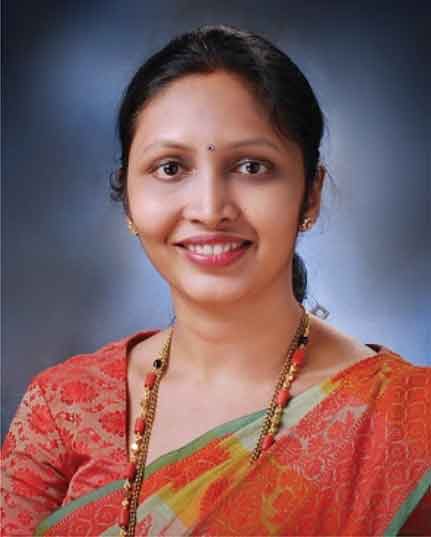
Deepa Prabhu
Dr. Deepa Prabhu is an Assistant Professor Senior Scale at the Department of Chemistry at the International Centre for Applied Sciences (ICAS), Manipal. She obtained her Master of Science in Organic Chemistry from Dept. of Science, Manipal Academy of Higher Education in 2011, and was awarded Doctor of Philosophy (Ph.D.) from the same University in 2015. She has received Best Paper Award in MR-12 in IIT Bombay and a Certificate of Appreciation for her Ph.D. work presentation. She has joined as an Assistant professor in ICAS and working since January 2015. She has published more than 30 research publications in various national and international journals (Scopus and web of Science indexed) and conference proceedings. Since 2015, she has served the organization in various capacities as a faculty member, faculty advisor for students, contributing member for the institutional and university activities, and guiding PhD scholar with MAHE intramural funding.
References
- Abedini, O., Behroozi, M., Marashi, P., Ranjbarnodeh, E., & Pouranvari, M. (2019). Intercritical heat treatment temperature dependence of mechanical properties and corrosion resistance of dual phase steel. Materials Research, 22(1)e20170969, http://www.scielo.br/scielo.php?script=sci_arttext&pid=S1516-14392019000100239&nrm=iso
- Akinbulumo, O. A., Odejobi, O. J., & Odekanle, E. L. (2020). Thermodynamics and adsorption study of the corrosion inhibition of mild steel by Euphorbia heterophylla L. extract in 1.5 M HCl. Results in Materials, 5(March), 100074. https://doi.org/10.1016/j.rinma.2020.100074
- Ali, D. (2019). Comparison of corrosion behaviors of bare Ti and TiO2. EmergingScience Journal, 3(4), 235–21. https://doi.org/10.28991/esj-2019-01185
- Amin, M. A., Ahmed, M. A., Arida, H. A., Arslan, T., Saracoglu, M., & Kandemirli, F. (2011). Monitoring corrosion and corrosion control of iron in HCl by non-ionic surfactants of the TRITON-X series – Part II. Temperature effect, activation energies and thermodynamics of adsorption. Corrosion Science, 53(2), 540–548. https://doi.org/10.1016/j.corsci.2010.09.019
- Basantia, S. K., Bhattacharya, A., Khutia, N., & Das, D. (2021). Plastic behavior of ferrite–pearlite, ferrite–bainite and ferrite–martensite steels: experiments and micromechanical modelling. Metals and Materials International, 27(5), 1025. https://doi.org/10.1007/s12540-019-00519-5
- Bentiss, F., Lebrini, M., & Lagrenée, M. (2005). Thermodynamic characterization of metal dissolution and inhibitor adsorption processes in mild steel/2,5-bis(n-thienyl)-1,3,4-thiadiazoles/hydrochloric acid system. Corrosion Science, 47(12), 2915–2931. https://doi.org/10.1016/j.corsci.2005.05.034
