Abstract
The use of recycled aggregates concrete (RAC) contributes effectively to reduce CO2 emissions from concrete manufacturing process while also protecting natural resources by utilizing existing available concrete as an aggregates source for a new one. Studies on the behaviour of RAC have revealed negative effects on concrete strength and microstructure development, resulting in deterioration of mechanical and durability properties. As a result, numerous practical studies have been implemented to enhance the RAC properties using various treatment techniques such as chemical, physical and heating treatments. However, most of these techniques are ineffective compared to conventional concrete applications due to poor mechanical performance of RAC, insufficient environmental requirements, and prolonged treatment times. Recently, the use of nanomaterials has been given significant concern in RAC research. Their nano-sized particles can help to reduce micropores formation by acting as a filling agent to produce a high-density microstructure, thereby enhancing the mechanical properties and durability of RAC. This had led to a wide range of studies being published on improving RAC properties by using nanomaterials. However, relatively few literatures had been conducted on the effects of different types of nanomaterials on the performance of RAC exposed to various types of loads and various external environmental impacts. Besides, the conditions used by authors in these literatures limit comparisons, and in some cases contradictory findings are observed. Thus, this paper aims to bridge the knowledge gap between researchers. This would allow the potential of nanotechnology in innovations to be applied in appropriate areas of RAC applications to benefit the general public good. This paper aims to provide a critical review and comprehensive conclusions on the performance of nano-modified RAC under external loads, environmental impacts and other various conditions. The effects of nanomaterials on the compressive, tensile, and flexural strength of RAC are discussed. The nanomaterials considered are nano-SiO2, nano-CaCO3, nano-TiO2, nano-Clay, nano-Al2O3, and nano-Carbon. Durability characteristics including water absorption, chloride penetration, fire exposure, abrasion resistance, acid and carbonation diffusions are extensively discussed. Microstructure characteristics using SEM, XRD, EDS, and micro-hardness of nano-modified RAC are addressed as well.
1. Introduction
Construction and Demolition (C&D) concrete are regarded as an essential and environmental challenge in the concrete enterprise. In 2020, 70% of the C&D concrete were planned to be recycled and used again, with the hope that concrete recycling will minimize CO2 emissions resulting from concrete manufacturing process and will reduce pressures at landfills (Feng, Wang, et al., Citation2022). In Europe, USA, and Brazil, aggregates demand for concrete production is 2.7 billion, 900 million, and 700 million tons per year, respectively. In conjunction with the increase of C&D waste, which accounts for nearly 36% of all wastes generated on Earth (S. Liu et al., Citation2021). Consequently, inadequate and irregular C&D disposal is a concern in many nations and has tremendous environmental and human consequences. Despite the different and numerous categories of C&D resulting from the demolished concrete, this procedure can produce a material known as recycled concrete aggregates (RCA). The use of RCA as a replacement for natural aggregates (NA) can help sustainable development by providing an environmentally beneficial answer to the lack of raw resources and the rising demand for C&D landfill space. For example, RCA represents 6–8% of total aggregates in Europe, and 0.5 million tons in Australia. In the US, the volume of RCA has increased gradually from 20 million tons in 1980 to 71 million tons in 2007 annually (Aslani et al., Citation2018). National Ready-mix Concrete Association (NRMCA) recommends using RCA up to 10% as virgin aggregates; research suggests using up to 20% in structural applications (Silva et al., Citation2019). German standards allow RCA to make up to 45% of the total aggregate based on concrete’s classification (De Brito et al., Citation2019). Thus, by selecting the source and type of RCA in the mixtures with care, it is possible to develop the structural behaviour of the concrete elements and achieve results comparable to those obtained with conventional aggregates (Zhou et al., Citation2021). Generally, the practices on utilizing RCA have been conducted in many investigations to check the validity of using RCA as alternative materials in structural concrete member (Ossa et al., Citation2016). Tayeh et al. (Tayeh et al., Citation2020) conducted a critical review on utilizing RCA in high-performance concrete. It was reported that using RCA increased overall performance of concrete within certain limits of RCA replacement ratios and seemed to be promising compared to natural aggregates concrete (NAC).
However, a large number of past studies have demonstrated the negative effects of RCA on concrete behaviour compared to concrete of NA. The negative behaviour of recycled aggregate concrete (RAC) manufactured with RCA has been ascribed to the poor properties of waste aggregates with attached mortar on the surface around RCA (Shaban et al., Citation2019). This weak mortar produces undesirable outcomes in RAC mixtures, such as higher water absorption and porosity (Agarwal et al., Citation2019; Kou & Poon, Citation2013), lower strength properties (L. J. Li et al., Citation2016), and less resistance to external environmental factors (De Brito et al., Citation2016) by comparison to blends made of NA. Besides, the attached mortar creates a new “interfacial transition zone (ITZ)” in the areas between old and new cement paste matrix. This ITZ is responsible for the hardened properties and durability of the structure, meaning that the weaker the ITZ, the weaker the performance of RAC blends (Mukharjee & Barai, Citation2014a). Figures shows the constitutive phases and scanning electron microscope (SEM) of ITZ within the RAC blends (Z. Liu et al., Citation2016). These effects lead to decrease in compressive strength of 5% to 55%, and reduction in tensile strength of 8% to 35% (Ali & Qureshi, Citation2019; Arora et al., Citation2019; Ismail et al., Citation2017; L. J. Li et al., Citation2016; Pedro et al., Citation2017; Sadati & Khayat, Citation2016; J. Thomas et al., Citation2018). The decline of strength is mainly due to the existence of porous mortar causing permeability of RAC and developing a risk towards the concrete degradation (Rizvi et al., Citation2010).
Figure 1. Constitutive phases & SEM image of RAC (Z. Liu et al., Citation2016).
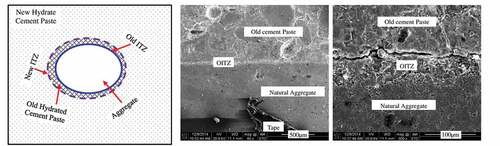
Due to the aforementioned drawbacks, several techniques have been developed to improve the overall performance of RAC structures. Removal of attached mortar, strengthening of weak mortar, and modification of mixing process are considered as the most common techniques to improve the quality of RAC mixtures (Ouyang et al., Citation2020). However, these treatments have not provided satisfactory solutions for RAC performance. For example, acid treatment to remove adhered cement is not suitable to meet environmental requirements (Tam et al., Citation2007), and polymer dispersant to strengthen mortar is expensive and uncommon in practice (Kou & Poon, Citation2010). Also, modifying mixing processes to improve the mechanical properties of RAC consumes time to complete (Tam & Tam, Citation2008). In addition, Kou and Poon (Kou & Poon, Citation2012) and Li et al. (J. Li et al., Citation2009) checked the use of pozzolanic powders (silica fume, fly ash, and blast-furnace slag) to strengthen the mortar-adhered RCA. Despite a slight improvement in mechanical properties of RAC, carbon depths and water absorptions were higher as compared to the conventional concrete. Zhan et al. (Zhan et al., Citation2019) studied the effects of carbonation treatment to enhance the properties of attached mortar in RCA. An acceptable reduction in water absorption and chloride diffusions were found, however, this technique required a safety precaution during chemicals handling by following the required washing with a huge amount of freshwater to remove these chemical pollutants. Heating treatment provides excellent adequacy in decreasing the water absorption of RCA, resulting in higher stiffness and greater dynamic modulus (Santha Kumar et al., Citation2019). However, when applying this process, the mechanical properties of RCA have occasionally degraded at elevated temperatures due to the mineral changes in the attached mortar, aggregates, and ITZ interfaces, eventually leading to the development of thermal cracking in RAC specimens (Zhao et al., Citation2017). Although these modification techniques have performed adequately in some cases, they are all prone to unsatisfactory solutions.
Nano concrete is a type of concrete that incorporates nanomaterials or that contains nanomaterials with a particle size of less than 500 nm (Hou et al., Citation2015). It was previously believed that incorporating nanoparticles into concrete would increase the behaviour of conventional concrete. Nanoparticles improve the bulk characteristics of concrete, and can provide an excellent filler effect by improving the cement’s intersectional zone and resulting in a higher density concrete (Pacheco-Torgal & Jalali, Citation2011). Allujami et al. (Allujami et al., Citation2021) carried out a comprehensive study on the incorporation of nanomaterials to conventional concrete pavement. It was demonstrated that adding different types of nanomaterials contributed to improve the hardened properties of concrete. Compressive, tensile, flexural, and dynamic strengths were significantly increased at several rates, mainly dependent on the type and concentration of nanoparticles added. The optimal nano content was reviewed as well.
Recently, researchers have employed nanotechnology to improve the RAC qualities, due to the ability of nanoparticles to fill the pores and compensate for the unstable performance induced by the addition of RCA to concrete mixes compared to other traditional filling materials. Besides, the excellent stability, high activity, and cost-effectiveness of NPS promote its role in fulfilling the criteria for sustainable buildings (Dawood & Mahmood, Citation2021). Thus, the implementation of nanomaterials has become an urgent need to produce ultra-high-performance mixes (C. Liu et al., Citation2020), owing to the NPS’ ability to provide higher compressive and tensile strengths of conventional concrete mixtures (Camiletti et al., Citation2013; Mohammed & Adamu, Citation2018; Staub de Melo & Trichês, Citation2018), higher flexural strength (Adamu, Mohammed, Shafiq, Shahir Liew, et al., Citation2018; J. Liu et al., Citation2018), higher dynamic strength (Su et al., Citation2016), and better durability against environmental effects (Adamu, Mohammed, Shafiq, Liew, et al., Citation2018; Ali Shafabakhsh et al., Citation2020).
Despite several studies on the effect of nanomaterials on RAC structures, few existing review papers have failed to address the effects of various types of nanoparticles when introduced into RAC mixtures and have not taken all other aspects of nanomaterials influence on RAC blends (Zheng et al., Citation2021). Therefore, achieving a critical review to include all aspects of nano effect on the RAC applications is becoming nowadays an urgent necessity. This review paper covers the following gaps that not previously addressed: 1) A thorough comparison of studies on the influence of nanoparticles addition on the workability of fresh RAC mixes; 2) A critical comparative analysis between studies on the effect of introducing different nanomaterials to hardened RAC mixes at various loadings; 3) A comprehensive study on the effect of adding nanoparticles on the durability of RAC structures; 4) A careful review of the combined effect between nanoparticles and supplementary cementitious materials when added together into RAC mixes; 5) A comprehensive analysis of RAC microstructure due to the nanomaterial’s modification using the most common techniques.
In this paper, nano-SiO2, nano-CaCO3, nano-Al2O3, nano-TiO2, nano-Clay, and nano-Carbon are focused. Furthermore, challenges related to nanotechnology use in RAC are identified, and research recommendations and conclusions are provided. The present study aims to provide a more comprehensive review on the use of nanotechnology in the RAC applications. As a result, this review aims to expand the usage of RCA materials in the concrete structures industry in order to satisfy sustainability goals and conserve the natural resources through the application of innovative nanotechnology techniques.
2. Properties of nanomaterials
A nanomaterial is a material with at least one dimension in 3-D space or whose composition has been reduced to a nanoscale size (1–100 nanometer (nm)) (Wu et al., Citation2014). Its superior performance comes not only from being ultra-fine particles size but also from its high specific surface area of up to ∼750 m2/g (Han et al., Citation2019; Haruehansapong et al., Citation2017), and high aspect ratio of up to 132,000,000:1 (Abu Al-Rub et al., Citation2012). Li et al. (Li, Xuan, Chu, et al., Citation2021) discovered that the size of SiO2 nanoparticles had no significant effect on strength of concrete mix. Using a similar approach, larger nanoparticles with sizes up to 106 nm demonstrated higher mechanical performance and durability but were less successful in workability when compared to smaller size (i.e., 12.2 nm) (Li, Xuan, Sojobi, et al., Citation2021). Nanomaterials are classified according to their chemical composition and manufacturing process. Silica direct synthesis and quartz crystallization are the well-characterized techniques in nano-silica morphology (Gorbatsevich et al., Citation2017), while calcite, periclase, and chloraluminite are the basic components for nano-calcium carbonate synthesis (Hussein et al., Citation2020). Nano-titanium is a titania oxide synthesized using hydrothermal and microwave techniques from ilmenite, rutile, and anatase (Ziental et al., Citation2020). Nano-clay and nano-carbon are from chemical weathering of aluminum silicate minerals and thermal-chemical vapour deposition of hydrocarbon gas, respectively (Niroumand et al., Citation2013; Scott, Citation2017). In addition, via utilizing nano-sized microscopy, nanomaterials can be available as amorphous, hexagonal, spherical, cubic, needle-shaped, cylindrical shapes (Gorbatsevich et al., Citation2017; Liao & Liao, Citation2007; Mansor et al., Citation2018). The solubility of nanoparticles powder in aqueous water is a critical factor affecting the performance of nanocomposites. Due to the inert molecule, hydrophobic, and van der wall characteristics of nanoparticles, they are insoluble in aqueous media, producing the so-called sedimentation phenomenon. Therefore, need specific kinds of catalysts to ensure uniform dispersion of nanomaterials inside composites (Carriço et al., Citation2018; Gorbatsevich et al., Citation2017; Malešič et al., Citation2019). So, current research has focused on developing liquid-solid nanoparticles. It was revealed that colloidal nano-SiO2 ensured homogeneous dispersion of nanoparticles and prevented agglomeration, resulting in an enhancement in concrete strength compared to nano powder (Erdem et al., Citation2018). Moreover, colloidal nanoparticles increased cement hydration in the early curing days by reducing low-stiffness calcium-silicate-hydrates (C-S-H) gel and increasing high-stiffness gel (Mukharjee & Barai, Citation2015). The microstructure of nanomaterials is determined using scanning and transmission electron microscopy, confirming their morphology (Abu Al-Rub et al., Citation2012; Niroumand et al., Citation2013; Ziental et al., Citation2020). In addition, X-ray diffraction measures the intensity of nanomaterials, showing a single broad peak for nano-silica (Gorbatsevich et al., Citation2017), two diffraction peaks for nano-titanium and nano-carbon (Chenari et al., Citation2016; Habeb Abdulrazzak et al., Citation2019), and more peaks for nano-calcium carbonate (Shafiu Kamba et al., Citation2013). The higher the number of peaks, the more crystalline the nature. High-resolution imaging using energy dispersive X-ray spectroscopy is an important key to measure the qualitative elemental composition of nanomaterials. A 99% of purity silicon and 1% of impurity elements are the main components in nano-silica (Gorbatsevich et al., Citation2017), while higher limestone and low silicon concentrations are clearly shown in nano-calcium carbonate (Hussein et al., Citation2020). The energy X-ray analysis has demonstrated the presence of carbon, silicon, aluminum, and oxygen as prominent elements of nano-clay; a high percentage of carbon and oxygen atoms on nano-carbon surfaces (Abu Al-Rub et al., Citation2012). The thermal behaviour is also an important factor to determine the heat conductivity of nanomaterials, due to the possibility of internal component changes at elevated temperatures.
3. Nanomaterials on fresh RAC
The behaviour of RAC in its fresh state can provide insight into how it can perform in the hardened state. Workability properties of RAC are essential when specifying the requirements of a ready-mix concrete plant in its fresh condition. In terms of nanomaterials, adding nanoparticles to concrete mixes has shown to reduce its workability owing to the increased powder surface area and ultrafine particle size of nanoparticles, resulting in a decrease of free water available within wet blends (Allujami et al., Citation2021). For fresh RAC, the inclusion of nanoparticles has been studied to check workability of fresh properties of concrete mixes. For example, Wang et al. (Y. Wang et al., Citation2019) noticed that the addition of nano-silica decreased the workability of RAC due to the nano-sized particles and ultra-high specific surface area of silica, resulting in easily agglomeration under the van der Waals force (Li et al., Citation2017). When nano-silica was increased up to 8%, the slump values showed an additional decrease of 35%, compared to the 15% reduction in the mixes with a 3% content of nano. Similar findings were reached by Mukharjee and Barai (Mukharjee & Barai, Citation2015) and Hosseini et al. (Hosseini et al., Citation2011), stating that substituting cement with nano-SiO2 at 1.5% and 3% resulted in reduction workability by 28% and 50%, respectively, compared to conventional specimens. Moreover, Gao et al. (Gao et al., Citation2020) found that incorporating silica nanoparticles at the dosage of 1% and 2% decreased the workability of RAC by 32% to 59%, respectively.
In addition, ultra-sonication process resulted in a higher reduction in the slump rates. Erdem et al. (Erdem et al., Citation2018) investigated the influence of colloidal nano-silica on the workability of concrete made of 100% RCA with 1% and 1.5% nano-silica. The test revealed a declining trend of slump when the nano-silica slurry was added to the RAC mixes. Additionally, Nuaklong et al. (Nuaklong et al., Citation2020) examined the integration of two different kinds of nanoparticles (nano-silica and nano-rice husk ash) into RAC geopolymer blends. Their results indicated a significant reduction in flowability of silica-modified RAC by 16% as compared to a 10% decrease in rice husk ash mixes.
Another measurement of nano-modified RAC’s workability is called vebe time test. By this inspection, freshly concrete properties are determined via the time reqiured for full remoulding and compactibility. Based on this, Tavakoli et al. (Tavakoli et al., Citation2020) examined the effect of SiO2 nanoparticles on the workability of roller-compacted concrete pavement containing 40% RCA replacement and 0.7% nanoparticle dosage. The nano-modified RAC was found to have low workability, in which the vebe time was increased by approximately 35% compared to traditional RAC.
