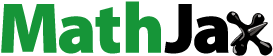
Abstract
The strength behavior of Corn Husk Ash polymer concrete reinforced with coconut fibre was investigated in this study. The study assessed the suitability of using coconut fibre in concrete to improve its strength properties. The concrete was prepared and cured based on British Standard. Different proportions of the coconut fibre as well as the Corn Husk Ash (CHA) polymer were used in the concrete mixes, with a total of nineteen different mix proportions. The specimens were cured for 28, 90 and 180 days. Compressive strength, tensile splitting strength, torsion and toughness were determined using specimens from the various mixes. Tests were conducted on the 28th, 90th and 180th days of curing, following (Compression) and (Tensile Splitting). Analysis of Variance (ANOVA) test was conducted to determine the differences in the behavior of the different mixes as well as the correlation between the different constructs. The addition of coconut fibre to the concrete improved the toughness, torsion and to some extent the tensile strength of the concrete at the standard curing age of 28 days. CHA as an additive to the concrete resulted in a positive relationship with the Torsion and Toughness properties of concrete at later curing age at 1% level of significance (r = 0.480 and r = 0.730, p < 0.01 respectively). Compressive strength of concrete correlated positively with tensile strength, torsion and toughness of concrete (r = 0.830, r = 0.721 and r = 0.452 respectively with p < 0.01).
PUBLIC INTEREST STATEMENT
The study assessed the possibility of using coconut fibre in concrete to improve its strength properties, as well as find use for the volumes of agricultural residues including coconut fibre and corn husk which would otherwise be wasted. Corn husk ash was used as an admixture in the concrete to protect coconut fibres from degradation. The addition of coconut fibre to concrete improved the strength properties of the concrete. The increase in toughness, torsion and the tensile strength could be attributed to the fact that, the fibre presence in the concrete contributed greatly in offering restraint to early twist or strain in the concrete. The introduction of coconut fibre in concrete for construction is therefore recommended with the addition of corn husk ash polymer. This concrete can be used for housing in rural communities with abundance of the agricultural residues, to reduce the rising cost of building.
1. Introduction
Concrete made with Ordinary Portland cement is strong in compression but weak in tension and tends to be brittle. The weakness in tension can be overcome using materials that are good in tension such steel bars and sufficient quantities of certain fibres. The addition of fibres could change the performance of the fibre-matrix composite, resulting in the enhancement of the energy absorption capacity, of concrete (Momoh & Osofero, Citation2020). The addition of fibres could improve the bonding of reinforcing bars and thus enhance the bearing capacity and other performance attributes of the reinforced concrete (Zhou et al., Citation2020). The prevention of shrinkage cracks may contribute to reduction in the material permeability as well (Gutierrezi et al., Citation2005). Synthetic and natural fibres have both been used to improve the performance of concrete (Al-Oraimi & Seibi, Citation1995). The use of synthetic fibres however have adverse effect on the environment. According to CoDyre et al. (Citation2018), synthetic fibres have high environmental impact. The authors cited carbon and glass fibres production as having energy consumption values of 355 MJ/kg and 31.7 MJ/kg respectively. P. P. Yalley and Kwan (Citation2009) suggested natural fibres as close substitute to synthetic fibres. Natural fibres exhibit a wide range of tensile strength and stiffness with low energy requirements. Natural fibres such as flax, palm fibres, coconut fibres, etc. have been used in concrete to improve its properties. The fibres from coconut, palm, sisal, flax, straw, bamboo, jute and cane have a long history of use since they are readily available and can easily be procured from the natural environment (Hejazi et al., Citation2012). Ku et al. (Citation2011) postulated that flax fibres has tensile strength between 500 and 1500 N/mm2, modulus of elasticity of 25,600 N/mm2 and an embodied energy of 2.75 MJ/kg. P. P. Yalley and Kwan (Citation2009) used coconut fibre to enhance concrete and the toughness of the concrete was improved, however, the long-term durability was not studied. The coconut fibres that were used were from Ghana. The motivation for the use of the coconut fibres, according to P. P. Yalley and Kwan (Citation2009) were their availability and cheaper cost.