- Bignozzi, M. C., Calcinelli, L., Carati, M., Ceschini, L., Chiavari, C., Masi, G., & Morri, A. (2020). Effect of heat treatment conditions on retained austenite and corrosion resistance of the X190CrVMo20-4-1 stainless steel. Metals and Materials International, 26(9), 1318–1328. https://doi.org/10.1007/s12540-019-00384-2
- Bilal, M. M., Yaqoob, K., Zahid, M. H., haq, E. U., Tanveer, W. H., Wadood, A., & Ahmed, B. (2019). Effect of austempering conditions on the microstructure and mechanical properties of AISI 4340 and AISI 4140 steels. Journal of Materials Research and Technology, 8(6), 5194–5200. https://doi.org/10.1016/j.jmrt.2019.08.042
- Charitha, B. P., & Rao, P. (2020). Pectin as a potential green inhibitor for corrosion control of 6061al–15%(v) sic(p) composite in acid medium: electrochemical and surface studies. Journal of Failure Analysis and Prevention, 20(5), 1684–1696. https://doi.org/10.1007/s11668-020-00973-z
- Deepa, P., & Padmalatha, R. (2017). Corrosion behaviour of 6063 aluminium alloy in acidic and in alkaline media. Arabian Journal of Chemistry, 10(2), S2234–S2244. https://doi.org/10.1016/j.arabjc.2013.07.059
- Fereiduni, E., & Ghasemi Banadkouki, S. S. (2014). Improvement of mechanical properties in a dual-phase ferrite–martensite AISI4140 steel under tough-strong ferrite formation. Materials & Design (1980-2015), 56(56), 232–240. https://doi.org/10.1016/j.matdes.2013.11.005
- Fouda, A. S., Al-Sarawy, A. A., Ahmed, F. S., & El-Abbasy, H. M. (2009). Corrosion inhibition of aluminum 6063 using some pharmaceutical compounds. Corrosion Science, 51(3), 485–492. https://doi.org/10.1016/j.corsci.2008.10.012
- Galo, G. T., Morandim-Giannetti, A. D. A., Cotting, F., Aoki, I. V., & Aquino, I. P. (2020). Evaluation of purple onion (allium cepa l.) extract as a natural corrosion inhibitor for carbon steel in acidic media. Metals and Materials International. https://doi.org/10.1007/s12540-020-00679-9
- Gerengi, H., Sen, N., Uygur, I., & Kaya, E. (2020). Corrosion behavior of dual phase 600 and 800 steels in 3.5 wt.% NaCl environment. Journal of Adhesion Science and Technology, 34(8), 903–915. https://doi.org/10.1080/01694243.2019.1688925
- Gürkan Aydın, A. Y. (2019). Effect of Quenching and Tempering Temperature on Corrosion Behavior of Boron Steels in 3.5 wt.% NaCl Solution. . International Journal of Electrochemical Science, 14, 2126–2135. https://doi.org/10.20964/2019.03.22
- Izadi, M., Shahrabi, T., & Ramezanzadeh, B. (2017). Electrochemical investigations of the corrosion resistance of a hybrid sol–gel film containing green corrosion inhibitor-encapsulated nanocontainers. Journal of the Taiwan Institute of Chemical Engineers, 81, 356–372. https://doi.org/10.1016/j.jtice.2017.10.039
- Karimi, A., Kheirandish, S., Mahmoudiniya, M. (2017). Effect of bainite volume fraction on mechanical properties of a ferrite-bainite-martensite steel. Kovove Mater, 55(3), 175–182.