On the other hand, Shaikh et al. (F. Shaikh et al., Citation2018) measured the slump of fresh RAC caused by nano-silica modification by applying two methods of mixing (direct mixing and pre-soaking methods). Unexpectedly, the workability of mixtures was increased after incorporation of nano-silica, and the direct mix indicted a higher slump than the pre-soaking method. Similar findings were agreed by Zhang et al. (Zhang et al., Citation2016), showing that pre-soaking RCA in nano-SiO2 slurries increased workability by 16% compared to untreated RCA. Younis and Mustafa (Younis & Mustafa, Citation2018) pointed out that all nano-modified RAC exhibited higher slumps than the mix design’s target value. Moreover, the combined effect of nano-SiO2 and nano-CaCO3 slurries showed higher workability of wet RAC (Meng et al., Citation2020).
Aside using nano-silica in RAC, Muduli and Mukharjee (Muduli & Mukharjee, Citation2019) accomplished a slump tests to evaluate the fresh properties of RAC before and after modification with nano-metakaolin. The inclusion of nano-metakaolin was found to reduce slump values of RAC due to the higher surface area of nano-sized metakaolin. This is due to absorption of a portion of free water, and the final slump was found to be 12% lower compared to free-nano RAC mixtures. However, as the RCA replacement developed, so did the workability. This trend was attributed to the availability of free water added during concrete mix design in order to compensate the high water need of RCA.
Furthermore, it has been determined that adding multi-walled nanotubes (MWCNTS) to RAC blends resulted in a significantly lower slump compared to conventional RAC at an approximate rate of 57%, as well as MWCNTS-treated RAC showed lower workability than fresh mixes treated with nano-SiO2 and nano-CaCO3 (Gao et al., Citation2020).
Indeed, it can be shown that adding nanomaterials reduces the workability of fresh RAC mixes and that increasing the cement replacement quantities achieve further reduction. This is mostly due to the nanoparticles’ small size and high-specific surface area, which need a large amount of water to uniformly disperse inside wet mixes. However, increasing the workability after nano addition, as shown in a limited number of studies, is due to the proper RCA surface treatment prior to mixing. Moreover, employing certain types of superplasticizers can be a viable solution for ensuring the workability of nano-modified RAC (Alhawat & Ashour, Citation2020). Finally, more research into the role of nanoparticles on fresh RCA concrete is required, in particular, its role on the development of fresh density and setting times of RAC.
4. Nanomaterials on hardened RAC
Hardened properties of concrete are one of the major interests for practical application of RAC structures. Studies have shown that the concrete strength of RAC is much lower than that of NA’ blends. However, the demand for RCA materials to conserve natural resources and minimize environmental effects has grown critical in the concrete industry at the same time. Thus, improvement of the hardened properties of RAC is considered directly responsible by way of the nanomaterials (Salkhordeh et al., Citation2011). The performance of RAC is primarily determined by the nature and proportions of NPS, source of RCA, and type of supplementary cementing additives in the blends (Salkhordeh et al., Citation2011; Yue et al., Citation2020). These studies have established that incorporating NPS could modify the hardened characteristics of RAC, as nanoparticles act as a 1) Nanofiller via filling micro-cracks and pores much superior to common filler materials. 2) Nano-reinforcement by enhancing the strength and consistence of paste. 3) Nucleation sites due to higher surface area, and 4) Highly reactive pozzolanas that consumes calcium hydroxide Ca(OH)2 to generate additional calcium silicate hydrates (C-S-H) (P. Zhang et al., Citation2017). The hardened properties of RAC considered in this section are compressive, tensile, and flexural strengths, as well as the impact resistance. Table lists the summery of existing studies about hardened properties of nano-modified RAC.
Table 1. Hardened properties of nano-modified RAC; N/A: not applicable
4.1. Compressive strength
The compressive strength test is the most significant measurement to determine the properties of RAC, as it provides an idea about the quality of the concrete structure. The next sections discuss the impact of various kinds of nanomaterials on RAC’s compression strength. Nano-SiO2, Nano-Clay, Nano-CaCO3, Nano-TiO2, and Nano-Carbon are presented.
4.1.1. Nano-silica (SiO2)
Nano-silica is a nano-sized powder composed of silicon dioxide (SiO2) microspheres with diameters of 100 nm or less (Haruehansapong et al., Citation2017). Its effect on RAC mixes has been more prominent than that on conventional mixes due to its substantial role in densifying ITZ areas, improving bond strength, and delaying development of RCA-induced cracks (Yue et al., Citation2020). In this, Shaikh et al. (F. Shaikh et al., Citation2018) showed a 40% increase in compressive strength at a higher replacement of RCA compared to conventional specimens. Sadeghi-Nik et al. (Sadeghi-Nik et al., Citation2019) discovered that incorporating 2% of nano-silica improved compressive strength by 35%—75% depending on the replacement ratios of RCA. Similar results were observed by Nuaklong et al. (Nuaklong et al., Citation2018) and Meng et al (Meng et al., Citation2020), where the compressive strength of RAC containing 100% RCA was increased by 30% due to the incorporating of 2% and 5% NPS, respectively. Several of these studies revealed that the optimum concentration of SiO2 nanoparticles should not exceed 2% (Li et al., Citation2017; Murthi et al., Citation2020; Vasanthi & Senthil Selvan, Citation2020; Ying et al., Citation2017; Yue et al., Citation2020). Others recommended that the proportion should not exceed 1.5% (Erdem et al., Citation2018). A few research stated that 3% of nano-silica exhibited the greatest compressive strength (Agarwal et al., Citation2019). Yonggui et al. (Yonggui et al., Citation2020) pointed out that the optimum nano-SiO2 concentration in fiber reinforced RAC was 6% for low RCA replacement ratios, but 3% for higher RCA content.
The addition of supplemental cementing materials (SCMS) to concrete mixtures including nanoparticles has historically been a common method for enhancing the mechanical performance of the concrete mixture. Feng et al. (Feng, Tang, et al., Citation2022) conducted an experiment to investigate the influence of adding fly ash and nano-SiO2 on the strength of RAC containing 50% and 100% RCA. Fly ash replaced 10% and 20% of cement, while nanoparticles were added at 1%, 2%, and 3%. The combination of 10% fly ash and 3% nano-SiO2 was advised since it showed acceptable pozzolanic effects and could perform a supplementary hydration process with CH to boost the formation of C-S-H gels at the early and late curing ages, respectively. Similar investigation was carried out by Yunchao et al. (Yunchao et al., Citation2021), except instead of fly ash, silica fume was supplemented in various amounts (i.e., 3%, 6%, and 9%). The results showed that the compressive strength at 3, 7, 28 days reached the maximum when 6% silica fume and 3% nano-SiO2 were utilized.
Nano-silica can be used in combination with physical technology, which may reach an amazing effect in modifying the RAC. Recently, physical technology named compression casting is adopted together with nano-silica to modify the RAC. Liang et al. (Liang et al., Citation2022) took an alternative strategy, combining compression casting technology with the addition of nano-silica at a 2% dose to improve the RAC combination. The results demonstrated a considerable increase in compressive strength of 143% compared to 88% using the compression casting process alone. Silica nanoparticles have been shown to effectively improve the compressive strength of RAC, due to its exceptional role in refining the ITZ between old and new cement mortar paste via producing extra C-S-H gels that responsible for improving the compressive strength of RAC (Murthi et al., Citation2020).
In addition, there have been some hybrid use of nanomaterials to improve the compressive strength of concrete. For example, Yue et al. (Yue et al., Citation2020) discovered that adding micro-CaCO3 at 1% to nano-silica-modified RAC mixes had an additional 15% increase in 90-day compressive strength above the strength gained by adding only nano-SiO2 alone. In this scenario, 2% nano-SiO2 was superior to other doses. The combined effect of nano-silica and micro-CaCO3 not only encouraged the multilayer hydration of cementitious materials, but also enhanced the particle dense packing of binders. Similarly, pre-soaking of RCA in a slurry of nano-SiO2 and nano-CaCO3 by Zhang et al. (Zhang et al., Citation2016) increased the compressive strength by about 25%.
4.1.2. Nano-clay (NCL)
Nanoclays are nanoparticles of layered mineral silicates. It has been classified into several types such as kaolinite, montmorillonite and halloysite (Uddin, Citation2008). The utilization of clay nanoparticles in the RAC mixes has been carried out in several researches due to its main role to act as nanofillers and highly reactive pozzolanas (Mirgozar Langaroudi & Mohammadi, Citation2018), that produces RAC with higher compressive strength. Toghroli et al. (Toghroli et al., Citation2020) found that replacing cement with 1% and 2% nano-clay (NCL) and 10% silica fume enhanced the compressive strength of RAC. The 1% replacement of NCL and 10% replacement of RCA were the optimum ratios, with a 7% improvement in maximum compression capacity as compared to mixes with NA. However, at higher replacement of NCL up to 3% by cement weight, no significant improvement was noticed due to the poor dispersion, irregular distribution, and remarkable agglomeration of clay nanoparticles inside the RAC.
Metakaolin is a pozzolan form of kaolinite minerals that is broadly applied in mortar and concrete to strengthen their properties (Ragab, Citation2019). The effect of nano-metakaolin (NMK) in RAC studied by Muduli and Mukharjee (Muduli & Mukharjee, Citation2019) showed that higher content of NMK produced better performance and higher compressive strength at all RCA replacement proportions. The optimum replacement ratio was observed to be 15% of cement mass. Xie et al. (Xie et al., Citation2020), on the other hand, proved that the highest compressive strength of RAC was achieved when the cement was substituted with a lower dosage of metakaolin nanoparticles (5%), where the compressive strength was raised by 14.6% above control samples. These studied have shown that NCL effectively increases the compressive strength of RAC.
4.1.3. Nano-CaCO3 (NCC)
Nano-calcium carbonate (NCC), also called limestone nanoparticles, is an ultra-fine powder of CaCO3 with a mean grain size of fewer than 100 nanometers. Its implementation in concrete mixtures has been extensively researched due to its role as a superior filler in concrete blends (Meng et al., Citation2017). The role of limestone nanoparticles in the RAC is not different from that in conventional concrete. According to Li et al. (Li et al., Citation2017), adding of NCC had no significant effect on RAC. However, at an early age, the compressive strength was enhanced by up to 21% and 7% for mixes containing 1% and 2% nanoparticles, respectively. In contrast, Gao et al. (Gao et al., Citation2020) discovered that the optimum substitution of limestone nanoparticles was 2%, and the ultimate compressive strength of RAC was increased to 36.6 Mpa compared to 30.5 Mpa in mixes containing 1% of nanoparticles. Furthermore, it has been concluded that the modification of nanoparticles, when added to RAC mixtures, could result in an additional enhancement. The ultrasonication process to disperse limestone nanoparticles indicated a better performance than the surfactant technique (Kawashima et al., Citation2014). Nonetheless, these two skills afforded a better uniform dispersion of lime nanoparticles within the RAC and contributed to promoting the filling effect of nanograins within concrete blends. Some studies have sought to use nanomaterials as nano-slurries instead of directly mixing with concrete components. Accordingly, Meng et al. (Meng et al., Citation2020) inspected the compression behaviour of fly ash RAC, reinforced with 10 mm of steel reinforcement and treated with nano-CaCO3 slurries. The results indicated a significant enhancement in compressive strength two times compared to normal RAC mixtures. However, more attention should be taken when applying this method, as the pre-soaking technique of nanoparticles could result in a decrease in the workability of RAC structures (F. Shaikh et al., Citation2018).
4.1.4. Nano-TiO2 (NT)
Nano-titanium (NT) is a famous type of nanomaterial that is widely used as photocatalytic material in concrete pavements. TiO2 nanoparticles can effectively eliminate harmful gases in the air without harming or polluting the pavement surface (Staub de Melo & Trichês, Citation2018). The primary application of nano-TiO2 is to improve the early strength of concrete, allowing the pavement to be opened to traffic earlier and thus allowing the paving investment to begin sooner. In the case of RCA mixes, researchers have been drawn to the use of nano-TiO2 due to its exceptional ability to increase the compressive strength of blends (Hassan et al., Citation2012) and decrease overall porosity by creating denser RAC mixes (Moro et al., Citation2020).
Ying et al. (Ying et al., Citation2017) and Xu et al. (Xu et al., Citation2020) examined the nano-TiO2 effect on the RAC compression behaviour by using different mixing techniques and varying ratios of both RCA and NPS. The former concluded that mixing nanoparticles directly with cement paste containing recycled aggregates improved compressive strength. This enhancement was clearly visible in specimens mixed by 2% nano-TiO2, where the compressive strength exceeded 18% when compared to the reference. Incorporating 3% of NT resulted in adverse and negative behaviour (Ying et al., Citation2017). The latter, on the other hand, employed a different enhanced method to improve the mechanical performance of supplementary RAC pervious pavement. The recycled aggregates were first soaked in the TiO2 solution to obtain the TiO2-soaked RCA. Cement was replaced by fly ash at (5%, 10%, and 20%); natural aggregates were substituted with RCA in a variety of ratios, including 30%, 50%, and 70% that had been presoaked in different concentrations of NT slurries. Their findings demonstrated that the higher compressive strength was reached in the porous pavement when RCA and fly ash were added up to 50% and 5%, respectively with optimized NT concentration at 0.3%. This positive performance was altered to negative when RCA immersed in NT solution at 0.5% concentration, due to the formation of more calcium meteorites, which attempted to reduce the performance of the recycled pervious pavement (Xu et al., Citation2020). Indeed, nano-TiO2 has been shown to increase the packing density of concrete by improving the homogeneity of ITZ zones in concrete blends (Nikbin et al., Citation2019). However, studies on its effect on RAC performance at early ages are still scarce and need further investigations.
4.1.5. Carbon nanotubes (CNTS)
Carbon nanotubes (CNTS) are tubes composed of carbon with nanometer-sized diameters. They can be noticed in two different basic structures; single-walled and multi-walled carbon nanotubes (Alhans et al., Citation2018). CNTS acts as a reinforcing material in cementitious composites, its nucleation and bridging effects aim to enhance the strength properties of the mixtures (Xu et al., Citation2015). However, the impact of CNTS on the performance of RAC structures is still unknown and has been addressed in only a few papers. Gao et al. (Gao et al., Citation2020) looked into the effect of CNTS addition on the RAC strength made of different sources of waste cementitious materials (i.e., 55% of clay bricks aggregates, 45% of RCA and mortar). MWCNTS at a level of 0.1% were added by weight of cement, while the natural aggregates were replaced by RCA at proportions of 50%, 70% and 100%. Their findings showed a notable rise in the 28-day compression strength by approximately 42% over untreated RAC specimens and exceeding that of RAC combined with nano-CaCO3. In a different investigation, Song et al. (Bin Song et al., Citation2021) looked at the influence of incorporating MWCNTS on the interfacial mechanical properties of RAC. For that purpose, MWCNTS was added at a level of 0.05%, 0.1%, 0.2%, 0.5% and 1% by weight of cement. Interfacial shear strength was determined by pouring a 5 mm thick ring of old mortar around a cylinder granite plate located in the middle of a rectangular block, followed by a new mortar ring poured around the outside. It was discovered that incorporating 0.2% MWCNTS indicated the highest shear strength, with a 53% improvement over free-nano mixtures. This was due to nanotubes’ function of densifying the microstructure and providing fewer micro-cracks when compared to conventional RAC and other nano fillers.
To summarize, CNTS is an extremely superior material that significantly improves the compressive strength of RAC structures by producing more solid micro-structures and stronger ITZ zones. In addition, thanks to its nano-size particles, CNTS can significantly improve RAC’s microstructure with the reduction of its permeability by covering the surface of its recycle aggregates, retaining its porosity, speeding up the reaction to cement hydration, and strengthening the bonds between the aggregates and the cement paste (Gao et al., Citation2020). However, the main drawback of employing CNTS within RAC mixtures is that it is tending to agglomerate due to the high Van der Waals’ force among particles, resulting in poor micro-structures and lower strength of blends. Thus, approaches for dispersing and de-agglomeration of the CNTS have been successfully employed. These methods include ultrasonication, magnetic stirring, homogenization, and surfactants (Carriço et al., Citation2018).
4.2. Tensile strength
Evaluating the tensile splitting strength of RAC structures is considered a primary issue, no less important than compressive strength. This is due to the fact that concrete structures are very susceptible to tensile cracking due to different types of forces and applied loads (Bui et al., Citation2018). Tensile behaviour of nano-modified RAC combined with nano-SiO2 has been remarkably similar to that reported in compressive strength, with an enhancement ratio of between 6% and 27% greater than that of conventional RAC blends (Agarwal et al., Citation2019; Gao et al., Citation2020; Younis & Mustafa, Citation2018). Furthermore, it was found that the optimal replacement of silica nanoparticles in the supplementary cementitious materials (SCMS) modified RAC corresponding to higher tensile splitting strength was determined to be 1% for high-volume fly ash (Nuaklong et al., Citation2018), 1% for rice-husk ash (Nuaklong et al., Citation2020), 1.5% for bagasse ash (Murthi et al., Citation2020), and 2% for metakaolin-limestone (Sadeghi-Nik et al., Citation2019). Wang et al. (Y. Wang et al., Citation2019), on the other hand, indicated that the optimal quantity of nano-SiO2 was found to be 3% for blends containing 50% of RCA and 2 kg/m3 of basalt fibers, and increased to 6% for blends containing 100% RCA.
Few literatures have addressed the tensile behaviour of nano-modified RAC mixtures made with other nanomaterials. Muduli and Mukharjee (Muduli & Mukharjee, Citation2019) determined that the effective replacement rate of metakaolin nanoparticles was 15%, such as in the compression scenario. The tensile strength of NMK-modified RAC increased by 18% compared to normal RAC mixed with both 50% and 100% of RCA.