Gutierrezi et al. (Citation2005) proposed that the fibre-reinforced concrete characteristics could be improved by including supplementary cementitious material (SCM). The most common SCMs presently used are silica fume, granulate blast furnace slag and fly ash (Co Dyre et al., Citation2018). Adding pozzolanic by-products to Portland cement are effective in preventing degradation of natural fibres. This is because cement based polymers tend to dilute the alkaline content in the cement hence improving the durability of the concrete fibre composite (Zhang & Li, Citation2013). There has been an increase in research on the usage of cementitious polymers which are economically and environmentally friendly (P. P. K. Yalley & Asiedu, Citation2013). The areas that P. P. K. Yalley and Asiedu (Citation2013) gave adequate attention included the use of agricultural materials as alternative cementitious polymers, and opined that corn husks are readily available, has no economic value and an environmentally friendly material.
In the view of Halm et al. (Citation2004), corn is the largest staple food in Ghana as it accounts for 50–60% of the total cereals produced. It currently has an annual production of 1.7 million metric tons indicating a growth rate of 13.33%, which accounts for more than 4% of the country’s agricultural gross domestic product. A study by Ahenkora et al. (Citation1999) revealed that corn husk is used as human food and silage for animals. Despite having been associated with many applications in Ghana, it is disposed-off along with corn stalk and leaves either by burning or tilling into the soil (P. P. K. Yalley & Asiedu, Citation2013). As a way of ensuring a sustainable use, this study focused on ways of utilizing corn husk in fibre-concrete production with the aim of improving the strength characteristics in the long term. The utilization of corn husk ash (CHA) as a cementitious material to supplement cement to improve the strength of fibre-reinforced concrete was investigated in this research.
2. Materials and methods
2.1. Materials
Ordinary Portland Cement from Ghana Cement Limited was used. They were produced in accordance with the requirements of (BS EN 197-1, Citation2011). Natural sand and crushed granite of maximum size 10 mm were used as aggregates for the concrete production following (BS EN 12620, Citation2002). Water used for the concrete conformed to (BS EN Citation1008, 2002). The coconut fibres with aspect ratio of 125 were used based on the recommendations of P. P. Yalley and Kwan (Citation2009). The corn husk ash as a cementitious polymer conformed to (BS EN 450-1, Citation2012).
The chemical composition of the Ordinary Portland Cement and the Corn Husk Ash used in the study is presented in .
Table 1. Chemical composition of Ordinary Portland Cement (OPC) and Corn Husk Ash (CHA)
2.2. Concrete preparation and methods
Mixing, casting, compaction and curing were based on (BS EN 206, Citation2013). Slump height were taken as a grading for workability of the concrete. Appropriate super-plasticizer was added to the concrete to maintain a standard workability. Different proportions of the coconut fibre as well as the CHA polymer were used in the concrete mixes. There was a control mix (specimens without fibres and CHA polymer) labelled as mix A, and mix Ci/j (specimens with i% fibre and j% CHA polymer). A total of 19 different mix proportions were thus cast for the study. The mix proportions for each of the mixes are listed in . Steel moulds with dimensions of 100 mm x 100 mm x 100 mm were used for casting cubes for compressive strength test, 100 mm x 200 mm cylinders were cast for tensile splitting and torsion tests. Three (3) specimens were cast for each test for all the days of testing. In all 342 specimens of cubes and cylinders were cast. The concrete cubes and the cylinders were removed from the moulds 24 hours after casting and immersed in water for curing. The specimens were tested on the 28th, 90th and 180th days of curing.