- Katiyar, P. K., Misra, S., & Mondal, K. (2019). Comparative corrosion behavior of five microstructures (pearlite, bainite, spheroidized, martensite, and tempered martensite) made from a high carbon steel. Metallurgical and Materials Transactions A, 50(3), 1489–1501. https://doi.org/10.1007/s11661-018-5086-1
- Keleştemur, O., Aksoy, M., & Yildiz, S. (2009). Corrosion behavior of tempered dual-phase steel embedded in concrete. International Journal of Minerals, Metallurgy and Materials, 16(1), 43–50. https://doi.org/10.1016/S1674-4799(09)60008-X
- Kumar, S., Kumar, A., Vinaya, M., Sah, R., R., & Manjini, S. (2019). Mechanical and electrochemical behavior of dual-phase steels having varying ferrite–martensite volume fractions. Journal of Materials Engineering and Performance, 28(6), 3600–3613. https://doi.org/10.1007/s11665-019-04101-3
- Li, X., & Deng, S. (2012). Inhibition effect of Dendrocalamus brandisii leaves extract on aluminum in HCl, H3PO4 solutions. Corrosion Science, 65, 299–308. https://doi.org/10.1016/j.corsci.2012.08.033
- Li, X., Deng, S., & Fu, H. (2011). Sodium molybdate as a corrosion inhibitor for aluminium in H3PO4 solution. Corrosion Science, 53(9), 2748–2753. https://doi.org/10.1016/j.corsci.2011.05.002
- Loto, R. T., & Loto, C. A. (2020). Inhibition effect of apium graveolens, punica granatum, and camellia sinensis extracts on plain carbon steel. Cogent Engineering, 7(1), 1798579. https://doi.org/10.1080/23311916.2020.1798579
- Lowmunkhong, P., Ungthararak, D., & Sutthivaiyakit, P. (2010). Tryptamine as a corrosion inhibitor of mild steel in hydrochloric acid solution. Corrosion Science, 52(1), 30–36. https://doi.org/10.1016/j.corsci.2009.08.039
- Ma, J., Feng, F., Yu, B., Chen, H., & Fan, L. (2020). Effect of cooling temperature on the microstructure and corrosion behavior of X80 pipeline steel. International Journal of Minerals, Metallurgy and Materials, 27(3), 347–353. https://doi.org/10.1007/s12613-019-1882-x
- Machnikova, E., Whitmire, K. H., & Hackerman, N. (2008). Corrosion inhibition of carbon steel in hydrochloric acid by furan derivatives. Electrochimica Acta, 53(20), 6024–6032. https://doi.org/10.1016/j.electacta.2008.03.021
- Mahgoub, F. M., Abdel-Nabey, B. A., & El-Samadisy, Y. A. (2010). Adopting a multipurpose inhibitor to control corrosion of ferrous alloys in cooling water systems. Materials Chemistry and Physics, 120(1), 104–108. https://doi.org/10.1016/j.matchemphys.2009.10.028
- Majd, M. T., Shahrabi, T., & Ramezanzadeh, B. (2019). Low carbon steel surface modification by an effective corrosion protective nanocomposite film based on neodymium-polyacrylic acid-benzimidazole. Journal of Alloys and Compounds, 783, 952–968. https://doi.org/10.1016/j.jallcom.2018.12.367
- Mert, B. D., Mert, M. E., Kardaş, G., & Yazıcı, B. (2011). Experimental and theoretical investigation of 3-amino-1,2,4-triazole-5-thiol as a corrosion inhibitor for carbon steel in HCl medium. Corrosion Science, 53(12), 4265–4272. https://doi.org/10.1016/j.corsci.2011.08.038
- Nathan, C. C. (1973). Corrosion Inhibitors. National Association of Corrosion Engineers. https://books.google.co.in/books?id=Bb5RAAAAMAAJ
- Negm, N. A., Kandile, N. G., Aiad, I. A., & Mohammad, M. A. (2011). New eco-friendly cationic surfactants: Synthesis, characterization and applicability as corrosion inhibitors for carbon steel in 1N HCl. Colloids and Surfaces. A, Physicochemical and Engineering Aspects, 391(1), 224–233. https://doi.org/10.1016/j.colsurfa.2011.09.032
- Obi-Egbedi, N. O., Obot, I. B., & Umoren, S. A. (2012). Spondias mombin L. as a green corrosion inhibitor for aluminium in sulphuric acid: Correlation between inhibitive effect and electronic properties of extracts major constituents using density functional theory. Arabian Journal of Chemistry, 5(3), 361–373. https://doi.org/10.1016/j.arabjc.2010.09.002