Meng et al. (Meng et al., Citation2020) noted that substituting cement with 5% nano-CaCO3 slurries resulted in no significant enhancement in the tensile behaviour of RAC, and the ultimate strength was very close to that value of the conventional RAC before nano modification. However, Gao et al. (Gao et al., Citation2020) found that incorporating 2% of nano-CaCO3 increased the tensile strength of RAC by 5.6%, and displayed better results than RAC incorporated with 1% nanoparticles. Furthermore, it has been reached that the mixing technique of limestone nanoparticles with RAC played a good role in the tensile performance of RAC. Immersing RCA alone in the nano-CaCO3 surfactants at an early stage led to a slight improvement in tensile strength when compared to other mixing methods (Sasanipour et al., Citation2021).
For comparative purposes, Gao et al. (Gao et al., Citation2020) published another study to measure the tensile strength of RAC by incorporating MWCNTS. MWCNTS were added at a dosage of 0.1% by weight of cement, and natural aggregates were replaced by RCA at 70%. Results indicated an increment in tensile strength by approximately 16% compared to untreated RAC blends. Moreover, the ultrasonication process to disperse carbon nanoparticles exhibited more efficiency than another surfactant method, with a 3% increase in tensile strength between the two methods.
Indeed, the nanoparticles included within the RAC blends can rapidly absorb calcium hydroxide Ca(OH)2 to form C-S-H products, resulting in a decrease in voids induced by the presence of a high number of ITZ areas at different locations in the blends. Therefore, the bond between RCA and mortar becomes strong as in conventional concrete and the old mortar gets denser, as a result, the tensile strength can significantly increase. However, further research should be conducted to evaluate the tensile strength of nano-incorporated RAC and to identify optimization ways when various kinds of nanomaterials are included in RAC blends.
4.3. Flexural strength
Flexural strength of RAC is considered as an important indicator of the stability of concrete structures. The greater the strength of a RAC, the more units can be included in a restoration. As a result of the presence of adhered mortar and porous ITZ between the old and new cement pastes, the addition of RCA in concrete manufacture resulted in a substantial reduction in flexural strength (Sadati & Khayat, Citation2016). As a result, it is becoming necessary to improve the flexural performance of RAC with ultra-fine materials such as nanoparticles. Agarwal et al. (Agarwal et al., Citation2019) estimated the nano-SiO2 influence on the flexural strength of blends made of different proportions of nano-SiO2 i.e., 1%, 3%, and 5% and different replacement of RCA, i.e.,, 20%, 40%, and 60%. According to their findings, the optimal percentages of nano-silica and RCA were 3% and 40%, respectively. Additionally, increasing the replacement ratios of RCA to greater than 50% showed no impact on flexural performance. Mukharjee and Barai (Mukharjee & Barai, Citation2014b) found a reduction of about 10% at higher replacement ratios of RCA. Vasanthi and Selvan (Vasanthi & Senthil Selvan, Citation2020) conducted an experimental study to determine the flexural strength of RAC mixed with 100% of RCA and various amounts of silica nanoparticles (i.e., 1%, 2% and 3%). For this objective, beams were tested at 7, 14 and 28 days. A slight improvement in flexural strength at all ages was observed due to NPS incorporation, and 2% nano-silica was found to be optimal. Wang et al. (X. Wang et al., Citation2020) showed that pre-soaking RCA in various concentrations of nano-SiO2 slurries for different soaking times (24, 48, and 72 hours) had minimal impact on RAC’s flexural strength.
Nuaklong et al. (Nuaklong et al., Citation2018) focused on the impact of nano-silica on bending performance of geopolymer blends consisting of 100% RCA and nano-silica at 1%, 2% and 3%. The results indicated a 20% increase in flexural strength when 1% of silica nanoparticles were included. Yuvaraj and Anish (Yuvaraj & Anish, Citation2019) conducted a similar report to evaluate the flexural strength of beams mixed with 60% RCA. Fly ash and nano-silica were mixed as partial replacements of cement at 60% and 2.5%, respectively. Flexural performance was significantly improved, with flexural strength rising by about 69% when compared to traditional RAC. Krishna et al. (Krishna & Krishna, Citation2017) used metakaolin as a partial substitution for cement in the preparation of RAC specimens at different contents (i.e., 10%, 15%, and 20%), and nano-silica was added at 1%, 2%, and 3%. Their findings showed that replacing 50% of the RCA, 15% of the metakaolin, and 2% of the silica increased flexural strength by 33%. In fact, the strength improvement of RAC prepared with SCMS and nano-silica is due to the particles size of SCMS react with Ca(OH)2 to form additional binder material (Lavergne et al., Citation2019).
On the other hand, investigations into flexural behaviour have been expanded to comprise the inclusion of reinforcement when added to RAC. Wang et al. (Y. Wang et al., Citation2019) inspected the combined effect of nano-silica and basalt fibers on flexural performance of RAC at two RCA replacement ratios (50% and 100%). Nano-SiO2 and basalt fibers were added at a rate of 3%, 6%, and 8%, and 1 kg/m3, 2 kg/m3, and 3 kg/m3, respectively. At 28 days, the four-point flexural strength of prismatic beams were determined. The presence of fibers alone enhanced RAC’s flexural by 1.6% compared to 100% RAC. The flexural strength increased and then decreased with a higher level of basalt fibers up to 2 kg/m3 content. The integration of nanoparticles showed, however, an adverse effect and a similar behaviour to that of conventional RAC. Meng et al. (Meng et al., Citation2020) carried out a laboratory test to evaluate the flexural strength of nano-modified reinforced RAC. The results of RAC beam revealed an unexpected reduction in ultimate moment due to the addition of 5% Nano-SiO2 and 5% Nano-CaCO3 slurries. Consequently, this behaviour should be paid more attention in the future. Jaishankar and Raja (Jaishankar & Karthick Raja, Citation2019) conducted an experiment using recycled concrete mixes including nano-silica and polypropylene fibers. Nanoparticles were added at 0.5%, 1%, 1.5%, and 2%, while polypropylene fibers were added at 2% as reinforcing additives. Their findings showed a 16% increment in flexural strength compared to free-nano RAC.
Researchers have also worked extensively using other types of nanomaterials. For example, Muduli and Mukharjee (Muduli & Mukharjee, Citation2019) pointed out that substituting cement by nano-metakaolin improved bending strength by a maximum of 12.5%. The optimal ratio of metakaolin nanoparticles was discovered to be 15%. Toghroli et al. (Toghroli et al., Citation2020) carried out series of experiments to investigate the flexural response of RAC reinforced with steel and glass fibers, using nano-clay various proportions, at volumetric fractions of 1% and 2%. Mixes were prepared with 10%, 25%, 50%, and 100% RCA, and cement was partially substituted with 1%, 2%, and 3% nano-clay. The ideal content of nanoparticles was determined to be 1% at low replacement ratios of RCA but rose to 3% at high replacement rates of RCA up to 100%. Also, the addition of steel fibers showed better performance compared to glass fibers.
Moro et al. (Moro et al., Citation2020) experimentally probed the effect of TiO2 nanoparticles on the flexural strength of RAC mortar made of 100% RCA. Samples were assessed at 90 days after being exposed to high temperatures, and the flexural strength test was measured according to ASTM standards. Their results revealed that the addition of 1% TiO2 nanoparticles slightly improved flexural strength by 1% compared to traditional RAC. When the temperature was raised to 500°C, the use of nano-TiO2 had a negative impact on resistance in terms of flexural strength.
Sharma and Arora (Sharma & Arora, Citation2018) published a report on the incorporation of nano-graphene into fly ash RAC made entirely of recycled fine aggregates. Graphene nanoparticles were added at 0.05% and 0.1% concentrations, while fly ash was used as a cement replacement at a 30% ratio. Flexural strength increased by 30% and 33% at 28 and 120 days, respectively, at a dosage of 0.05% nano-graphene, which presented the optimal content.
Studies have shown that nanoparticles react with Ca(OH)2 crystals arrayed along with ITZ areas between RCA and hardened mortar paste, producing extra C-S-H gel and filling micropores. As a result, the microstructure becomes more densified and more compacted (Mohamed, Citation2016). Additionally, as compared to free-nano RAC, the nano-sized particles promote the RAC’ bending capacity via increasing the cracks resistance of structures and delaying the initiation of micro-cracks at early ages under high flexural pressures (Zhang et al., Citation2015). However, except for nano-silica, research on the flexural performance of nano-incorporated RAC are very scarce and need further work to determine the optimal ratios of both nanoparticles and RCA corresponding to the highest strength.
Impact resistance
Impact resistance of concrete structure is defined as a structure’s ability to withstand repeated impacts and absorb large energies without causing serious damage, fracture, or immediate failure. The impact load is a well-known dynamic force that acts on the structure during its life. Unlike static load, if the structure, however, not designed to bear dynamic impact load, micro-cracks will quickly be prorogated, causing significant deformations and hence collapse in a short period. In the case of RAC structures, the collapse is more noticeable than it is in conventional concrete, because of the lower density, more porosity, and higher crushing index of RCA compared to NCA (Xiao et al., Citation2015). Researchers, therefore, tried to improve the impact performance of RAC through the use of nanomaterials in its mixtures. Li et al. (Li et al., Citation2016) investigated the impact behaviour of RAC incorporated with 100% of RCA and different types of nanoparticles, namely nano-SiO2 and nano-CaCO3, were added at 1% and 2% of the cement weight, respectively. Split Hopkinson pressure bar (SHPB) was used to determine the impact performance of RAC specimens. Their findings demonstrated a significant increase in dynamic compressive strength, particularly at low impact velocities, with the highest strength observed in the RAC when 1% nano-SiO2 was added. Erdem et al. (Erdem et al., Citation2018), on the other hand, carried out a test to check the effect of adding various dosages nano-SiO2 to RAC mixes on the impact performance. For that purpose, natural aggregates and cement were replaced with 100% RCA and 0.5, 1, 1.5% silica nanoparticles, respectively. Repeated drop weight blows impact (RDWBI) apparatus was employed to determine the impact resistance of NPS-modified RAC. Unlike the results obtained prior (Li et al., Citation2016), integrating 1.5% of nano-SiO2 displayed the highest number of blows, reflecting the best outcome in terms of increase of impact resistance, which increased by 100% and 200%, respectively, at first crack and failure stages. Moreover, the correlation between impact blows and both of compressive strength and flexural strength was established as shown in Figure .
Figure 2. Correlation between strength and impact resistance of RAC (Erdem et al., Citation2018).
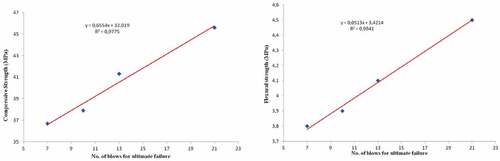
Adding nano-SiO2 to RAC mixes increased impact resistance linearly with compressive and flexural strength, and the greater correlation coefficient revealed a strong relation between impact resistance and strength properties, notably in flexural behaviour.
In conclusion, it has been demonstrated that nanomaterials can significantly improve the impact performance of RAC structures by contributing to the formation of a very dense mix by filling the pores induced by the presence of RCA. However, due to the difficulty of comparing impact results according to the limited number of studies, additional field investigations are required to fully understand the dynamic impact performance of RAC structures strengthened with various types of nanomaterials.
4.4. Bulk density
The bulk density of concrete is regarded as a critical indicator of aggregate gradation and features homogeneity. It expresses the effort required to compact the concrete. Recycled concrete aggregate density is proved to be lower than that of natural aggregate, and the more mortar and contaminants adhered to the recycled coarse aggregate, the lower the density of the RAC mixes. Thus, five experiments have been conducted to increase the bulk density of RAC with the use of nanomaterials. Ismail and Ramli (Ismail & Mahyuddin, Citation2014) demonstrated a remarkable increment in density of RAC due to the addition of silica nanoparticles. 0.75% nano level represented the highest value compared to 0.25, 0.5, and 1%. However, Mukharjee and Barai (Mukharjee & Barai, Citation2015) reported the maximum density of RAC at 3% of nano-silica, increasing up to 9% compared to free-nano RAC and comparable to standard NAC samples.
Singh et al. (Singh et al., Citation2018) showed that immersing RCA into 3% nano-silica slurry resulted in a 29% reduction in volume of voids compared to untreated RAC. Toghroli et al. (Toghroli et al., Citation2020), in different investigation, conducted an extensive study on the nano-clay effect on the RAC bulk density using different RCA replacement ratios (i.e., 10, 25, 50, and 100%), and nanoparticles doses (i.e., 1, 2, and 3%). Their findings showed a significant enhancement in the RAC density, particularly, at high level of clay nanoparticles. Further, increasing the RCA content resulted in more reduction in mixtures density.
As a result, nanoparticles play a significant role in improving the density or recycled aggregates concrete through acting as nanofillers to fill the gaps and lockdown the cracks. However, further studies should be conducted on the effect of other types of nanomaterials.
5. Nanomaterials on durability of RAC
One of the most essential perspectives of RAC is their durability property. It should be emphasized, however, that owing to the variety of RCA in terms of source, replacement ratio, physical characteristics, kind of supplementary additives, and nature of environmental exposure, it is difficult to compare durability findings across prior papers (C. Thomas et al., Citation2013). Generally, a large number of papers have confirmed the poor performance of using RCA in the concrete under harsh environmental conditions (Ma et al., Citation2019). Additionally, the RAC durability have dropped linearly as the RCA replacement ratio increased. These disadvantages could be ascribed mostly to the RAC’s older mortar, which may have contributed to a decline of resistance towards the environmental impacts (Bhasya & Bharatkumar, Citation2018). The presence of attached mortar increases the absorption capacity and permeability of RAC in comparison with conventional concrete blends due to the propagation of visible cracks along old and new ITZ between mortar and aggregates (Bui et al., Citation2018). As a result, researchers have investigated a variety of techniques to improving the durability of RAC by improving the quality and properties of RCA. Despite the abundance of these studies, there are few reviews that focused on how nanomaterials affect the durability properties of RAC. This section critically reviews studies regarding the environmental impacts on the nano-strengthened RAC applications.
5.1. Durability of nano-SiO2 incorporated RAC
Nano-SiO2 is regarded as the most popular type among nanomaterials. Its role in the RAC mixtures is to refine the microstructure by improving the pozzolanic reaction between components and producing extra C-S-H gel (Singh et al., Citation2019). This gel is responsible to improve the durability and extending the life of RAC constructions (Allujami et al., Citation2021). Several studies have explored the effect of silica nanoparticles on the durability aspects of RAC when exposed to environmental impacts. These studies are covered in the following sections.
5.1.1. Permeability and water absorption
One of the major distinctions between RAC and NAC is water absorption. The higher water absorption of RAC is attributed to the existence of attached old mortar around the RCA surfaces (Toghroli et al., Citation2020). A study by Hosseini et al. (Hosseini et al., Citation2011) evaluated its effect on RAC anti-absorption at two distinct nano levels (1.5% and 3%). Their findings showed a reduction in water permeability by improving the microstructure and ITZ of the blends, and that more nano-silica replacement indicated a further enhancement in water absorption and therefore better durability. Meng et al. (R. T. Meng et al., Citation2013) studied the enhancement of water absorption in the RAC by substituting cement with 1% and 2% silica nanoparticles. Despite an acceptable reduction in permeability of about 7% in nano-strengthening RAC samples, two proportions of nano-silica demonstrated the same results. According to Mukharjee and Barai (Mukharjee & Barai, Citation2014c), water absorption increased with an increase in the RCA replacement ratio and decreased with an increase in nano-silica doses. In comparison to traditional RAC with 100% RCA, the addition of 3% NPS reduced water absorption by 34%. Shaikh et al. (F. U. A. Shaikh et al., Citation2015) determined the rate of water absorption with 50 mm thick disk. Natural aggregates were replaced by RCA at 25% and 50%, and nano-silica was included at a dosage of 1% and 2% by weight of cement. It was concluded that the addition of 1% and 2% silica nanoparticles minimized the sorptivity by (12.5%, 35%) and (27%, 32%) in the RAC containing 25% and 50%, respectively. Furthermore, the volume of voids was decreased by 7% and 11% in the samples mixed with 50% of RCA due to the incorporation of 1% and 2% NP, respectively.
On the other hand, Tavakoli et al. (Tavakoli, Citation2014) assessed the influence SiO2 nanoparticles on the rate of water absorption in recycled mortar containing different replacements of waste blocks varying between 25 ~ 100%. Their findings indicated a slight reduction in the water permeability by approximately 8 ~ 10% compared to RAC with zero nanoparticles. That is because silica has created a nucleus to improve the cement hydration products (Mukharjee & Barai, Citation2015).
Shaikh et al. (F. Shaikh et al., Citation2018) also examined the durability performance of nano-silica incorporated RAC, but in their test, water sorptivity was compared adopting two mixing methods of nanoparticles (direct mix and presoaking). Their findings showed that water absorption was reduced by about 53% in samples of 2% NPS slurry, compared to a 41% reduction in the direct mixing method. It was interpreted that presoaking technique caused nanoparticles to disperse more around the surface of RCA, resulting in a 9% decrease in the voids volume when compared to other procedure. Li et al. (Li et al., Citation2017) reached to similar conclusion with the mixes containing 30% RCA and two dosages of silica nanoparticles (i.e., 1% and 2%). Water absorption of nano slurries-modified RAC was found to be 19% lower than that of conventional RAC. Their results agreed with those of Younis and Mustafa (Younis & Mustafa, Citation2018).
Shaban et al. (Shaban et al., Citation2019) also conducted an experimental test to measure the water absorption of RAC due to the modification by nano-silica slurries at ratios of 1%, 2%, and 3% by cement mass. The test was operated for three different presoaking times (i.e., 1, 2 and 4 hours), with another two different presoaking slurries of RCA. These presoaking mediums were fly ash and silica fume, and fly ash and cement at (20%, 40%, and 60%). The results indicated that a concentration of 3% NPS slurries was considered to be the desirable method among the other treatment techniques, and water absorption was decreased by 48% compared to the conventional RAC. Increasing the soaking times up to 4 hour indicated further enhancement when compared to shorter treatment times.