Table 2. Different mixes and Mix proportions (quantities) used in the study
2.3. Compressive strength test
Three cubes and three cylinders from each mix were tested for compression in line with (BS EN 12390-3, Citation2019) at day 28, 90 and 180 after casting using a GD10A compression testing machine with a maximum capacity of 2500KN ().
2.4. Tensile splitting strength test
Tensile splitting strength test was conducted following (BS EN 12390-6, Citation2009). In the splitting tensile tests cylindrical specimen were subjected to splitting tension along their axis. 100 mm x 200 mm cylinders were used for the test.
The tensile splitting strength was calculated by the formula:
where
fct is the tensile splitting strength, in Newton per square millimetre
F is the maximum load, in Newton
L is the length of the line of contact of the specimen, in millimetres
d is the diameter of the cylinder, in millimetres.
2.5. Torsion test
The samples for the torsional test were first roughened at the ends with grinder. Steel rings, with Poly paste applied to the inner perimeter, were fixed to each end of the samples. The Poly paste then dried and hardened and formed a strong bond between the rings and the concrete. The samples were tested after one day of preparation. The steel rings had protruding radial arms, which could be pushed to induce a torque in the concrete cylinder. The cylinder was set up as shown in . A twisting load was applied, and the load and respective angle of displacements were record
The applied torque, T is given by:
where,
PT = applied load causing torsion (N), (see ).
Z = Distance between the point of application of the load and the centre of the cylinder (mm). (Z = 0.13 m)
3. Result and discussion
3.1. Behaviour under compression
shows the variation of compressive strength for plain concrete and concrete with CHA content containing three different coconut fibre contents. Discussions are based on the average results of three samples tested.
3.1.1. Influence of coconut fibre content on compressive strength
At the normal curing age of 28 days, there was an average reduction of 11%, 23% and 26% respectively in compressive strength for concrete with 0.25% (C0.25/0), 0.5% (C0.5/0) and 0.75% (C0.75/0) fibre content but without CHA polymer as shown in . It was further noted that at latter age of 90 days and 180 days, the compressive strengths of the specimens with fibre addition but without CHA polymer deteriorated further compared to the plain concrete. The reduction in the compressive strengths were 34%, 21% and 37% lower than the plain concrete at the 180th day. The plain concrete however, increased in strength with increasing curing age.
The additional reduction of concrete with coconut fibre but without cementitious polymers (CHA) might be due to the fact that the coconut fibres might have absorbed more water during the six-month immersion in water, causing unstable volume and subsequent poor cohesion between the fibre-matrix interface.
3.1.2. Influence of CHA on compressive strength
At constant fibre weight fractions, Concrete specimens with 20% CHA polymer had the highest compressive strength at all the curing ages (). For all the CHA polymer content, specimens with 0.5% CF content had the highest compressive strength at both 90 and 180 days curing ages. At the 28-day curing age, the compressive strength of the plain concrete was higher than all the specimens with CHA polymer replacement. It was also noted that at the 28-day curing age, the compressive strengths reduced with increase in the CHA polymer content. A higher CHA polymer content meant lower content of OPC, hence lower early age strength, since the OPC performs relatively better at early ages than pozzolanic polymers. This agrees with findings of Dembovska et al. (Citation2017).
The specimens with CHA polymer content provided better performance in compressive strength at 90th day and 180th day curing. This could be explained that the CHA polymers diluted the alkaline content in the cement hence improved the strength of the concrete fibre composite with time. From , at the same coconut fibre content, specimens with 20% CHA polymer content had the highest compressive strength at the latter ages.
The addition of fibres, however, adversely affected the compressive strength. This might be due to the difficulties in compaction which consequently created voids in the concrete specimens. The further decrease in the compressive strength of fibre reinforced concrete at the 90th and 180th day testing period might be due to the degradation of the fibres in the alkaline environment of the concrete resulting in high volume of voids and hence reduction in the compressive strength. Adding pozzolanic by-products (CHA polymer) to OPC was effective in preventing degradation of natural fibres and possible reduction of the permeability of the concrete leading to reduction in bleeding and thus increased the compressive strength at the latter ages (Ramakrishna & Sundara, Citation2005; Romildo et al., Citation2000).