- Oguzie, E. E., Njoku, V. O., Enenebeaku, C. K., Akalezi, C. O., & Obi, C. (2008). Effect of hexamethylpararosaniline chloride (crystal violet) on mild steel corrosion in acidic media. Corrosion Science, 50(12), 3480–3486. https://doi.org/10.1016/j.corsci.2008.09.017
- Olugbade, T., Liu, C., & Lu, J. (2019). Enhanced Passivation Layer by Cr Diffusion of 301 Stainless Steel Facilitated by SMAT. Advanced Engineering Materials, 21(8), 1900125. https://doi.org/10.1002/adem.201900125
- Olugbade, T., & Lu, J. (2019a). Characterization of the Corrosion of Nanostructured 17-4 PH Stainless Steel by Surface Mechanical Attrition Treatment (SMAT). Analytical Letters, 52(16), 2454–2471. https://doi.org/10.1080/00032719.2019.1611842
- Olugbade, T., & Lu, J. (2019b). Enhanced Corrosion Properties of Nanostructured 316 Stainless Steel in 0.6 M NaCl Solution. Journal of Bio- and Tribo-Corrosion, 5(2), 38. https://doi.org/10.1007/s40735-019-0235-7
- Olugbade, T. O. (2021). Electrochemical Characterization of the Corrosion of Mild Steel in Saline Following Mechanical Deformation. Analytical Letters, 54(6), 1055–1067. https://doi.org/10.1080/00032719.2020.1793994
- Pais, M., & Rao, P. (2020). Maltodextrin for corrosion mitigation of zinc in sulfamic acid: Electrochemical, surface and spectroscopic studies. International Journal of Biological Macromolecules, 145, 575–585. https://doi.org/10.1016/j.ijbiomac.2019.12.197
- Panchenkoa, Y. M., Marshakova, A. I.,Nikolaeva, L. A., & Igonin, T. N. (2020). Estimating the first-year corrosion losses of structural metals for continental regions of the world. Civil Engineering Journal, 6(8), 1503–1519. https://doi.org/10.28991/cej-2020-03091563
- Prabhu, D., Prabhu, P. R., & Rao, P. (2021). Thermodynamics, adsorption, and response surface methodology investigation of the corrosion inhibition of aluminum by Terminalia chebula Ritz. extract in H3PO4. Chemical Papers, 75(2), 653–667. https://doi.org/10.1007/s11696-020-01318-8
- Prabhu, D., & Rao, P. (2013). Coriandrum sativum L. - A novel green inhibitor for the corrosion inhibition of aluminium in 1.0 M phosphoric acid solution. Journal of Environmental Chemical Engineering, 1(4), 676–683. https://doi.org/10.1016/j.jece.2013.07.004
- Prabhu, D., & Rao, P. (2019). A Comparative Study on Inhibitory Actions of TCE, GIE, and CSE on the Corrosion of 6063 Al Alloy in H3PO4 Medium. Journal of Bio- and Tribo-Corrosion, 5(3), 76. https://doi.org/10.1007/s40735-019-0269-x
- Prabhu, P. R., Prabhu, D., & Rao, P. (2020). Analysis of Garcinia indica Choisy extract as eco-friendly corrosion inhibitor for aluminum in phosphoric acid using the design of experiment. Journal of Materials Research and Technology, 9(3), 3622–3631. https://doi.org/10.1016/j.jmrt.2020.01.100
- Prabhu, P. R., Prabhu, D., Sharma, S., & Kulkarni, S. M. (2020). Surface Properties and Corrosion Behavior of Turn-Assisted Deep-Cold-Rolled AISI 4140 Steel. Journal of Materials Engineering and Performance, 29(9), 5871–5885. https://doi.org/10.1007/s11665-020-05051-x
- Qiang, Y., Zhang, S., Tan, B., & Chen, S. (2018). Evaluation of Ginkgo leaf extract as an eco-friendly corrosion inhibitor of X70 steel in HCl solution. Corrosion Science, 133, 6–16. https://doi.org/10.1016/j.corsci.2018.01.008
- Şahin, E. A., Solmaz, R., Gecibesler, İ. H., & Kardaş, G. (2020). Adsorption ability, stability and corrosion inhibition mechanism of phoenix dactylifera extrat on mild steel. Materials Research Express, 7(1), 16585. https://doi.org/10.1088/2053-1591/ab6ad3