Observations by Nuaklong et al. (Nuaklong et al., Citation2018) suggested an improvement in durability properties when nano-silica was used in RCA geopolymer concrete with calcium-rich fly ash. Nano-silica particles were added at percentage of (1%, 2%, 3%). Compared to the conventional RAC blends, water absorption of NPS-incorporated RAC was decreased by 55%, and the optimal ratio of silica nanoparticles was observed to be 1%. The increase in water absorption of the mixtures with higher proportions of NPS caused by incomplete dispersion of silica in blends, which resulted in a higher volume of voids and thus higher permeability. A similar study was also conducted, but in their report, nano-rice husk ash (NRHA) was mixed into the geopolymer RAC blends in proportions of 1.2%, 2.4%, and 3.6% (Nuaklong et al., Citation2020). The results showed that adding NRHA in the optimal amount of 1.2% reduced water absorption and was more effective in reducing permeability than nano-silica. This behaviour has been attributed to the presence of SiO2-rich materials, resulting in an increase the SiO2/Al2O3 ratio from 4.17 to 4.33.
Sadeghi-Ni et al. (Sadeghi-Nik et al., Citation2019) carried out an experiment to determine the effect of SiO2 nanoparticles on the water absorption of pozzolanic RCA self-compacted concrete at different replacement ratios. Metakaolin and limestone were mixed at a rate of 20% with various RCA replacement contents ranging from 20% to 100%. The combined effect of nanoparticles and metakaolin contributed to a 5% decrease in absorption rather than of limestone incorporation, and the optimal replacement of RCA was 20%. The nanosized silica and the extremely small size of the metakaolin particles aided to reduce the permeable pores by filling and blocking the channels of capillary networks (Chalangaran et al., Citation2020). Agarwal et al. (Agarwal et al., Citation2019) pointed out that higher replacement of nano-silica up to 5% had no significant role in reducing water absorption of RAC.
Tavakoli et al. (Tavakoli et al., Citation2020) investigated a comparative study to determine the rate of water absorption in NPS-incorporated RAC with varying waste materials content, that is, glass sand, ceramic sand, and concrete sand. Nano-SiO2 was added at proportions of 0.5%, 0.7%, 1% and 1.5% as cement weight. The results demonstrated that nano-SiO2 significantly decreased the water absorption of waste concrete mixtures, with the best results obtained when 0.7% NPS was used. In addition, a 40% replacement of glass sand showed the best performance, reducing the absorption by approximately 60% when compared to ceramic and concrete sand, which indicated reductions of 55% and 54%, respectively.
The incorporation of nano-silica can effectively decrease the water absorption of RAC by minimizing the total volume of voids induced by the presence of RCA. However, increasing the NPS concentration may have little effect on permeability owing to the nanoparticles’ tendency to agglomerate, resulting in poor dispersion within the RAC mixes. As a result, in this case, adopting the pre-soaking method has shown a substantial increase in RAC durability when compared to a direct mix of nano-silica, due to uniform dispersion of nanoparticles causing higher packing density of the structure (Mukharjee & Barai, Citation2014a).
5.1.2. Chloride penetration
When it comes to the concrete durability, chloride attack is one of the most dangerous chemical attacks that causes the structure’s durability to deteriorate. When chlorides penetrate the concrete, corrosion of the reinforcement begins by destroying the protective layer around the reinforcement, causing a reduction in concrete durability and complete deterioration of the structure over time (Al-Saleh, Citation2015). However, the performance of chloride permeability in RAC structures is completely different and more susceptible to the risk, owing primarily to the porosity and high permeability factors of RCA compared to natural aggregates (Alabi & Mahachi, Citation2021). In view of this drawback, nano-silica particles have been employed in several studies to enhance the chloride resistance of RAC. This was shown when Zhang et al. (Zhang et al., Citation2016) presented an experimental study to measure the chloride diffusion in RAC blends before and after RCA strengthening with nano-silica slurries. The resistance of chloride diffusion was considerably enhanced by about 15% compared to conventional RAC due to the improvement of the ITZ zones, which contributed to the densification of the microstructure and increased the durability of RAC against chloride attack. Shaikh et al. (F. U. A. Shaikh et al., Citation2015) showed a reduction in chloride diffusion by about 15% and 32% in RAC mixes with 25% RCA replacement ratios by adding silica nanoparticles at 1% and 2%, respectively. Higher RCA replacement rates up to 50% resulted in a reduction of 12% and 28% in blends mixed with 1% and 2% NPS, respectively. Moreover, pre-soaking of RCA in nano slurries produced better results than direct mixing technique, with chloride resistance increasing by 61% and 58% in pre-soaking and direct mixing technique, respectively, when compared to conventional RAC (F. Shaikh et al., Citation2018).
Ying et al. (Ying et al., Citation2017) focused their study on the performance of chloride diffusion in RAC mixed completely with RCA and with different replacement ratios of nano-silica at 1%, 2% and 3%. The resistance to chloride penetration increased by 33% for samples with 2% NPS, while the addition of higher dosages of silica nanoparticles resulted in a slight improvement towards chloride resistance. The correlation between nano-strengthened RAC compressive strength and chloride diffusion was also illustrated. As the compressive strength of RAC mixtures increased, the durability was clearly increased due to the totally enhancement of density and compact microstructure of blends (Zhang et al., Citation2015). Shi et al. (Shi et al., Citation2018) presented a comparative examination to investigate the chloride resistance of RAC by using three enhancement methods (silica fume slurry, fly ash slurry, and nano-silica slurry) with ratios of 0.76%, 0.63%, and 0.13%, respectively. Disks of 50 mm in height and 100 mm in diameter were exposed to rapid chloride migration test for different curing ages of the RAC samples. Their findings indicated a considerable improvement in chloride resistance, with reductions in chloride penetration of approximately 33%, 84%, and 70% for the samples modified with slurries of fly ash, silica fume, and nano-silica, respectively. Increasing the curing times to 56 days showed no remarkable effect on chloride penetration due to the stability of the compressive strength of RAC mixtures. In the study conducted by Nuaklong et al. (Nuaklong et al., Citation2018), RCA geopolymer concrete specimens were immersed in a sodium chloride solution for varying lengths of time (30 to 120 days) to examine the effect of nano-SiO2 admixture on chloride penetration depth. Unlike normal cement composite, when NPS was added, the chloride resistance of geopolymer concrete decreased by 50%, 60%, and 66% for samples mixed with 1%, 2%, and 3%, respectively. Increased exposure time resulted in deeper chloride penetration due to the higher calcium fly content, which caused a decrease in the CaO/SiO2 ratio, resulting in a lower chloride binding capacity. Furthermore, Agarwal et al. (Agarwal et al., Citation2019) evaluated the chloride diffusion of RAC at early curing age using various replacement ratios of RCA, that is, 20%, 40% and 60%, as well as, different replacement content of nano-SiO2, that is, 1%, 3% and 5%. After 7 days, Rapid chloride penetration tests showed that adding NPS at lower ratios minimized the chloride depths by approximately 4%~26%. However, the addition of higher ratios of silica nanoparticles up to 5% showed no enhancement in chloride resistance due to NPS agglomeration caused by improper dispersion of nanoparticles within the RAC blends.
On the other hand, very few studies have discussed the effect of nanoparticles addition on the corrosion behaviour of steel due to chloride penetration in RAC mixtures. Based on that, Zeng et al. (Zeng et al., Citation2019) conducted an experimental test to determine the steel corrosion rate of RAC concrete containing 100% RCA and strengthened with nano-silica slurries. Rapid corrosion test was performed by subjecting the specimens to wetting and drying cycles for up to 22 weeks. During these cycles, RAC were submerged in the 3.5% sodium chloride (NaCl) sol during the wetting period and exposed to air during the drying period. The results showed that nano-silica helped to improve the durability properties by lowering the corrosion rate of the reinforcement by about 27% when compared to conventional RAC without nano modification. The same results were obtained by Alhawat and Ashour (Alhawat & Ashour, Citation2020), but in their approach, RAC were prepared with different replacement percentages of RCA, that is, 25%, 50% and 100%, as well as two different ratios of silica nanoparticles, that is, 1.5% and 3%. Accelerated corrosion test was carried out by immersing reinforced RAC in 3.5% NaCl solution for a period of 2, 5, 10, 15 days. Their findings illustrated an enhancement in corrosion resistance by 31%, 36%, 45% and 48% after 2, 5, 10 and 15 days, respectively. Increasing the RCA content up to 100% resulted in a more significant reduction in corrosion rates by 40%, 42%, 45% and 52%, owing the role of nano-silica as a filler material that filling the pores up to nano size, producing less porous concrete and more resistance to chloride penetration. Doubling the nano content to 3% showed a slight enhancement over 1.5% NPS.
It can be noted that inclusion of nano-silica promotes early cement hydration, resulting in an increase in high-stiffness C-S-H gels. Hence, the RAC becomes strong and dense, and less porous resulting in lower diffusion of chlorides ions. In addition, replacing cement with SCMS composites offers additional protection against chloride attack, due to the dual effect of nano-silica and pozzolanic effect of supplementary materials, producing a very dense structure. However, no remarkable effect on chloride resistance has been noticed when mixing higher content of SiO2 nanoparticles, because of the agglomeration and poor dispersion of NPS.
5.1.3. Fire resistance
The resistance to fire is influenced by the thermal and physical properties of concrete materials such as type of structure, type of aggregates, type of supplementary composites, moisture content, fire rate (Umasabor & Okovido, Citation2018). When concrete is exposed to high temperatures for a long period of time, the strength and stiffness properties are reduced. In addition, the spalling phenomenon may occur, in which materials break off from the concrete surface, the cross-section of elements is reduced and the steel reinforcement degrades (Krzemień & Hager, Citation2015). In the case of sustainable concrete made of RCA, the behaviour of RAC at high temperatures is completely different from that of natural concrete, as extensively reviewed by Cree et al. (Cree et al., Citation2013). Their study presented the unsatisfactory behaviour of RAC at elevated temperatures, and residual compressive strength was discovered to be insignificant compared to normal concrete at the same exposures.
However, studies on the fire resistance performance of nano-incorporated RAC are few, unclear, and need further research. Nuaklong et al. (Nuaklong et al., Citation2020) examined replacing natural aggregates with 100% volume of RCA in high-calcium fly ash geopolymer concrete. Nano-silica was mixed in proportions of 1, 2 and 3% by cement volume. For different exposure times (30, 60 and 90 minutes), the cylindrical specimens were heated to 900°C. Their findings pointed out that, compared with conventional specimen, the addition of silica nanoparticles has no apparent effect on the spalling resistance of RAC because of the decomposition of adhering mortar on the surfacing of the RCA, which led to the sudden collapse of RAC under high fire exposure. In addition, the post-fire residual compressive strength of RAC mixed with nano-SiO2 has decreased by around 10%, 17% and 22%, respectively, at 1%, 2% and 3% addition of NPS. With a similar survey, Nuaklong et al. (Nuaklong et al., Citation2020) incorporated nano-rice husk ash into RAC mixtures at different dosages (1.2%, 2.4%, and 3.6%). Unlike nano-silica, NRHA demonstrated a higher residual compressive strength at elevated temperature, with increases of 12%, 10%, and 6% compared to untreated RAC.
In another research, Yonggui et al. (Yonggui et al., Citation2020) examined the influence of mixing nano-silica on RAC performance after being exposed to different temperatures of 25, 200, 400, and 600 °C. Recycled aggregates and basalt fibers were used at 50% and 100%, and 1 kg/m3, 2 kg/m3 and 3 kg/m3, respectively, as well as nano-silica were included as a cement replacement at 3%, 6% and 8%. At 400°C, the addition of silica nanoparticles up to 3% to samples containing 50% RCA improved the fire resistance by 45% compared to normal RAC. However, as the NPS content increased, the residual compressive strength decreased. Furthermore, at 600°C, all samples demonstrated a decrease in relative compressive strength, with a maximum decrease of about 45% for samples mixed with a higher replacement of nano content (8%) by cement volume. However, at a higher replacement of RCA, the optimum ratio of nano-silica was found to be 6%, with an improvement of about 4% over free nano-RAC.
When RAC is heated to low-medium temperatures, the impact of nano-silica on fire resistance is shown by an increase in residual compressive strength. However, at very high temperatures, nano-silica has indicated no enhanced effect on fire resistance of RAC, especially at higher RCA replacement, owing to the increase in pore content within the mortar matrix and deterioration of ITZ bonding ability (Cree et al., Citation2013), resulting in the structure collapsing suddenly.
5.1.4. Abrasion resistance
Abrasion resistance of concrete is defined as the strength of a concrete surface to withstand the wearing forces caused by rolling wheels, forklift tines, or even falling objects (Ren et al., Citation2017). Pavement’s abrasion resistance is mainly proportional to its compressive strength, W/C value, well-graded aggregates, and type of supplementary cementitious materials (Pyo et al., Citation2018). For a pavement made of RCA materials, the abrasion resistance is reduced because of the inferior and poor quality of the RCA and concrete matrix (Kumar, Citation2017). As a result, studies based on nanotechnology have been conducted to evaluate abrasion resistance of RAC. According to Shaban et al. (Shaban et al., Citation2019), RAC was examined for its abrasion resistance following treatment with nano-silica slurries for different soaking times (1, 2 and 4 h). Pre-soaking of RCA in 3% silica slurry resulted in the highest resistance of around 21% compared to conventional mixtures and higher than samples strengthened with 1% and 2% nano-slurry. Moreover, increasing the soaking time improved abrasion resistance by 36%. Tavakoli et al. (Tavakoli et al., Citation2020) investigated the abrasion resistance of roller-compacted rigid pavement modified with SiO2 nanoparticles and mixed with different types of waste concrete. Their findings revealed that the addition of NPS enhanced the abrasion resistance by 31% when compared to reference RAC. However, the addition of ceramic and glass waste demonstrated a 40% increase in resistance.
As a result, the decreased abrasion resistance of mixes including RCA has been ascribed to the poorer quality of ITZ, the weak connection between the aggregates and mortar pastes, and the lower quality of RCA against abrasion. When nano-silica is introduced to RAC blends, the abrasion impact decreases significantly when compared to untreated RAC. This improvement in abrasion can be attributed to the denser microstructure of the nano-modified RAC and the reduction in pore volume in the hardened cement paste (Adamu, Mohammed, Shafiq, Liew, et al., Citation2018).
5.1.5 Acid resistance and carbonation
Acid attacks are among the most serious threats to the durability of concrete structures. When sulfuric acid attacks concrete, the calcium hydroxide Ca(OH)2 reacts with the acid to form gypsum or ettringite. As a result, the microstructure becomes weak, capillary porosity increases, cement paste is lost, and specimen size decreases, resulting in a reduction in the concrete strength (Makhloufi et al., Citation2014). For RAC, a significant reduction has been observed due to severe acid attacks, resulting in unacceptable performance (Vinay Kumar et al., Citation2018). Hence, the RAC mix composition requires some modifications in terms of nano additives in order to reduce RAC permeability. Rao et al. (Rao et al., Citation2018) assessed the influence of quaternary components on the acid resistance of RAC blends. SCMS like silica fume and fly ash were mixed at 20% and 10% respectively, and nano-silica was added at 2% and 3% of the cement weight. The specimens were placed in 3% and 5% acid chemical solutions for a total of 56 days. The results indicated that compressive strength was reduced by approximately 12% for all specimens due to acid penetration, but the weight loss was more significant for the blends containing 3% silica nanoparticles compared to 2% nano content.
Concrete carbonation is a chemical process by which CO2 penetrates into concrete through pores and reacts with Ca(OH)2 to form calcium carbonates (CaCO3) (Moffatt & Thomas, Citation2017). The carbonation reaction has many impacts on concrete: (1) Carbonation affects strength, porosity, pore distributions and chemical composition of cement materials. (2) Carbonation causes reinforcement corrosion by consuming Ca(OH)2 in a concrete mix, destroying the protective layer around the steel and resulting in deterioration over time. (3) Carbonation promotes the spreads of shrinkage cracks, reducing the durability of concrete structures (W. Liu et al., Citation2016). Therefore, several techniques have been developed to improve the performance of RAC against the carbonated diffusion, but quite a few have focused on the use of nanotechnology. Shi et al. (Shi et al., Citation2018) measured carbonation depths according to Chinese Standard GB/T50082-2009 by placing silica nanoparticle-modified RAC samples in a carbonation room exposed to CO2 levels at 20 ± 3% for 3, 7, 14, and 28 days. When compared to conventional RAC, the findings showed a significant decrease in carbonation depth of about 35%, with an additional improvement achieved by increasing curing ages. Furthermore, the carbonation depth was concluded to have a linear correlation with the compressive strength of RAC, with the carbonation resistance increasing as the compressive strength increased.
Hence, the acid and carbonation resistance of RAC made of waste concrete aggregates has been shown to act lower compared to normal aggregates concrete due to the large volume voids available in RCA, permitting chemicals to penetrate easily into the concrete blends, resulting in a sharp decline in mechanical performance of RAC. However, the pozzolanic nature and the nano-sized particle of nano-silica when incorporated to RAC have shown an enhanced performance due to the filling effect of nanoparticles, resulting in a highly dense structure and decreased of acids and CO2 diffusions compared to untreated RAC.