3.2. Tensile strength
3.2.1. Influence of coconut fibre (CF) on tensile strength
compares the tensile strength of specimens with the three fibre weight fractions (0,25, 0.50 and 0.75%) without the CHA polymer. For higher percentages (0.5% and 0.75%) of coconut fibre without CHA polymer content, the cylindrical specimens had only cracks in the specimen, but did not split into two halves like those of the plain concrete as shown in . In other words, the fibre weight fraction of 0.25% did not improve the splitting tensile strength and resulted in the reduction of an average of 23% for the concrete at the 28th day curing age as indicated in . At the 180th day of curing, the tensile strength had reduced by an average of 25% from the initial tensile strength for all fibre weight fractions. The CF might have degraded creating more voids, hence the decrease in the tensile strength of concrete specimens without the CHA polymer.
3.2.2. Influence of CHA on tensile strength
At constant fibre weight fractions, specimens with 20% CHA polymer had the highest tensile strength at all the curing ages (). The tensile strength of the specimens increased with increasing percentage of the CHA polymer up to 20%. The tensile strength reduced with further increase in the CHA amount (i.e. 25% and 30%). At the 180th day, the tensile strength, was on the average 9%, 21%, 54%, 40% and 28% higher than the 28th day strength for specimens with 10%, 15%, 20%, 25% and 30% CHA polymer content respectively. This then implied that the pozzolanic reaction in the CHA polymer was effective at the latter age of curing. The best performing specimens were those with 0.5% CF and 20% CHA polymer contents, and it was about 40% higher over the plain concrete after 180 days of curing.
3.3. Behaviour under torsion
The behaviour of coconut fibre-reinforced polymer concrete under pure torsion was investigated.
When fibre was added to plain concrete the torsion strength increased by between 10% and 20% (). The optimum fibre weight fraction was 0.5%, beyond which the torsional strength started to decrease. For the same fibre content, results showed that there was an optimum CHA polymer content of 20% for 28, 90 and 180 days. Specimens without CHA polymers lost between 12% and 37% of their torsional strength on the 180th day. It was noted that the specimens with CHA polymers were able to maintain their torsional strength at the latter days of curing. This could be attributed to the fact that the CHA polymer was efficient in protecting the fibre hence the specimens resisted the twist due to torsion. It could also be deduced that the fibres were solely responsible for the torsional strength. The CHA was only responsible for protecting the fibres from being decomposed by the hydration by-product of the cement matrix.
Table 3. Average torsion and toughness of specimens under different CF and CHA combinations
3.4. Toughness
Generally, coconut fibre-reinforced concrete could absorb much more energy before failure compared with the plain concrete at all curing ages (). The optimum weight percentage of fibre and CHA polymer for this investigation were 0.5% and 20% respectively (C0.5/20). The total area under torsion-twist curve, which measures toughness, increased substantially with the addition of coconut fibre, by between 10% and 36% at all curing ages, resulting in a more ductile behaviour. It was noted again that specimens without the inclusion of CHA polymer lost their energy absorbing capacity at the 180th day of curing. Nanayakkaza et al. (Citation2005) explained that the high lignin content of natural fibres is responsible for enhanced toughness in fibre reinforced concrete. The lignin content is responsible for stiffening the cell-wall of the fibre preventing embrittlement of the fibres. The toughness of the specimens with CHA polymers were maintained with slight increase in specimens with higher percentage of CHA polymers. The improvement in ductility is more pronounced in specimen with fibre weight fraction of 0.5% and CHA polymer content of 20%.