- Salamci, E., & S. Candan, F. K. (2017). Effect of microstructure on corrosion behavior of dual-phase steels. Kovove Mater, 5(2), 133–139. https://doi.org/10.4149/km_2017_2_133
- Salman, T. A., Jawad, Q. A., Hussain, M. A. M., Al-Amiery, A. A., Shaker, L. M., Kadhum, A. A. H., & Takriff, M. S. (2020). New environmental friendly corrosion inhibitor of mild steel in hydrochloric acid solution: Adsorption and thermal studies. Cogent Engineering, 7(1), 1826077. https://doi.org/10.1080/23311916.2020.1826077
- Shahzad, M., Tayyaba, Q., Manzoor, T., ud-din, R., Subhani, T., & Qureshi, A. H. (2018). The effects of martensite morphology on mechanical properties, corrosion behavior and hydrogen assisted cracking in A516 grade steel. Materials Research Express, 5(1), 16516. https://doi.org/10.1088/2053-1591/aaa55f
- Shinato, K. W., Zewde, A. A., & Jin, Y. (2020). Corrosion protection of copper and copper alloys in different corrosive medium using environmentally friendly corrosion inhibitors. Corrosion Reviews, 38(2), 101–109. https://doi.org/10.1515/corrrev-2019-0105
- Solomon, M. M., Umoren, S. A., Udosoro, I. I., & Udoh, A. P. (2010). Inhibitive and adsorption behaviour of carboxymethyl cellulose on mild steel corrosion in sulphuric acid solution. Corrosion Science, 52(4), 1317–1325. https://doi.org/10.1016/j.corsci.2009.11.041
- Song, D., Hao, J., Yang, F., Chen, H., Liang, N., Wu, Y., … Jiang, J. (2019). Corrosion behavior and mechanism of Cr–Mo alloyed steel: Role of ferrite/bainite duplex microstructure. Journal of Alloys and Compounds, 809, 151787. https://doi.org/10.1016/j.jallcom.2019.151787
- Song, D., Wang, G., Yang, F., Chen, H., Liang, N., Ma, H., … Ma, X. (2020). Microstructure and deformation behavior of a novel steel rebar: Effect of the heterogeneous microstructure of soft ferrite and Hard bainite. Journal of Materials Research and Technology, 9(6), 12281–12292. https://doi.org/10.1016/j.jmrt.2020.08.085
- Tabatabaei majd, M., Naderi, R., & Ramezanzadeh, B. (2020). Promotion of the active/barrier protection function of epoxy ester coating/steel system utilizing differently synthesized hybrid pigment through zinc acetylacetonate tailored with green inhibitor molecules. Progress in Organic Coatings, 138, 105380. https://doi.org/10.1016/j.porgcoat.2019.105380
- Tang, L., Mu, G., & Liu, G. (2003). The effect of neutral red on the corrosion inhibition of cold rolled steel in 1.0 M hydrochloric acid. Corrosion Science, 45(10), 2251–2262. https://doi.org/10.1016/S0010-938X(03)00046-5
- Toppo, A., Kaul, R., Pujar, M. G., Kamachi Mudali, U., & Kukreja, L. M. (2013). Enhancement of Corrosion Resistance of Type 304 Stainless Steel Through a Novel Thermo-mechanical Surface Treatment. Journal of Materials Engineering and Performance, 22(2), 632–639. https://doi.org/10.1007/s11665-012-0304-2
- Xiong, Z., Kostryzhev, A. G., Zhao, Y., & Pereloma, E. V. (2019). Microstructure Evolution during the Production of Dual Phase and Transformation Induced Plasticity Steels Using Modified Strip Casting Simulated in the Laboratory. Metals, 9(4), 449. https://doi.org/10.3390/met9040449
- Zhang, Q., Li, Q., & Chen, X. (2020). Effect of heat treatment on corrosion behavior of Mg–5Gd–3Y–0.5Zr alloy. RSC Advances, 10(71), 43371–43382. https://doi.org/10.1039/D0RA08933H
- Zhao, Z., Wang, X., Qiao, G., Zhang, S., Liao, B., & Xiao, F. (2019). Effect of bainite morphology on deformation compatibility of mesostructure in ferrite/bainite dual-phase steel: Mesostructure-based finite element analysis. Materials & Design, 180, 107870. https://doi.org/10.1016/j.matdes.2019.107870
- Zhu, J. G. (2019). Bainite transformation kinetics-microstructure characterization of austempered 4140 Steel. Metals - Open Access Metallurgy Journal, 10(2), 236. https://doi.org/10.3390/met10020236