5.2. Durability of Common Nanomaterials Incorporated RAC
Nano-CaCO3 is another nanomaterial whose impact on the RAC lifespan has been investigated before. Meng et al. (R. T. Meng et al., Citation2013) noticed that incorporating CaCO3 nanoparticles at 15% had no effect on RAC water absorption compared to conventional samples and was less effective than the nano-silica modification. A similar procedure was conducted by Zhang et al. (Zhang et al., Citation2016), but in their study, RCA was immersed in a slurry of both nano-lime and nano-silica. Water absorption was reduced by 44% compared to RCA without treatment and better than RCA treated with nano-silica solution only. Li et al. (Li et al., Citation2017) published an experiment to determine the rate of water absorption in RAC modified with nano-limestone powder at dosages of 1% and 2% of cement weight. The water absorption of nano-strengthened RAC was reduced by about 11% compared to the reference. It was also found that the chloride diffusion coefficient was decreased by 10% as a result of surface treatment with silica and calcium nanoparticles, which were closely related to the enhancement of ITZ areas between cement and aggregates (Zhang et al., Citation2016).
Titanium nanoparticles, on the other hand, have been suggested as a way to improve the durability of RAC. Ying et al. (Ying et al., Citation2017) observed that adding nano-TiO2 significantly reduced the chloride diffusivity by approximately 56%, 77%, and 11% for RAC mixed with 1%, 2% and 3% NPS, respectively. In addition, Chen et al.
(Chen & Kou, Citation2019) investigated the sulfur degradation of composite photocatalysts RAC prepared with recycled fine aggregates (RFA) soaked in 1-g NPS per 100 mL deionized water. Compared to control mixtures, the absorption and sulfur degradation of nano-modified RAC were 46% and 24% higher, respectively. In terms of fire resistance, Moro et al. (Moro et al., Citation2020) examined the relationship between nano-TiO2 and RAC’s ability to withstand fires at 100% RFA. Compression and flexural strength increased with the inclusion of NPS under normal temperature conditions; however, this enhancement produced a dramatic drop in strength when exposed to elevated temperatures.
Nano-clay and nano-aluminum were also employed to improve the durability of RAC. Abdul-Hamead et al. (Abdul-Hamead et al., Citation2018) found that the optimal dosage of aluminum nanoparticles was 1.5%, and water absorption of RAC was decreased by 10%. On the other hand, it was found that adding clay nanoparticles in the amount of 2.5% of cement weight reduced water absorption rate to its maximum. In addition, increasing RCA replacement ratio resulted in a higher rate of permeability (Toghroli et al., Citation2020).
Graphene, a modern emerging nanomaterial, is now thought to be a superior additive, significantly increasing concrete strength and water resistance (Akarsh et al., Citation2021). However, its utility in RAC applications is still limited, necessitating additional testing. Sharma and Arora (Sharma & Arora, Citation2018) studied the performance of nano-graphene enhanced fly ash RAC in proportions of 0.05% and 0.1% exposed to sulphate attack for up to 125 days. The results showed that higher compressive and flexural strength was achieved for specimens with 0.05% graphene. This behaviour is attributed to the role of nano-graphene to adsorb the attacking ions presented in the pore solution and rendering them ineffective for hydrate quality.
Studies have shown that nano-CaCO3, nano-TiO2, nano-clay, and nano-carbon have positively affected the durability of RAC, in terms of water absorption, chemicals diffusions, and fire resistance. This is due to the nano-filler effect, providing a densifying microstructure of RAC and homogenous blends to avoid the harmful substances penetrating the structures. However, with higher RCA substitution, nanoparticles have been concluded to have less role in the resistance to environmental variables than in lower substitution rates because of the large volume voids in which nanoparticles cannot completely fill them, resulting in easy penetrating of external chemicals into the cement paste. Additionally, with relatively few durability tests for nano-incorporated RAC made of waste concrete aggregates, more research should be carried out to reach the optimal results and to comprehensively understanding the actual behaviour towards external environments.
6. Nanomaterials on microstructure of RAC
Understanding the microstructure of natural concrete has become an urgent need to analyze the internal morphology of pore structure, cementitious paste, and ITZ zone between aggregates and cement paste. RAC, regardless of its classification, can share common microstructural properties, with considerably more complex structural system than normal concrete, depending on morphological, physical and chemical properties, as well as secondary ITZ zones within RCA (Evangelista & Guedes, Citation2018). These are keys that influence the microstructure development and, thus, the macroscopic hardened properties of RAC. Despite numerous investigations, understanding the microstructure of nano-modified RAC remains a significant challenge and needs more analysis. The most common methods for analyzing microstructure are scanning electron microscopy (SEM), X-ray diffraction, and microhardness which are covered in the following sections.
6.1. Scanning Electron Microscope
A scanning electron microscope (SEM) is one of the famous techniques to analyze the microstructure of nano-incorporated RAC. With this technique, high magnification and spatial resolution were obtained to determine the details of individual pieces of the structure (Evangelista & Guedes, Citation2018). By incorporating nanomaterials, the morphological distribution of RAC can be developed via the nano-size effect, interface improving effect, filler effect, pozzolanic effect, and high surface area effect of nanoparticles (Yonggui et al., Citation2020). In addition, nanograins can act as activators, promoting the hydration process inside RAC blends (Hosseini et al., Citation2009). Through the microstructural analysis, Wang et al. (Y. Wang et al., Citation2019) discovered that the presence of nano-SiO2 significantly improved the pore structure of RAC, with pores becoming smaller and more compacted. SEM revealed that nanoparticles could react with Ca(OH)2 to produce additional C-S-H gel, filling the pores and increasing the density of RAC blends. As well, Hosseini et al. (Hosseini et al., Citation2011) stated that the utilize of SiO2 nanoparticles improved the ITZ zones between RCA and mortar matrices, resulting in a reduction in porosity and an enhancement in ITZ strength. Using SEM images, Shaikh et al. (F. Shaikh et al., Citation2018) showed that the morphology of the microstructures of the matrix were compacted more due to the inclusion of nano-silica, and that presoaking RCA in the nano solution indicated more improvement compared to direct mix approach. Additionally, SEM identified considerably fewer microcracks in the ITZ after incorporation of nanoparticles (Li et al., Citation2017). A similar approach was also conducted by Younis and Mustafa (Younis & Mustafa, Citation2018), that microscopically studied three different ITZ zones (ITZ1 between NCA and old mortar, ITZ2 between new mortar and NCA, and ITZ3 between new and old mortar). Unlike conventional RAC, the ITZ3 in the nano-strengthened RAC could not be identified due to its high densification, and the zone between old and new cement paste was seen to be connected very well. Additionally, the high concentrations of Ca(OH)2 causing high porosity in RAC were overcome by nanoparticles that acted together, resulting in an extra C-S-H gel and thus filling the micropores and improving the strength and durability of the mixtures. Hosseini et al. (Hosseini et al., Citation2011) noticed that increasing the content of nano-silica resulted in an unstable microstructure due to depression issues and agglomeration phenomena with a higher dosage of nanoparticles, leading to a loss of workability and flowability of fresh RAC mixtures. Ismail and Ramli (Ismail & Mahyuddin, Citation2014) examined the microscopic structure of RAC containing 100% recycled coarse aggregates impregnated with nano-SiO2 solutions of varying concentrations. SEM tests illustrated an enhancement in the microstructure of treated RAC, accelerating the hydration reactions, enhancing the development of large quantities of hydrants components, and hence improving the ITZ and increasing the strength of blends. Yonggui et al. (Yonggui et al., Citation2020), in a different investigation, studied the microstructure of nano-modified RAC before and after subjected to elevated temperatures. Images of SEM plotted a deterioration of the ITZ bonding performance with the increase of temperatures, and the gaps between cement mixture compounds were gradually increased. Despite the ability of silica nanoparticles to improve the compactness of the RAC matrix at ambient temperatures, their effect at elevated temperatures led to an increase in pore content inside the mortar matrix, and the structure gradually became looser.
From another perspective, the surface morphology analysis revealed that nano-metakaolin significantly densified the microstructure of RAC due to very fine particles of metakaolin and their rough surfaces (Muduli & Mukharjee, Citation2019). Furthermore, Xie et al. (Xie et al., Citation2020) performed a comparative research to analyze the internal microstructure of nano-incorporated RAC by using two RAC specimens representing the highest and lowest strength with 5% metakaolin nanoparticles addition. For the higher strength sample, SEM indicated that metakaolin nanoparticles could fill a portion of mesopores and macropores, reducing the total pore volume of the RAC, and act as a strong volcanic ash, generating a large amount of C-S-H gel. For the lower strength sample, the presence of NPS could not overcome the large voids caused by the large replacement of RCA, and cracks or interconnected pores were still observed.
According to Li et al. (Li et al., Citation2017), nano-Al2O3 enhanced the microstructure of RAC. However, more microcracks were observed in the ITZ compared to RAC modified with nano-SiO2. This was because of the poor dispersion of alumina nanoparticles, which made it difficult to enter into ITZ zones to speed up the chemical reactions.
Moro et al. (Moro et al., Citation2020) examined the morphological features to investigate the nano-TiO2 effect on SEM contours of RAC blends exposed to elevated temperatures. SEM showed a significant densification in ITZ zones as a result of the adding 1% titanium nanoparticles, producing mixtures with lower porosity. This morphology was seen in the mixes at ambient temperatures. However, at elevated temperatures, the microstructure of nano-modified RAC changed due to the variations in the coefficient of thermal expansion between ITZ zones caused by TiO2, resulting in generation of internal stresses and a reduction of RAC strength. Xu et al. (Xu et al., Citation2020) revealed that immersing RCA in low TiO2 concentration solutions enhanced the formation of cement hydration products, resulting in a better RAC microstructure. However, increasing the concentration of TiO2 solutions produced an adverse effect of generating more calcium meteorites and thus reducing the RAC performance.
Song et al. (Bin Song et al., Citation2021) observed that incorporating MWCNTS into RAC mixtures significantly improved the compactness of microstructure in ITZ zones, by acting as bridging across the microcracks as shown in Figure , providing an effective load transfer, which eventually improved the performance of the RAC mixtures.
Figure 3. SEM images of MWCNTS-modified RAC (Bin Song et al., Citation2021).
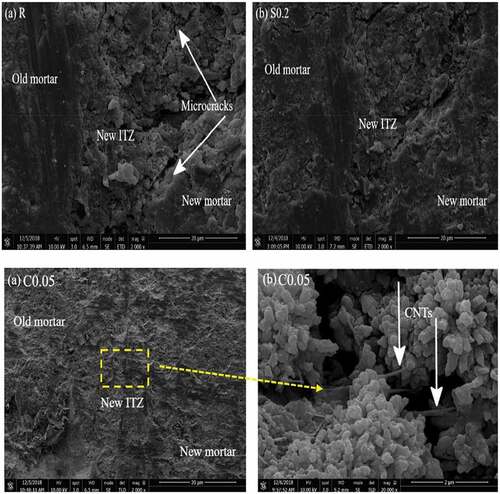
Sharma and Arora (Sharma & Arora, Citation2018), on the other hand, plotted SEM images of RAC mixes comprising 100% of recycled fine aggregates (RFA) and incorporated with 0.05% and 0.1% of nano-graphene. The results indicated a very dense microstructure due to the graphene’s nanofiller effect, especially at a low percentage of nanoparticles, indicating representing the higher mechanical strength of RAC. However, at 0.1% of graphene nanoparticles, SEM analysis showed agglomeration of nanoparticles on the surface of C-S-H products, resulting in a decrease in the strength of RAC compared to 0.05% content.
Analyzing the microstructure of nano-modified RAC via SEM technique has revealed an improved mixture due to the presence of nanoparticles, which acting as nanofillers, filling the micropores caused by RCA, react with Ca (OH)2 and develop additional C-S-H in the blends. As a result, the mechanical strengths and durability of RAC can be greatly enhanced. In addition, after being modified with nanoparticles, the weak ITZ zones, as shown in SEM images, have been improved in which nanoparticles densify the areas between mortar and aggregates, creating a homogeneous structure and lower porosity across ITZ zones. However, in conjunction with mechanical properties, morphological features have demonstrated that modifying RAC with higher proportions of nanoparticles could not well improve the microstructure of RAC due to the agglomeration phenomenon, resulting in irregular dispersion of nanoparticles inside the mixtures and producing unstable structure towards external loads and chemical attacks.
6.2. X-Ray Diffraction and Energy-dispersive X-Ray Spectroscopy
The X-ray diffraction (XRD) technique has been widely used to analyze the crystallographic structure of concrete as well as to define the intensity and quantification of cementitious components (Bahoria et al., Citation2018). XRD analysis was estimated in a few papers to study the nano effect on the crystalline state of RAC. Wang et al. (Y. Wang et al., Citation2019) used XRD to investigate the influence of nano-SiO2 on cement hydration after 28 days. XRD patterns plotted a decrease in intensity related to the Ca(OH)2 crystal after the addition of nanoparticles, which inhibited the formation of Ca(OH)2 crystal and aided to create a wide range of C-S-H. Furthermore, the incorporation of micro-CaCO3 to the nano-modified RAC resulted in additional decrease in Ca(OH)2 intensity by 46% when compared to normal RAC (Yue et al., Citation2020). By XRD patterns, Moro et al. (Moro et al., Citation2020) pointed out that adding nano-TiO2 to the RAC reduced Ca(OH)2 consumption despite a loss in compressive strength at elevated temperatures.
For further research, XRD analysis has been transformed into the so-called energy-dispersive spectroscopy (EDS) to analyze the chemical properties of structure and the calcium-silicon (Ca/Si) ratio to establish the presence of hydration product as a C-S-H gel or a Ca(OH)2 crystal (Rossen & Scrivener, Citation2017). EDS test carried out by Wang et al. (Y. Wang et al., Citation2019) found that the Ca/Si ratio in a new cement paste decreased by 83% and 86% due to the incorporation of 3% and 6%, respectively, compared to conventional RAC. However, increasing the silica nano content up to 8%, the Ca/Si ratio increased once more due to the agglomeration phenomenon of excessive NPS, resulting in a reduction in compressive strength and confirmed by the SEM results. Mukharjee and Barai (Mukharjee & Barai, Citation2017) conducted EDS analysis to determine the mineralogy of RAC mixes containing 3% nano-SiO2. The patterns of EDS depicted that Si and OH were the most abundant elements presented over the aggregate surface (spectrum 1 and spectrum 3), while Ca, Si, OH, and Fe were more noticeable in that part of cement mortar (spectrum 2 and spectrum 4) as illustrated in Figure .
Figure 4. EDS analysis of nano-SiO2 treated RAC (Mukharjee & Barai, Citation2017).
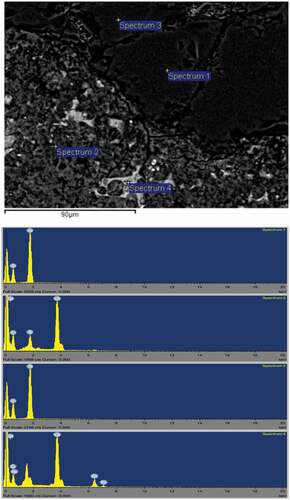
Shaban et al. (Shaban et al., Citation2019) carried out a comparative study to examine the EDS of RCA modified by different slurries. According to EDS analysis, RCA treated with (fly ash & silica fume) slurry had the lowest Ca/Si ratio when compared to nano-silica strengthened RCA sample. Moreover, a linear correlation between atomic Ca/Si ratio and impact value of nano-treated RCA was discovered.
Due to the higher porosity of RFA compared to NA, EDS observation showed that TiO2 nanoparticles, with higher concentration of titania (Ti), were more effective in RAC than TiO2-treated NAC. 0.0023 and 0.0015 g of nano-TiO2 were observed to be loaded in RFA and NA, respectively (Chen & Kou, Citation2019). Sharma and Arora (Sharma & Arora, Citation2018) conducted EDS mineralogy to confirm the presence of nano-graphene in RAC. The EDS pattern indicated that graphene was found to exist as agglomerated particles across RAC microstructure.
The XRD technique used to determine the crystallization feature in nano-modified RAC has revealed very lowed intensities of Ca(OH)2 due to the presence of nanoparticles, confirming the formation of wide ranges of C-S-H products along with ITZ zones and mortar matrices, and compatible with the SEM images. In addition, EDS analysis has emphasized the presence of hydration products, indicating lower values of Ca/Si due to the incorporation of nanoparticles, and higher values of Ca/Si at higher nano content, representing the poor mechanical performance and less durability of RAC. However, more microstructure analysis using XRD and EDS is required to provide a comprehensive examination of nano-modified RAC mixtures for being important to reflect, lately, the actual behaviour of the structure and to determine the optimized contents for both nanomaterials and RCA within the blends.
Micro-hardness
Micro-hardness testing is a technique for evaluating the hardness or resistance to a material’s deformation. This approach is commonly used in RAC elements to analyze the growth shape of mortar on the aggregate surface as well as the strength variations in the ITZ zones of aggregate, old mortar, and new mortar (Lee & Choi, Citation2013). In recent years, the micro-hardness test has included nano-RAC. Li et al. (Li, Xuan, Chu, et al., Citation2021) assessed the micro-hardness of RAC strengthened with nano-SiO2 at four levels (i.e., 2%, 4%, 6%, and 8%) using two different treatments.