3.5. Correlation among construct
shows that at the 5% level of significance, coconut fibre added to concrete production has a positive relationship with the torsion property of concrete (r = 0.321, p < 0.05). On the other hand, corn husk ash as an additive to concrete resulted in a positive relationship with the Torsion and Toughness properties of concrete at later curing age at 1% level of significance (r = 0.480 and r = 0.730, p < 0.01 respectively). Curing days as a determiner of hardened concrete properties was also found to increase the compressive and tensile strengths as well as the torsion and toughness properties of concrete with CHA at a unit increment of the curing days. It is worth noting that coconut fibre had a negative relationship with the compressive strength however it was not statistically significant (r = −0.062, p > 0.05). It is again observed in that compressive strength of concrete correlated positively with tensile strength, torsion and toughness of concrete (r = 0.830, r = 0.721 and r = 0.452 respectively with p < 0.01).
Table 4. Correlation among study construct
3.6. Corn husk ash influence on compressive strength at 180 days of curing
It is worth noting in that, compressive strength of concrete was significantly credible at the age of 180 days of curing due to the presence of corn husk ash in the concrete. The corn husk ash was found to significantly compensate the strength loss due to the presence of pores caused by the coconut fibre in the concrete. This was found to be true in the ANOVA generated by the model using stepwise regression analysis which makes it adequate to predict the strength of concrete (F = 8.661, p < 0.05). Thus, the coefficient of multiple determination, R2 shows that, corn husk ash explains about 34% of the variations in the compressive strength of fibre concrete at 180 days of curing. That is, one percent increment in the corn husk ash will result in 0.325 N/mm2 increment in the compressive strength of concrete (t = 2.943, p < 0.05). The minimum contribution supports the fact that, strength of concrete largely depends on the basic concrete materials, other than the additives. The predicting model is hence given as
Table 5. Regression coefficients of compressive strength of fibre concrete at 180 days curing
where fcu180 is the compressive strength at the 180th day of curing
shows that, corn husk ash contributes an increase of 0.036MPa to the tensile strength of fibre concrete at one percent increase in CHA (t = 2.943, p < 0.05). The coefficient of multiple determination, R2 shows that, corn husk ash explains about 27% of the variation in the tensile strength of concrete. The predicting model is found to be adequate for doing so due to the ANOVA value, F = 6.299, p < 0.05. Coconut fibre was excluded in the model due to its insignificant contribution to the strength of the concrete (t = −0.666, p > 0.05).
Table 6. Regression coefficients of tensile strength of fibre concrete at 180 days of curing
presents how much corn husk ash explains the variations in the torsional strength of concrete. It is noted that, at one percent increase in the corn husk ash, the torsional strength of fibre concrete increased at 0.026MPa (t = 4.705, p < 0.01) whereas the coconut has no significant contribution to the torsional strength of concrete hence excluded from the predicting model (t = 0.943, p > 0.05). However, the ANOVA analysis indicates the model can adequately be used to predict the strength of fibre concrete (F = 22.135, p < 0.01). The coefficient of multiple determination, R2 shows the corn husk explains about 57% of the variations in the strength of concrete. The predicting model is hence given as
Table 7. Regression coefficients of torsional strength of fibre concrete
Where Tc180 is the torsional strength at the 180th day of curing.
further prove significant contribution of corn husk ash on the toughness of fibre concrete at 120 days of curing. Thus, it is found from that, corn husk ash explains about 63% of the variations in the toughness of concrete (R2 = 0.634) with coconut fibre excluded from the model due to insignificant contribution to the predicting model (t = 0.02, p > 0.05). That is, at one percent increment of corn husk ash in the concrete, the toughness will increase at 3.085 unit (t = 5.428, p < 0.01). The predicting model is given as
Table 8. Regression coefficients of toughness at 180 days of curing
Where To180 is the toughness at the 180th day of curing.