Figure showed large variations in micro-hardness values when utilizing pre-spraying method, but consistent values when pre-mixing. Furthermore, adding nano-SiO2 improved microhardness at new mortar, old mortar, and new ITZ with the perfect mix when incorporating 4% nanoparticles, and matched the highest compressive strength of 43 MPa compared to 37 MPa in the control mix. When air-drying technique was added to nano-modification of RCA, the micro-hardness of RAC improved even more (Li, Xuan, Sojobi, et al., Citation2021). According to Zeng et al. (Zeng et al., Citation2019), nano-SiO2 suspension at 12% improved the micro-hardness of RAC relative to unmodified RAC. It demonstrated the highest in the ITZ between natural aggregates and old mortar, with a 26.78 HV compared to a 26.56 HV prior to nano strengthening. However, a high variation in the micro-hardness was observed at ITZ between old and new mortar indicating a 13% improvement compared to control. Mukharjee and Barai found (Mukharjee & Barai, Citation2014a) found that Vickers micro-hardness of ITZ in RAC was lower than in NAC, indicating more pores and cracks within the mix. However, the incorporation of 3% nano-SiO2 into concrete resulted in an increase in the micro-hardness of ITZ of RAC and this improvement was brought by a reduction in porosity across ITZ. Shi et al. (Shi et al., Citation2018) found that enhancing RAC by SiO2 nanoparticles slurry increased the micro-hardness of old ITZ by 55.6% compared to untreated RAC and higher than other treatment like CO2 and fly ash slurry. Moreover, a good linear correlation between micro-hardness and compressive strength was observed.
Figure 5. Micro-hardness of nano-SiO2 strengthened RAC (Li, Xuan, Chu, et al., Citation2021). (a) Pre-spraying. (b) Pre-mixing.
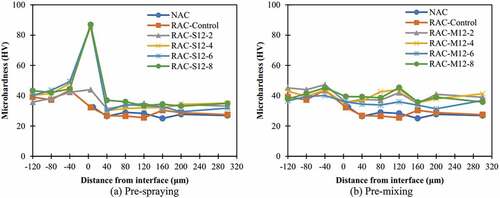
Micro-hardness testing is critical, but additional research is needed into other nanomaterials to better understand the hardness properties of RAC parts.
7. Dispersion
Reactivity, destiny, and transit are all influenced by NPS’ dispersion state (Sanchez et al., Citation2018). As NPS agglomerate, a portion of their surface area is covered, making it unavailable for response. This is particularly important. Accordingly, it has been proposed that NPS should be adequately dispersed prior to mixing with cement, aggregates, admixtures, and water. NPS have been used as nano powders in cement products in the general. In most cases, the nano powders were merely added to the final products as a filler. Ultrasonication was used with or without a chemical dispersing agent to pre-disperse the nano-powders in water (Li et al., Citation2017; Meng et al., Citation2020; Reales et al., Citation2021). Some investigations used NPS that were pre-dispersed in an aqueous solution before being used (Agarwal et al., Citation2019; Amin et al., Citation2015; Younis & Mustafa, Citation2018). It’s been assumed that NPs are uniformly distributed in the cement matrix in some conditions. However, on practical, in most scenarios, nanoparticle could agglomerate and form weak zones in the concrete matrix (Agarwal et al., Citation2019; Li et al., Citation2016), and the compressive strength and other mechanical properties were shown to be improved by sonicating powder-form NPs prior to mixing as previously mentioned.
Using organic compounds as dispersion agents, such as polycarboxylate-based water reducers and probe sonication (Sharma & Arora, Citation2018; Xu et al., Citation2020), has been particularly successful. Dispersion was facilitated in deionized water and in weak ionic solutions by the application of these agents, which led to the creation of a bubble enclosing agglomerates, which would be a process leading to the electrostatic barrier between NPS (Sharma & Arora, Citation2018). However, in strong ionic solutions, the circle is destroyed, and NPS agglomeration proceeds similarly to that with NPS without surfactant when they are mixed with cement and water (Yue et al., Citation2020).
8. Challenges and recommendations
Findings have shown that a number of interesting outcomes can improve the mechanical and durability aspects of the RAC. However, the lack of guidelines and regulations to employ nanomaterials in the RAC mixtures is governed by familiar criteria to achieve purposes. The discrepancies between the studies could be ascribed to the different types of RCA, type of nanomaterials and their proportions, type of additives and reinforcement. Therefore, carrying out more field test can play a significant role in formulating and developing standards and codes that can be depended on later in the future to perfectly design nano-incorporated RAC structures.
In addition, the design of RAC under dynamic forces is similar to that of natural concrete and has been widely discussed in previous times, however, the addition of nanomaterials can totally change the dynamic performance and practical studies should be more estimated. The utilization of nano-modified RAC in harsh environmental conditions is not well understood, making it difficult to determine the optimal behaviour and optimal nano type, particularly when RAC structures come into contact with water medium, where the combined effect of nanomaterials and external chemicals remains mysterious. Furthermore, calculating the overall cost of nano-enhanced RAC is a major issue that should not be overlooked owing to the massive amount of nanoparticles needed to construct the structure, particularly in large private facilities. This paper can serve as a foundation for filling gaps that have not been addressed previously, and future work can be expanded to include:
In-situ evaluation of the practical performance of current RCA structures is essential for determining the actual effect of nanomaterials on RAC applications. Detailed reports should be provided on the origins and physical nature of RCA.
A novel nanomaterial-modified binder with superior behaviour is needed to make RAC mixes with different nanomaterials perform similarly at the same optimal binder concentration.
Additional research is also required on the application of nanomaterials RAC structures exposed to high dynamic stresses.
It is essential that future studies should provide extensive experiments on the use of nanomaterials in reinforced RAC blends. Different types of reinforcement should be included.
More field investigations are required to ascertain the performance of supplementary cementing materials when incorporated with nano-modified RAC. The best exercise to tackle this is to compare the performance of RAC mixes at the optimum content of nanomaterials to their performance at optimum content of cementing materials at different RCA replacement levels.
More studies are necessary to determine the external environmental impacts on the nano-modified RAC structures. Wear resistance in rigid pavement, fire resistance at high elevated temperatures, carbonation diffusion need more field testing.
The relationship between internal microstructure and RAC behavior under external pressures and environmental effects owing to nano addition is yet unknown, and more research is needed to address this critical area.
Cost analysis of nano-modified sustainable buildings needs a lot of efforts to choose the optimized solution.
9. Conclusions
The effect of nanomaterials on the fresh, hardened and durability properties of RAC was reviewed. Workability; compressive, tensile, flexural, impact strength, and bulk density; water absorption, chloride permeability, fire resistance, abrasion resistance, acid and carbonation resistance were discussed. The efficiency and optimal behaviour of nano-modified RAC were also presented. On the basis of this paper, the main conclusions can be drawn as follows:
In most cases, nanomaterials reduce the workability of fresh RAC mixes, with the reduction increasing as RCA and nanoparticles levels rise. The introduction of nanoparticles reduces slump and flowability of RAC mixtures by a maximum of 59% and 16%, respectively. However, a proper treatment of RCA with nanoparticles, like pre-soaking technique, increases the workability of fresh mixes.
Despite their inferior efficiency in fresh mixes, the inclusion of nano-based materials improves the compressive, tensile, and flexural strength of RAC significantly. Mixing a low dosage of nano-clay or nano-CaCO3 up to 1% indicates the maximum strength as compared to higher nanodosages. The optimal percentage of nano-TiO2 is 2%, while the maximum MWCNTS content corresponding to the highest strength is estimated to be 0.1 or 0.2%.
Pre-treatment of RCA presents better mechanical performance of RAC. Pre-soaking of RCA in the nano-slurries displays higher hardened strength compared to other mixing techniques.
Despite the conflicting results, the addition of nano-SiO2 improves the overall durability of RAC. Even at low concentrations, SiO2 nanoparticles improve permeability and water absorption compared to higher nanoparticles levels. Similarly, a significant enhancement of 15%~66% in chloride resistance is presented at a low content of nano-SiO2, with no noticeable findings at higher nano proportions. Improvement in acid and carbonation resistance of SiO2-incoprorated RAC is demonstrated.
The fire resistance of SiO2-strengthened RAC is increased by 10%~45% when the inclusion 1%~3% of NPS. higher proportions of SiO2 nanoparticles cause negative fire resistance of RAC. A great improvement in abrasion resistance of up to 31% is observed compared to the control RAC.
The use of nano-clay, nano-TiO2, nano-CaCO3, and nano-carbon revealed a lack of understanding of RAC performance under harsh environmental conditions; further research is needed.
Pre-soaking RCA in nanosized solutions shows better durability than direct mixing method due to the proper dispersion of nanoparticles, resulting in more homogeneous and denser mixtures that are more resistant to the aggressive environmental impacts.
A further improvement in the durability of nano-modified RAC is shown when supplementary cementitious materials are used.
Nano addition causes complex microstructure and mineralogy changes in RAC mixtures system. SEM images demonstrate a change in porosity and pore size distributions, resulting in improving the ITZ zones and reducing the microcracks propagations. XRD and EDS analysis are found to be compatible with SEM results, which demonstrate a wide range of C-S-H gel distributed over cement mortar and ITZ zones, with a significant decrease in Ca/Si ratio due to the presence of nanoparticles. Furthermore, the incorporation of nanomaterials significantly increases the microhardness values of RAC, which has a high linear correlation with the strength values of treated mixes.
Dispersion of nanomaterials in RAC mixes is a critical issue, and novel techniques are needed to ensure uniform dispersion and prevent agglomeration of nanoparticles, especially at higher nano levels, or unsatisfactory results and unexpected behaviour can be produced.
Acknowledgements
The authors would like to thank the UCSI University for their financial support through the Research Excellence and Innovation Grant (REIG-FETBE-2021/002).
Disclosure statement
No potential conflict of interest was reported by the author(s).
Additional information
Funding
References
- Abdul-Hamead, A. A., Mohammed, R. K., & Othman, F. M. (2018). Investigation the effect of nano–particles and recycling mortar additives on physical and mechanical properties of concrete. Engineering and Technology Journal, 36(3), 295–38. https://doi.org/10.30684/etj.36.3a.8
- Abu Al-Rub, R. K., Ashour, A. I., & Tyson, B. M. (2012, Oct). On the aspect ratio effect of multi-walled carbon nanotube reinforcements on the mechanical properties of cementitious nanocomposites. construction and Building Materials, 35, 647–655. https://doi.org/10.1016/j.conbuildmat.2012.04.086
- Adamu, M., Mohammed, B. S., Shafiq, N., & Liew, M. S. (2018). Durability performance of high volume fly ash roller compacted concrete pavement containing crumb rubber and nano silica. Int. J. Pavement Eng, 5(10), 53–61. https://doi.org/10.1080/10298436.2018.1547825
- Adamu, M., Mohammed, B. S., Shafiq, N., Shahir Liew, M., & Zampieri, P. (2018). Effect of crumb rubber and nano silica on the fatigue performance of roller compacted concrete pavement. Cogent engineering, 5(1), 1–10. https://doi.org/10.1080/23311916.2018.1436027
- Agarwal, A., Bhusnur, S., & Shanmuga Priya, T. (2019). Experimental investigation on recycled aggregate with laboratory concrete waste and nano-silica. Materials Today: Proceedings, 22, 1433–1442. https://doi.org/10.1016/j.matpr.2020.01.487
- Akarsh, P. K., Marathe, S., & Bhat, A. K. (2021, Jan). Influence of graphene oxide on properties of concrete in the presence of silica fumes and M-sand. construction and Building Materials, 268, 121093. https://doi.org/10.1016/j.conbuildmat.2020.121093
- Alabi, S. A., & Mahachi, J. (2021). Chloride ion penetration performance of recycled concrete with different geopolymers. Materials Today: Proceedings, 38, 762–766. https://doi.org/10.1016/j.matpr.2020.04.199
- Alhans, R., Singh, A., Singhal, C., Narang, J., Wadhwa, S., & Mathur, A. (2018). Comparative analysis of single-walled and multi-walled carbon nanotubes for electrochemical sensing of glucose on gold printed circuit boards. Materials Science and Engineering: C, 90(April), 273–279. https://doi.org/10.1016/j.msec.2018.04.072
- Alhawat, M., & Ashour, A. (2020). Bond strength between corroded steel and recycled aggregate concrete incorporating nano silica. construction and Building Materials, 237, 117441. https://doi.org/10.1016/j.conbuildmat.2019.117441
- Ali, B., & Qureshi, L. A. (2019). Influence of glass fibers on mechanical and durability performance of concrete with recycled aggregates. construction and Building Materials, 228, 116783. https://doi.org/10.1016/j.conbuildmat.2019.116783
- Ali Shafabakhsh, G., Janaki, A. M., & Ani, O. J. (2020). Laboratory investigation on durability of nano clay modified concrete pavement. Engineering Journal, 24(3), 35–44. https://doi.org/10.4186/ej.2020.24.3.35
- Allujami, H. M., Jassam, T. M., & Al-Mansob, R. A. (2021). Nanomaterials characteristics and current utilization status in rigid pavements: Mechanical features and sustainability. A review. Materials Today: Proceedings. https://doi.org/10.1016/j.matpr.2021.04.077
- Al-Saleh, S. A. (2015). Analysis of total chloride content in concrete. Case Stud. Constr. Mater, 3, 78–82. https://doi.org/10.1016/j.cscm.2015.06.001
- Amin, M. S., El-Gamal, S. M. A., & Hashem, F. S. (2015). Fire resistance and mechanical properties of carbon nanotubes - Clay bricks wastes (Homra) composites cement. construction and Building Materials, 98, 237–249. https://doi.org/10.1016/j.conbuildmat.2015.08.074
- Arora, S., Singh, B., & Bhardwaj, B. (2019). Strength performance of recycled aggregate concretes containing mineral admixtures and their performance prediction through various modeling techniques. journal of Building Engineering, 24(November 2018), 100741. https://doi.org/10.1016/j.jobe.2019.100741
- Aslani, F., Ma, G., Yim Wan, D. L., & Muselin, G. (2018). Development of high-performance self-compacting concrete using waste recycled concrete aggregates and rubber granules. Journal of Cleaner Production, 182, 553–566. https://doi.org/10.1016/J.JCLEPRO.2018.02.074
- Bahoria, B. V., Parbat, D. K., & Nagarnaik, P. B. (2018, Jan). XRD analysis of natural sand, quarry dust, waste plastic (ldpe) to be used as a fine aggregate in concrete. Materials Today: Proceedings, 5(1), 1432–1438. https://doi.org/10.1016/j.matpr.2017.11.230
- Bhasya, V., & Bharatkumar, B. H. (2018, Mar). Mechanical and durability properties of concrete produced with treated recycled concrete aggregate. ACI Materials Journal, 115(2), 209–217. https://doi.org/10.14359/51701239
- Bin Song, X., Zhi Li, C., Dan Chen, D., & Lin Gu, X. (2021). Interfacial mechanical properties of recycled aggregate concrete reinforced by nano-materials. Construction and Building Materials, 270, 121446. https://doi.org/10.1016/j.conbuildmat.2020.121446
- Bui, N. K., Satomi, T., & Takahashi, H. (2018). Mechanical properties of concrete containing 100% treated coarse recycled concrete aggregate. construction and Building Materials, 163, 496–507. https://doi.org/10.1016/j.conbuildmat.2017.12.131
- Camiletti, J., Soliman, A. M., & Nehdi, M. L. (2013, Mar). Effect of nano-calcium carbonate on early-age properties of ultra-high-performance concrete. magazine of Concrete Research, 65(5), 297–307. https://doi.org/10.1680/macr.12.00015
- Carriço, A., Bogas, J. A., Hawreen, A., & Guedes, M. (2018). Durability of multi-walled carbon nanotube reinforced concrete. Construction and Building Materials, 164, 121–133. https://doi.org/10.1016/j.conbuildmat.2017.12.221
- Chalangaran, N., Farzampour, A., & Paslar, N. (2020). Nano silica and metakaolin effects on the behavior of concrete containing rubber crumbs. CivilEng, 1(3), 264–274. https://doi.org/10.3390/civileng1030017
- Chenari, H. M., Seibel, C., Hauschild, D., Reinert, F., & Abdollahian, H. (2016). Titanium dioxide nanoparticles: Synthesis, X-ray line analysis and chemical composition study. materials Research, 19(6), 1319–1323. https://doi.org/10.1590/1980-5373-MR-2016-0288
- Chen, X. F., & Kou, S. C. (2019). Sulfur dioxide degradation by composite photocatalysts prepared by recycled fine aggregates and nanoscale titanium dioxide. Nanomaterials, 9(11), 1533. https://doi.org/10.3390/nano9111533
- Cree, D., Green, M., & Noumowé, A. (2013). Residual strength of concrete containing recycled materials after exposure to fire: A review. construction and Building Materials, 45, 208–223. https://doi.org/10.1016/j.conbuildmat.2013.04.005
- Dawood, E. T., & Mahmood, M. S. (2021, Jun). Production of sustainable concrete brick units using nano-silica. Case Stud. Constr. Mater, 14, e00498. https://doi.org/10.1016/J.CSCM.2021.E00498
- De Brito, J., Agrela, F., & Silva, R. V. (2019, Jan). Legal regulations of recycled aggregate concrete in buildings and roads. New Trends Eco-efficient Recycl. Concr, 509–526. https://doi.org/10.1016/B978-0-08-102480-5.00018-X
- De Brito, J., Ferreira, J., Pacheco, J., Soares, D., & Guerreiro, M. (2016). Structural, material, mechanical and durability properties and behaviour of recycled aggregates concrete. journal of Building Engineering, 6, 1–16. https://doi.org/10.1016/j.jobe.2016.02.003
- Erdem, S., Hanbay, S., & Güler, Z. (2018). Micromechanical damage analysis and engineering performance of concrete with colloidal nano-silica and demolished concrete aggregates. construction and Building Materials, 171, 634–642. https://doi.org/10.1016/j.conbuildmat.2018.03.197
- Evangelista, L., & Guedes, M. (2018). Microstructural studies on recycled aggregate concrete. New trends in eco-efficient and recycled concrete, 24(11), 425–451. https://doi.org/10.1016/B978-0-08-102480-5.00014-2
- Feng, W., Tang, Y., Zhang, Y., Qi, C., Ma, L., & Li, L. (2022, Mar). Partially fly ash and nano-silica incorporated recycled coarse aggregate based concrete: Constitutive model and enhancement mechanism. Journal of Materials Research and Technology, 17, 192–210. https://doi.org/10.1016/J.JMRT.2021.12.135
- Feng, W., Wang, Y., Sun, J., Tang, Y., Wu, D., Jiang, Z., Wang, J., & Wang, X. (2022). Prediction of thermo-mechanical properties of rubber-modified recycled aggregate concrete. Construction and Building Materials, 318, 125970. https://doi.org/10.1016/j.conbuildmat.2021.125970
- Gao, C., Huang, L., Yan, L., Jin, R., & Chen, H. (2020). Mechanical properties of recycled aggregate concrete modified by nano-particles. construction and Building Materials, 241, 118030. https://doi.org/10.1016/j.conbuildmat.2020.118030
- Gorbatsevich, O. B., Kholodkov, D. N., Kurkin, T. S., Malakhova, Y. N., Strel’tsov, D. R., Buzin, A. I., Kazakova, V. V., & Muzafarov, A. M. (2017, Mar). Synthesis and properties of water-soluble silica nanoparticles. russian Chemical Bulletin, 66(3), 409–417. https://doi.org/10.1007/s11172-017-1748-1
- Habeb Abdulrazzak, F., Fadel Alkiam, A., & Hasan Hussein, F. (2019). Behavior of X-ray analysis of carbon nanotubes. In Perspective of Carbon Nanotubes. IntechOpen.
- Han, C., Hu, Y., Wang, K., & Luo, G. (2019, Nov). Preparation and in-situ surface modification of CaCO3 nanoparticles with calcium stearate in a microreaction system. Powder Technology, 356, 414–422. https://doi.org/10.1016/j.powtec.2019.08.054
- Haruehansapong, S., Pulngern, T., & Chucheepsakul, S. (2017). Effect of nanosilica particle size on the water permeability, abrasion resistance, drying shrinkage, and repair work properties of cement mortar containing Nano-SiO 2. Advances in Materials Science and Engineering, 2017, 1–11. https://doi.org/10.1155/2017/4213690
- Hassan, M. M., Dylla, H., Mohammad, L. N., & Rupnow, T. (2012). Methods for the application of titanium dioxide coatings to concrete pavement. Int. J. Pavement Res. Technol, 5 (1), 12–20. [Online]. Available https://trid.trb.org/view/1128493
- Hosseini, P., Booshehrian, A., Delkash, M., Ghavami, S., & Zanjani, M. K. (2009). Use of nano-SiO2 to improve microstructure and compressive strength of recycled aggregate concretes. Nanotechnol. Constr, 3, 215–221. https://doi.org/10.1007/978-3-642-00980-829
- Hosseini, P., Booshehrian, A., & Madari, A. (2011). Developing concrete recycling strategies by utilization of nano-SiO 2 particles. Waste and Biomass Valorization, 2(3), 347–355. https://doi.org/10.1007/s12649-011-9071-9
- Hou, P., Qian, J., Cheng, X., & Shah, S. P. (2015, Jan). Effects of the pozzolanic reactivity of nanoSiO2 on cement-based materials. Cement and Concrete Composites, 55, 250–258. https://doi.org/10.1016/J.CEMCONCOMP.2014.09.014
- Hussein, A. I., Ab-Ghani, Z., Mat, A. N. C., Ghani, N. A. A., Husein, A., & Rahman, I. A. (2020). Synthesis and characterization of spherical calcium carbonate nanoparticles derived from cockle shells. applied Sciences, 10(20), 1–14. https://doi.org/10.3390/app10207170
- Ismail, S., Kwan, W. H., & Ramli, M. (2017). Mechanical strength and durability properties of concrete containing treated recycled concrete aggregates under different curing conditions. construction and Building Materials, 155, 296–306. https://doi.org/10.1016/j.conbuildmat.2017.08.076
- Ismail, S., & Mahyuddin, R. (2014). A study on the effect of surface-treated coarse recycled concrete aggregate (RCA) on the compressive strength of concrete. advanced Materials Research, 935(8), 184–187. https://doi.org/10.4028/www.scientific.net/AMR.935.184
- Jaishankar, P., & Karthick Raja, R. (2019). Micro structure properties of parent concrete with nano silica and polypropylene fibre in recycled aggregate. Int. J. Recent Technol. Eng, 8(3), 4949–4953. https://doi.org/10.35940/ijrte.C5610.098319
- Kawashima, S., Seo, J. W. T., Corr, D., Hersam, M. C., & Shah, S. P. (2014). Dispersion of CaCO3 nanoparticles by sonication and surfactant treatment for application in fly ash-cement systems. materials and Structures, 47(6), 1011–1023. https://doi.org/10.1617/s11527-013-0110-9
- Kou, S. C., & Poon, C. S. (2010, Sep). Properties of concrete prepared with PVA-impregnated recycled concrete aggregates. cement and Concrete Composites, 32(8), 649–654. https://doi.org/10.1016/j.cemconcomp.2010.05.003
- Kou, S. C., & Poon, C. S. (2012, Oct). Enhancing the durability properties of concrete prepared with coarse recycled aggregate. construction and Building Materials, 35, 69–76. https://doi.org/10.1016/j.conbuildmat.2012.02.032
- Kou, S. C., & Poon, C. S. (2013). Long-term mechanical and durability properties of recycled aggregate concrete prepared with the incorporation of fly ash. cement and Concrete Composites, 37(1), 12–19. https://doi.org/10.1016/j.cemconcomp.2012.12.011
- Krishna, P. H., & Krishna, P. B. (2017). Effect of nano-silica and metakaolin on properties of recycled coarse aggregate concrete. International Journal of Advanced Engneering and Research Development, 4(1), 831. https://doi.org/10.21090/ijaerd.04015
- Krzemień, K., & Hager, I. (2015). Assessment of concrete susceptibility to fire spalling: A report on the state-of-the-art in testing procedures. Procedia Engineering, 108, 285–292. https://doi.org/10.1016/j.proeng.2015.06.149
- Kumar, R. (2017). Influence of recycled coarse aggregate derived from construction and demolition waste (CDW) on abrasion resistance of pavement concrete. Construction and Building Materials, 142, 248–255. https://doi.org/10.1016/j.conbuildmat.2017.03.077
- Lavergne, F., Belhadi, R., Carriat, J., & Ben Fraj, A. (2019, Jan). Effect of nano-silica particles on the hydration, the rheology and the strength development of a blended cement paste. Cement and Concrete Composites, 95, 42–55. https://doi.org/10.1016/j.cemconcomp.2018.10.007
- Lee, G. C., & Choi, H. B. (2013). Study on interfacial transition zone properties of recycled aggregate by micro-hardness test. construction and Building Materials, 40, 455–460. https://doi.org/10.1016/j.conbuildmat.2012.09.114
- Liang, Z., Hu, Z., Zhou, Y., Wu, Y., Zhou, X., Hu, B., & Guo, M. (2022, Jan). Improving recycled aggregate concrete by compression casting and nano-silica. Nanotechnology Reviews, 11(1), 1273–1290. https://doi.org/10.1515/NTREV-2022-0065/ASSET/GRAPHIC/J_NTREV-2022-0065_FIG_013.JPG
- Liao, D. L., & Liao, B. Q. (2007). Shape, size and photocatalytic activity control of TiO2 nanoparticles with surfactants. Journal of Photochemistry and Photobiology A: Chemistry, 187(2–3), 363–369. https://doi.org/10.1016/j.jphotochem.2006.11.003
- Li, W., Long, C., Tam, V. W. Y., Poon, C. S., & Hui Duan, W. (2017, Jul). Effects of nano-particles on failure process and microstructural properties of recycled aggregate concrete. construction and Building Materials, 142, 42–50. https://doi.org/10.1016/j.conbuildmat.2017.03.051
- Li, W., Luo, Z., Long, C., Wu, C., Duan, W. H., & Shah, S. P. (2016). Effects of nanoparticle on the dynamic behaviors of recycled aggregate concrete under impact loading. Materials & Design, 112, 58–66. https://doi.org/10.1016/j.matdes.2016.09.045
- Li, L. J., Tu, G. R., Lan, C., & Liu, F. (2016). Mechanical characterization of waste-rubber-modified recycled-aggregate concrete. Journal of Cleaner Production, 124, 325–338. https://doi.org/10.1016/j.jclepro.2016.03.003
- Liu, Z., Cai, C. S., Peng, H., & Fan, F. (2016). Experimental study of the geopolymeric recycled aggregate concrete. Journal of Materials in Civil Engineering, 28(9), 1–9. https://doi.org/10.1061/(ASCE)MT.1943-5533.0001584
- Liu, J., Chen, H., Guan, B., Liu, K., Wen, J., & Sun, Z. (2018). Influence of mineral nano-fibers on the physical properties of road cement concrete material. Construction and Building Materials, 190, 287–293. https://doi.org/10.1016/j.conbuildmat.2018.09.025
- Liu, W., Cui, H., Dong, Z., Xing, F., Zhang, H., & Lo, T. Y. (2016). Carbonation of concrete made with dredged marine sand and its effect on chloride binding. Construction and Building Materials, 120, 1–9. https://doi.org/10.1016/j.conbuildmat.2016.05.011
- Liu, C., He, X., Deng, X., Wu, Y., Zheng, Z., Liu, J., & Hui, D. (2020). Application of nanomaterials in ultra-high performance concrete: A review. nanotechnology Reviews, 9(1), 1427–1444. https://doi.org/10.1515/ntrev-2020-0107
- Liu, S., Shen, P., Xuan, D., Li, L., Sojobi, A., Zhan, B., & Poon, C. S. (2021). A comparison of liquid-solid and gas-solid accelerated carbonation for enhancement of recycled concrete aggregate. Cement and Concrete Composites, 118, 103988. https://doi.org/10.1016/j.cemconcomp.2021.103988
- Li, J., Xiao, H., & Zhou, Y. (2009, Mar). Influence of coating recycled aggregate surface with pozzolanic powder on properties of recycled aggregate concrete. construction and Building Materials, 23(3), 1287–1291. https://doi.org/10.1016/j.conbuildmat.2008.07.019
- Li, L., Xuan, D., Chu, S. H., Lu, J. X., & Poon, C. S. (2021). Efficiency and mechanism of nano-silica pre-spraying treatment in performance enhancement of recycled aggregate concrete. Construction and Building Materials, 301, 124093. https://doi.org/10.1016/j.conbuildmat.2021.124093
- Li, L., Xuan, D., Sojobi, A. O., Liu, S., Chu, S. H., & Poon, C. S. (2021). Development of nano-silica treatment methods to enhance recycled aggregate concrete. Cement and Concrete Composites, 118(February), 103963. https://doi.org/10.1016/j.cemconcomp.2021.103963
- Makhloufi, Z., Bederina, M., Bouhicha, M., & Kadri, E. H. (2014). Effect of mineral admixtures on resistance to sulfuric acid solution of mortars with quaternary binders. Physics Procedia, 55, 329–335. https://doi.org/10.1016/j.phpro.2014.07.048
- Malešič, J., Kadivec, M., Kunaver, M., Skalar, T., & Cigić, I. K. (2019). Nano calcium carbonate versus nano calcium hydroxide in alcohols as a deacidification medium for lignocellulosic paper. heritage Science, 7(1), 1–14. https://doi.org/10.1186/s40494-019-0294-6
- Mansor, M. R., Fadzullah, S. H. S. M., Masripan, N. A. B., Omar, G., & Akop, M. Z., Comparison between functionalized graphene and carbon nanotubes: Effect of morphology and surface group on mechanical, electrical, and thermal properties of nanocomposites, in Functionalized Graphene Nanocomposites and Their Derivatives: Synthesis, Processing and Applications, Elsevier, 2018, pp. 177–204.
- Ma, Z., Tang, Q., Yang, D., & Ba, G. (2019). Durability studies on the recycled aggregate concrete in China over the past decade: A review. Advanced Civil Engneering, 2019. https://doi.org/10.1155/2019/4073130
- Meng, R. T., Meng, T., Huang, M. Z., & Xu, Q. L. (2013). Research on composite strengthening nano-technique of recycled aggregate. applied Mechanics and Materials, 357–360, 1189–1193. https://doi.org/10.4028/www.scientific.net/AMM.357-360.1189
- Meng, T., Yu, Y., & Wang, Z. (2017, May). Effect of nano-CaCO3 slurry on the mechanical properties and micro-structure of concrete with and without fly ash. Composites Part B: Engineering, 117, 124–129. https://doi.org/10.1016/j.compositesb.2017.02.030
- Meng, T., Zhang, J., Wei, H., & Shen, J. (2020). Effect of nano-strengthening on the properties and microstructure of recycled concrete. nanotechnology Reviews, 9(1), 79–92. https://doi.org/10.1515/ntrev-2020-0008
- Mirgozar Langaroudi, M. A., & Mohammadi, Y. (2018). Effect of nano-clay on workability, mechanical, and durability properties of self-consolidating concrete containing mineral admixtures. Construction and Building Materials, 191, 619–634. https://doi.org/10.1016/j.conbuildmat.2018.10.044
- Moffatt, E. G., & Thomas, M. D. A. (2017). Performance of rapid-repair concrete in an aggressive marine environment. Construction and Building Materials, 132, 478–486. https://doi.org/10.1016/j.conbuildmat.2016.12.004
- Mohamed, A. M. (2016, Aug). Influence of nano materials on flexural behavior and compressive strength of concrete. HBRC Journal, 12(2), 212–225. https://doi.org/10.1016/j.hbrcj.2014.11.006
- Mohammed, B. S., & Adamu, M. (2018). Mechanical performance of roller compacted concrete pavement containing crumb rubber and nano silica. Construction and Building Materials, 159, 234–251. https://doi.org/10.1016/j.conbuildmat.2017.10.098
- Moro, C., Francioso, V., & Velay-Lizancos, M. (2020). Nano-TiO2 effects on high temperature resistance of recycled mortars. Journal of Cleaner Production, 263(x), 121581. https://doi.org/10.1016/j.jclepro.2020.121581
- Muduli, R., & Mukharjee, B. B. (2019). Effect of incorporation of metakaolin and recycled coarse aggregate on properties of concrete. Journal of Cleaner Production, 209, 398–414. https://doi.org/10.1016/j.jclepro.2018.10.221
- Mukharjee, B. B., & Barai, S. V. (2014a). Influence of incorporation of nano-silica and recycled aggregates on compressive strength and microstructure of concrete. Construction and Building Materials, 71, 570–578. https://doi.org/10.1016/j.conbuildmat.2014.08.040
- Mukharjee, B. B., & Barai, S. V. (2014b). Influence of nano-silica on the properties of recycled aggregate concrete. construction and Building Materials, 55, 29–37. https://doi.org/10.1016/j.conbuildmat.2014.01.003
- Mukharjee, B. B., & Barai, S. V. (2014c). Statistical techniques to analyze properties of nano-engineered concrete using recycled coarse aggregates. Journal of Cleaner Production, 83, 273–285. https://doi.org/10.1016/j.jclepro.2014.07.045
- Mukharjee, B. B., & Barai, S. V. (2015). Characteristics of sustainable concrete incorporating recycled coarse aggregates and colloidal nano-silica. advances in Concrete Construction, 3(3), 187–202. https://doi.org/10.12989/acc.2015.3.3.187
- Mukharjee, B. B., & Barai, S. V. (2017). Mechanical and microstructural characterization of recycled aggregate concrete containing silica nanoparticles. J. Sustain. Cem. Mater, 6(1), 37–53. https://doi.org/10.1080/21650373.2016.1230899
- Murthi, P., Poongodi, K., Awoyera, P. O., Gobinath, R., & Saravanan, R. (2020). Enhancing the strength properties of high-performance concrete using ternary blended cement: OPC, nano-silica, bagasse ash. Silicon, 12(8), 1949–1956. https://doi.org/10.1007/s12633-019-00324-0
- Nikbin, I. M., Mohebbi, R., Dezhampanah, S., Mehdipour, S., Mohammadi, R., & Nejat, T. (2019, Sep). Gamma ray shielding properties of heavy-weight concrete containing nano-TiO2. radiation Physics and Chemistry, 162, 157–167. https://doi.org/10.1016/j.radphyschem.2019.05.008
- Niroumand, H., Zain, M. F. M., & Alhosseini, S. N. (2013). The influence of nano-clays on compressive strength of earth bricks as sustainable materials. Procedia - Social and Behavioral Sciences, 89, 862–865. https://doi.org/10.1016/j.sbspro.2013.08.945
- Nuaklong, P., Jongvivatsakul, P., Pothisiri, T., Sata, V., & Chindaprasirt, P. (2020). Influence of rice husk ash on mechanical properties and fire resistance of recycled aggregate high-calcium fly ash geopolymer concrete. Journal of Cleaner Production, 252, 119797. https://doi.org/10.1016/j.jclepro.2019.119797
- Nuaklong, P., Sata, V., Wongsa, A., Srinavin, K., & Chindaprasirt, P. (2018). Recycled aggregate high calcium fly ash geopolymer concrete with inclusion of OPC and nano-SiO2. construction and Building Materials, 174, 244–252. https://doi.org/10.1016/j.conbuildmat.2018.04.123
- Ossa, A., García, J. L., & Botero, E. (2016, Nov). Use of recycled construction and demolition waste (CDW) aggregates: A sustainable alternative for the pavement construction industry. Journal of Cleaner Production, 135, 379–386. https://doi.org/10.1016/j.jclepro.2016.06.088
- Ouyang, K., Shi, C., Chu, H., Guo, H., Song, B., Ding, Y., Guan, X., Zhu, J., Zhang, H., Wang, Y., & Zheng, J. (2020). An overview on the efficiency of different pretreatment techniques for recycled concrete aggregate. Journal of Cleaner Production, 263, 121264. https://doi.org/10.1016/j.jclepro.2020.121264
- Pacheco-Torgal, F., & Jalali, S. (2011). Nanotechnology: Advantages and drawbacks in the field of construction and building materials. Construction and Building Materials, 25(2), Elsevier, 582–590. https://doi.org/10.1016/j.conbuildmat.2010.07.009
- Pedro, D., de Brito, J., & Evangelista, L. (2017). Structural concrete with simultaneous incorporation of fine and coarse recycled concrete aggregates: Mechanical, durability and long-term properties. Construction and Building Materials, 154, 294–309. https://doi.org/10.1016/j.conbuildmat.2017.07.215
- Pyo, S., Abate, S. Y., & Kim, H. K. (2018). Abrasion resistance of ultra high performance concrete incorporating coarser aggregate. Construction and Building Materials, 165, 11–16. https://doi.org/10.1016/j.conbuildmat.2018.01.036
- Ragab, A. E.-R. (2019). Physico-chemical properties of nano metakaolin on the characteristics of blended limestone cement. Journal of Building Pathology and Rehabilitation, 4(1), 1–10. https://doi.org/10.1007/s41024-019-0048-6
- Rao, K. J., Keerthi, K., & Vasam, S. (2018). Acid resistance of quaternary blended recycled aggregate concrete. Case Studies in Construction Materials, 8(January), 423–433. https://doi.org/10.1016/j.cscm.2018.03.005
- Reales, O. A. M., Jaramillo, Y. P. A., Ocampo, C., Botero, J. C. O., Quintero, J. H., & Filho, R. D. T. (2021, Sep). Anionic, cationic, and nonionic surfactants used as dispersing agents for carbon nanotubes and their effect on cement hydration. Journal of Materials in Civil Engineering, 33(11), 04021325. https://doi.org/10.1061/(ASCE)MT.1943-5533.0003955
- Ren, W., Han, S., Li, J., & Liu, M. (2017, Jul). Investigation of the relative abrasion resistance of concrete pavement with chip-sprinkled surfaces. Wear, 382-383, 95–101. https://doi.org/10.1016/j.wear.2017.04.011
- Rizvi, R., Tighe, S., Henderson, V., & Norris, J. (2010). Evaluating the use of recycled concrete aggregate in pervious concrete pavement. Transportation Research Record: Journal of the Transportation Research Board, 2164(2164), 132–140. https://doi.org/10.3141/2164-17
- Rossen, J. E., & Scrivener, K. L. (2017, Jan). Optimization of SEM-EDS to determine the C–A–S–H composition in matured cement paste samples. Materials Characterization, 123, 294–306. https://doi.org/10.1016/j.matchar.2016.11.041
- Sadati, S., & Khayat, K. H. (2016). Field performance of concrete pavement incorporating recycled concrete aggregate. Construction and Building Materials, 126, 691–700. https://doi.org/10.1016/j.conbuildmat.2016.09.087
- Sadeghi-Nik, A., Berenjian, J., Alimohammadi, S., Lotfi-Omran, O., Sadeghi-Nik, A., & Karimaei, M. (2019). The effect of recycled concrete aggregates and metakaolin on the mechanical properties of self-compacting concrete containing nanoparticles. Iranian Journal of Science and Technology, Transactions of Civil Engineering, 43(S1), 503–515. https://doi.org/10.1007/s40996-018-0182-4
- Salkhordeh, S., Golbazi, P., & Amini, H. (2011). The improvement of 28-day compressive strength of self compacting concrete made by different percentages of recycled concrete aggregates using nano-silica. World Academy of Science, Engineering and Technology, 59(11), 874–877.