4. Conclusion
The addition of coconut fibre to concrete improved the toughness, torsion and to some extent the tensile strength of the concrete at the standard curing age of 28 days. The increase in toughness, torsion and the tensile strength could be attributed to the fact that, the fibre presence in the concrete contributed greatly in offering restraint to early twist or strain in the concrete. It is also clear that the coconut fibres suffered no harm in the alkaline pore water in the concrete due to the presence of CHA polymer, hence, much energy is needed to de-bond and stretch the fibres even at later age, and hence, higher concrete toughness on the 180th day of testing. The corn husk ash in the mix contributed significantly to the toughness of fibre concrete as well as the compressive strength. The corn husk ash was found to compensate the strength loss due to the presence of pores caused by the coconut fibre in the concrete. The introduction of coconut fibre in concrete for construction is therefore recommended with the addition of corn husk ash polymer.
Authors’ contribution
All authors contributed to the paper equally. All authors read and approved the final manuscript.
Authors’ Information
Authors’ current positions: P.P.K.Y. (Senior Lecturer, Construction and Wood Technology Department); E.A.K. (Senior Lecturer, Construction and Wood Technology Department) and A.S. (Tutor, Building and Construction Department, Kumasi Technical Institute).
Acknowledgements
The authors appreciate the contribution and support of the technicians at the laboratories of Construction and Wood Technology Department, Akenten Appiah-Menka University of Skills Training and Entrepreneurial Development, Kumasi Campus.
Disclosure statement
The authors declare that they have no competing interests.
Availability of data and materials
The datasets used and/or analysed during the current study are available from the corresponding author on reasonable request.
Additional information
Funding
Notes on contributors

Emmanuel Appiah-Kubi
Dr. Emmanuel Appiah-Kubi is a Senior Lecturer at the Department of Construction and Wood Technology at the Akenten Appiah-Menka University for Skills Training and Entrepreneurial Development, Kumasi, Ghana. He is a member of the Ghana Institution of Engineering (GhIE) and a Professional Engineer. He has experience in concrete technology and has designed several concrete and reinforced concrete structures, design and construction of timber and bamboo structures. He conducts research into properties of construction materials including concrete, laterite, wood, bamboo and other wood-based materials for efficient utilization. Together with a team he is working on utilizing agricultural residues and local materials in composite building materials to reduce the cost of housing in Ghana. He is a member of ISO Technical Committee - ISO/TC 165 for Timber Structures as well as an expert for INBAR and belongs to INBAR Task Forces for Bamboo Construction and Rattan.
References
- Ahenkora, K., Twumasi, A. S., Sallah, P. Y. K., & Obeng-Antwi, K. (1999). Protein nutritional quality and consumer acceptability of tropical Ghanaian quality protein maize. Food and Nutrition Bulletin, 20(3), 354–16. https://doi.org/10.1177/156482659902000313
- Al-Oraimi, S. K., & Seibi, A. C. (1995). Mechanical characterisation and impact behaviour of concrete reinforced with natural fibres. Composite Structures, 32(1–4), 165–171. https://doi.org/10.1016/0263-8223(95)00043-7
- BS EN 1008. (2002). Mixing water for concrete. Specification for sampling, testing and assessing the suitability of water, including water recovered from processes in the concrete industry, as mixing water for concrete. British Standard Institution.
- BS EN 12390-3. (2019). Testing hardened concrete. Compressive strength of test specimens. British Standard Institution.
- BS EN 12390-6. (2009). Testing hardened concrete. Tensile splitting strength of test specimens. British Standard Institution.
- BS EN 12620. (2002). Aggregates for concrete. British Standard Institution.
- BS EN 197-1. (2011). Cement. Composition, specifications and conformity criteria for common cements. British Standard Institution.
- BS EN 206. (2013). Concrete. Specification, performance, production and conformity. British Standard Institution.
- BS EN 450-1. (2012). Fly ash for concrete. Definition, specifications and conformity criteria. British Standard Institution.