- Sanchez, F., Kosson D, Brown K, Delapp R, Teising R, Gonzalez R, Lewis J, Brown L, Reches Y, Helbing M, Thomson K. (2018). Development of nano-modified concrete for next generation of storage systems. https://doi.org/10.2172/1469196
- Santha Kumar, G., Saini, P. K., Karade, S. R., & Minocha, A. K. (2019, Sep). Chemico-thermal treatment for quality enhancement of recycled concrete fine aggregates. Journal of Material Cycles and Waste management, 21(5), 1197–1210. https://doi.org/10.1007/s10163-019-00874-w
- Sasanipour, H., Aslani, F., & Taherinezhad, J. (2021). Chloride ion permeability improvement of recycled aggregate concrete using pretreated recycled aggregates by silica fume slurry. Construction and Building Materials, 270, 121498. https://doi.org/10.1016/j.conbuildmat.2020.121498
- Scott, L. T. (2017). Methods for the chemical synthesis of carbon nanotubes: An approach based on hemispherical polyarene templates. Pure and Applied Chemistry, Jun, 89(6), 809–820. https://doi.org/10.1515/pac-2016-1222
- Shaban, W. M., Yang, J., Su, H., Liu, Q.-F., Tsang, D. C. W., Wang, L., Xie, J., & Li, L. (2019). Properties of recycled concrete aggregates strengthened by different types of pozzolan slurry. Construction and Building Materials, 216, 632–647. https://doi.org/10.1016/j.conbuildmat.2019.04.231
- Shafiu Kamba, A., Ismail, M., Tengku Ibrahim, T. A., & Zakaria, Z. A. B. (2013). Synthesis and characterisation of calcium carbonate aragonite nanocrystals from cockle shell powder (Anadara granosa),”. Journal of Nanomaterials, 2013, 1–9. https://doi.org/10.1155/2013/398357
- Shaikh, F., Chavda, V., Minhaj, N., & Arel, H. S. (2018). Effect of mixing methods of nano silica on properties of recycled aggregate concrete. Structural Concrete, 19(2), 387–399. https://doi.org/10.1002/suco.201700091
- Shaikh, F. U. A., Odoh, H., & Than, A. B. (2015). Effect of nano silica on properties of concretes containing recycled coarse aggregates. Proceedings of the Institution of Civil Engineers - Construction Materials, 168(2), 68–76. https://doi.org/10.1680/coma.14.00009
- Sharma, S., & Arora, S. (2018). Economical graphene reinforced fly ash cement composite made with recycled aggregates for improved sulphate resistance and mechanical performance. Construction and Building Materials, 162, 608–612. https://doi.org/10.1016/j.conbuildmat.2017.12.027
- Shi, C., Wu, Z., Cao, Z., Ling, T. C., & Zheng, J. (2018). Performance of mortar prepared with recycled concrete aggregate enhanced by CO2 and pozzolan slurry. Cement and Concrete Composites, 86, 130–138. https://doi.org/10.1016/j.cemconcomp.2017.10.013
- Silva, R. V., de Brito, J., & Dhir, R. K. (2019, Nov). Use of recycled aggregates arising from construction and demolition waste in new construction applications. Journal of Cleaner Production, 236, 117629. https://doi.org/10.1016/J.JCLEPRO.2019.117629
- Singh, L. P., Ali, D., Tyagi, I., Sharma, U., Singh, R., & Hou, P. (2019). Durability studies of nano-engineered fly ash concrete. consTruction and Building Materials, 194, 205–215. https://doi.org/10.1016/j.conbuildmat.2018.11.022
- Singh, L. P., Bisht, V., Aswathy, M. S., Chaurasia, L., & Gupta, S. (2018). Studies on performance enhancement of recycled aggregate by incorporating bio and nano materials. Construction and Building Materials, 181, 217–226. https://doi.org/10.1016/j.conbuildmat.2018.05.248
- Staub de Melo, J. V., & Trichês, G. (2018). Study of the influence of nano-TiO 2 on the properties of Portland cement concrete for application on road surfaces. Road Materials and Pavement Design, 19(5), 1011–1026. https://doi.org/10.1080/14680629.2017.1285811
- Su, Y., Li, J., Wu, C., Wu, P., & Li, Z. X. (2016). Influences of nano-particles on dynamic strength of ultra-high performance concrete. Composites Part B: Engineering, 91, 595–609. https://doi.org/10.1016/j.compositesb.2016.01.044
- Tam, V. W. Y., & Tam, C. M. (2008, Oct). Diversifying two-stage mixing approach (TSMA) for recycled aggregate concrete: TSMAs and TSMAsc. Construction and Building Materials, 22(10), 2068–2077. https://doi.org/10.1016/j.conbuildmat.2007.07.024
- Tam, V. W. Y., Tam, C. M., & Le, K. N. (2007, Mar). Removal of cement mortar remains from recycled aggregate using pre-soaking approaches. Resources, Conservation and Recycling, 50(1), 82–101. https://doi.org/10.1016/j.resconrec.2006.05.012
- Tavakoli, D. (2014). Properties of concrete made with waste clay brick as sand incorporating nano SiO2. Indian Journal of Science and Technology, 7(12), 1899–1905. https://doi.org/10.17485/ijst/2014/v7i12.1
- Tavakoli, D., Sakenian Dehkordi, R., Divandari, H., & de Brito, J. (2020). Properties of roller-compacted concrete pavement containing waste aggregates and nano SiO2. Construction and Building Materials, 249, 118747. https://doi.org/10.1016/j.conbuildmat.2020.118747
- Tayeh, B. A., Al Saffar, D. M., & Alyousef, R. (2020, Jul). The utilization of recycled aggregate in high performance concrete: A review. Journal of Materials Research and Technology, 9(4), 8469–8481. https://doi.org/10.1016/J.JMRT.2020.05.126
- Thomas, C., Setién, J., Polanco, J. A., Alaejos, P., & Sánchez De Juan, M. (2013). Durability of recycled aggregate concrete. Construction and Building Materials, 40, 1054–1065. https://doi.org/10.1016/j.conbuildmat.2012.11.106
- Thomas, J., Thaickavil, N. N., & Wilson, P. M. (2018). Strength and durability of concrete containing recycled concrete aggregates. Journal of Building Engineering, 19, 349–365. https://doi.org/10.1016/j.jobe.2018.05.007
- Toghroli, A., Mehrabi, P., Shariati, M., Trung, N. T., Jahandari, S., & Rasekh, H. (2020). Evaluating the use of recycled concrete aggregate and pozzolanic additives in fiber-reinforced pervious concrete with industrial and recycled fibers. Construction and Building Materials, 252, 118997. https://doi.org/10.1016/j.conbuildmat.2020.118997
- Uddin, F. (2008). Clays, nanoclays, and montmorillonite minerals. Metallurgical and Materials Transactions A, 39(12), 2804–2814. https://doi.org/10.1007/s11661-008-9603-5
- Umasabor, R. I., & Okovido, J. O. (2018). Fire resistance evaluation of rice husk ash concrete. Heliyon, 4(12), e01035. https://doi.org/10.1016/j.heliyon.2018.1035
- Vasanthi, P., & Senthil Selvan, S. (2020). Study on mechanical performance of recycled aggregate concrete with modified nano silica in cement. International Journal of Advanced Science and Technology, 29(7), 1060–1069.
- Vinay Kumar, B. M., Ananthan, H., & Balaji, K. V. A. (2018). Experimental studies on utilization of recycled coarse and fine aggregates in high performance concrete mixes. Alexandria engineering Journal, 57(3), 1749–1759. https://doi.org/10.1016/j.aej.2017.05.003
- Wang, X., Cheng, F., Wang, Y., Zhang, X., & Niu, H. (2020). Impact properties of recycled aggregate concrete with nanosilica modification. Adv. Civ. Eng, 2020. https://doi.org/10.1155/2020/8878368
- Wang, Y., Hughes, P., Niu, H., & Fan, Y. (2019). A new method to improve the properties of recycled aggregate concrete: Composite addition of basalt fiber and nano-silica. Journal of Cleaner Production, 236, 2019. https://doi.org/10.1016/j.jclepro.2019.07.077
- Wu, C., Ma, W., Li, Y., & Chen, Y. (2014). Nano materials and its application in space. Trans Tech Publication, 482, 34–37. https://doi.org/10.4028/www.scientific.net/AMM.482.34
- Xiao, J., Li, L., Shen, L., & Poon, C. S. (2015). Compressive behaviour of recycled aggregate concrete under impact loading. Cement and Concrete Research, 71, 46–55. https://doi.org/10.1016/j.cemconres.2015.01.014
- Xie, J., Zhang, H., Duan, L., Yang, Y., Yan, J., Shan, D., Liu, X., Pang, J., Chen, Y., Li, X., & Zhang, Y. (2020). Effect of nano metakaolin on compressive strength of recycled concrete. Construction and Building Materials, 256, 119393. https://doi.org/10.1016/j.conbuildmat.2020.119393
- Xu, Y. et al. (2020). Studying the mix design and investigating the photocatalytic performance of pervious concrete containing TiO2-Soaked recycled aggregates. Journal of Cleaner Production, 248, 119281. https://doi.org/10.1016/j.jclepro.2019.119281
- Xu, S., Liu, J., & Li, Q. (2015, Feb). Mechanical properties and microstructure of multi-walled carbon nanotube-reinforced cement paste. Construction and Building Materials, 76, 16–23. https://doi.org/10.1016/j.conbuildmat.2014.11.049
- Ying, J., Zhou, B., & Xiao, J. (2017). Pore structure and chloride diffusivity of recycled aggregate concrete with nano-SiO2 and nano-TiO2. construction and Building Materials, 150, 49–55. https://doi.org/10.1016/j.conbuildmat.2017.05.168
- Yonggui, W., Shuaipeng, L., Hughes, P., & Yuhui, F. (2020). Mechanical properties and microstructure of basalt fibre and nano-silica reinforced recycled concrete after exposure to elevated temperatures. Construction and Building Materials, 247, 118561. https://doi.org/10.1016/j.conbuildmat.2020.118561
- Younis, K. H., & Mustafa, S. M. (2018). Feasibility of using nanoparticles of SiO 2 to improve the performance of recycled aggregate concrete. Advances in Materials Science and Engineering, 2018, 3–5. https://doi.org/10.1155/2018/1512830
- Yue, Y., Zhou, Y., Xing, F., Gong, G., Hu, B., & Guo, M. (2020). An industrial applicable method to improve the properties of recycled aggregate concrete by incorporating nano-silica and micro-CaCO3. Journal of Cleaner Production, 259, 120920. https://doi.org/10.1016/j.jclepro.2020.120920
- Yunchao, T., Zheng, C., Wanhui, F., Yumei, N., Cong, L., & Jieming, C. (2021, Jan). Combined effects of nano-silica and silica fume on the mechanical behavior of recycled aggregate concrete. Nanotechnology Reviews, 10(1), 819–838. https://doi.org/10.1515/NTREV-2021-0058/ASSET/GRAPHIC/J_NTREV-2021-0058_FIG_019.JPG
- Yuvaraj, S., & Anish, V. (2019). Strength properties of nano silica recycled sustainable concrete. International Journal of Engineering and Innovative Technology, 8(10), 2245–2248. https://doi.org/10.35940/ijitee.I8954.0881019
- Zeng, W., Zhao, Y., Zheng, H., & Sun Poon, C. (2019). Improvement in corrosion resistance of recycled aggregate concrete by nano silica suspension modification on recycled aggregates. Cement and Concrete Composites, 106(November), 103476. https://doi.org/10.1016/j.cemconcomp.2019.103476
- Zhang, P., Wan, J., Wang, K., & Li, Q. (2017, Sep). Influence of nano-SiO 2 on properties of fresh and hardened high performance concrete: A state-of-the-art review. Construction and Building Materials, 148, 648–658. https://doi.org/10.1016/j.conbuildmat.2017.05.059
- Zhang, H., Zhao, Y., Meng, T., & Shah, S. P. (2015). The modification effects of a nano-silica slurry on microstructure, strength, and strain development of recycled aggregate concrete applied in an enlarged structural test. Construction and Building Materials, 95, 721–735. https://doi.org/10.1016/j.conbuildmat.2015.07.089
- Zhang, H., Zhao, Y., Meng, T., & Shah, S. P. (2016). Surface treatment on recycled coarse aggregates with nanomaterials. Journal of Materials in Civil Engineering, 28(2), 1–11. https://doi.org/10.1061/(ASCE)MT.1943-5533.0001368
- Zhan, B. J., Xuan, D. X., Zeng, W., & Poon, C. S. (2019, Nov). Carbonation treatment of recycled concrete aggregate: Effect on transport properties and steel corrosion of recycled aggregate concrete. Cement and Concrete Composites, 104, 103360. https://doi.org/10.1016/j.cemconcomp.2019.103360
- Zhao, H., Wang, Y., & Liu, F. (2017, Jul). Stress–strain relationship of coarse RCA concrete exposed to elevated temperatures. Magazine of Concrete Research, 69(13), 649–664. https://doi.org/10.1680/jmacr.16.00333
- Zheng, Y., Zhuo, J., & Zhang, P. (2021, Oct). A review on durability of nano-SiO2 and basalt fiber modified recycled aggregate concrete. Construction and Building Materials, 304, 124659. https://doi.org/10.1016/J.CONBUILDMAT.2021.124659
- Zhou, Y., Gao, H., Hu, Z., Qiu, Y., Guo, M., Huang, X., & Hu, B. (2021). Ductile, durable, and reliable alternative to FRP bars for reinforcing seawater sea-sand recycled concrete beams: Steel/FRP composite bars. Construction and Building Materials, 269(xxxx), 121264. https://doi.org/10.1016/j.conbuildmat.2020.121264
- Ziental, D., Czarczynska-Goslinska, B., Mlynarczyk, D. T., Glowacka-Sobotta, A., Stanisz, B., Goslinski, T., & Sobotta, L. (2020). Titanium dioxide nanoparticles: Prospects and applications in medicine. Nanomaterials, 10(2), 387. https://doi.org/10.3390/nano10020387