- CoDyre, L., Mak, K., & Fam, A. (2018). Flexural and axial behaviour of sandwich panels with bio-based flax fibre-reinforced polymer skins and various foam core densities. Journal of Sandwich Structures and Materials, 20(5), 595–616. https://doi.org/10.1177/1099636216667658
- Dembovska, L., Bajare, D., Pundiene, I., & Vitola, L. (2017). Effect of pozzolanic additives on the strength development of high performance concrete. Procedia Engineering, 172(2017), 202–210. https://doi.org/10.1016/j.proeng.2017.02.050
- Gutierrezi, R. M., Diaz, N., & Delyasto, S. (2005). Effect of pozzolans on the performance of fiber-reinforced mortars. Cement and Concrete Composites, 27(5), 593–598. https://doi.org/10.1016/j.cemconcomp.2004.09.010
- Halm, M., Amoa-Awua, W. K., & Jakobsen, M. (2004). Kenkey: An African fermented Maize product. In Y. H. Hui, L. Meunier-Goddik, J. Josephsen, W. Nip, P. S. Stanfield, & F. Toldra (Eds.), Handbook of food and beverage fermentation technology (pp. 719–816). Marcel Dekker Incorporated.
- Hejazi, S. M., Sheikhzadeh, M., Abtahi, S. M., & Zadhoush, A. (2012). A simple review of soil reinforcement by using natural and synthetic fibers. Construction and Building Materials, 30(2012), 100–116. https://doi.org/10.1016/j.conbuildmat.2011.11.045
- Ku, H., Wang, H., Pattarachaiyakoop, N., & Trada, M. (2011). A review of the tensile properties of natural fiber reinforced polymer composites. Composites Part B: Engineering, 42(4), 856–873. https://doi.org/10.1016/j.compositesb.2011.01.010
- Momoh, E. O., & Osofero, A. I. (2020). Recent developments in the application of oil palm fibers in cement composites. Frontiers of Structural and Civil Engineering, 14(1), 94–108. https://doi.org/10.1007/s11709-019-0576-9
- Nanayakkaza, N. H., Ismail, M. G. M. U., & Wijesundara, R. L. C. (2005). Characterisation and determination of properties of Sri Lanka coconut. Journal of Natural Fibres, 2(1), 69–81. https://doi.org/10.1300/J395v02n01_06
- Ramakrishna, G., & Sundara, T. (2005). Study into the durability of natural fibres and the effect of corroded fibres on the strength of mortar. Cement and Concrete Composite, 27(5), 575–582. https://doi.org/10.1016/j.cemconcomp.2004.09.008
- Romildo, D., Toledo, F., Karen, S., England, G. L., & Ghavami, K. (2000). Durability of alkali-sensitive sisal and coconut fibres in cement mortar composite. Cement and Concrete Composite, 22(2), 127–143. https://doi.org/10.1016/S0958-9465(99)00039-6
- Yalley, P. P., & Kwan, A. S. K. (2009). Use of coconut fibre as an enhancement of concrete. Journal of Engineering and Technology, 3, 54–73. https://orca.cardiff.ac.uk/43403/1/Yalley%20Kwan%20KNUST%20paper%20.%201.pdf
- Yalley, P. P. K., & Asiedu, E. (2013). Enhancing the properties of soil bricks by stabilizing with Corn Husk Ash. Civil and Environmental Research, 3(11), 43–52. https://www.iiste.org/Journals/index.php/CER/article/view/8116
- Zhang, P., & Li, Q. (2013). Effect of polypropylene fiber on durability of concrete composite containing fly ash and silica fume. Composites Part B: Engineering, 45(1), 1587–1594. https://doi.org/10.1016/j.compositesb.2012.10.006
- Zhou, H., Jia, B., Huang, H., & Mou, Y. (2020). Experimental study on basic mechanical properties of basalt fiber reinforced concrete. Materials, 13(6), 1362. https://doi.org/10.3390/ma13061362