Abstract
Bacterium Paenibacillus mucilaginosus strain N3 was isolated from agricultural farm soil (located at Boriavi village, Gujarat, India). Isolate showed an evidence of non-symbiotic nitrogen fixation, when grown in nitrogen-free bromothymol blue growth medium. It was tested positive for direct plant-growth-promoting traits like Indole-3-acetic acid production, solubilization of Tri-calcium-phosphate, and ammonia production. Further, N3 isolate was tested positive for siderophore production of catecholate type and catalase production as an indirect plant-growth-promoting trait. Biochemical tests along with 16s rRNA gene sequence analysis confirmed the strain N3 to be P. mucilaginosus. To determine its efficacy as a plant-growth-promoting rhizobacteria (PGPR), its talc-based biofertilizer was prepared and tested on the growth of green gram (Vigna radiata). Seeds treated with this biofertilizer showed an increase in overall dry biomass by 17% and sapling length by 28% (as compared to non-treated controls) after 10 days of sowing in pots. Thus, multiple plant-growth-promoting traits of P. mucilaginosus N3 determined in vitro along with its ability to promote growth in green gram in vivo we characterize this strain as an efficient PGPR.
Public Interest Statement
Commercially available microbial biofertilizers contain strains of bacteria which associate with the roots of the plant and imparts beneficial effect on plant. Such microbial strains possess symbiotic relation with plants. Most widely available biofertilizers contain nitrogen-fixing microbes like Rhizobium, Azotobacter, Azospirillum, Blue green Algae, or contain phosphate solubilizing strains of Pseudomonas and Bacillus. To improvise microbial biofertilizers, there is a need to isolate and characterize new microbial strains which are more efficient or possess more than one trait which aids growth promotion in plants. Thus, with this article, we describe isolation and characterization of new microbial strain possessing ability to enhance growth of the plant, such research will introduce new and efficient strains which can be used to develop more efficient biofertilizers.
1. Introduction
Paenibacillus genus was separated from Bacillus before 20 years by Ash, Priest, and Collins (Citation1993). They claimed that strains of Paenibacillus have dissimilarity in the consensus region of 16s rRNA as compared to strains of Bacillus. To our interest, species of Paenibacillus are similar to Bacillus in their action as plant-growth-promoting rhizobacteria (PGPR), but the nitrogen-fixing ability shown by some Paenibacillus strains provides them superiority. Characteristics that distinguish the genus Paenibacillus from other members of Bacillus includes, rod-shaped bacterial cells with a flagellum; producing ellipsoidal spores with swollen sporangia; positive for catalase; negative for H2S production; variable for oxidase; G + C content of their DNA ranging between 45% and 54 mol%, with anteiso-C15: 0 as the major cellular fatty acid, meso-diaminopimelic acid as the diagnostic diamino acid, and more than 89.6% intra-genus similarity in 16s rRNA gene sequences (Ash et al., Citation1993). Paenibacillus polymyxa (formerly Bacillus polymyxa) is most widely studied strain from this genus; it has ability to fix nitrogen and capable of producing antibiotic named polymyxin, and it is widely used as biocontrol agent for control of phytopathogens in agriculture (Eastman, Weselowski, Nathoo, & Yuan, Citation2014). Microbes are considered as a major resource for agricultural applications (Patel, Jha, Tank, & Saraf, Citation2011); however, strains other than P. polymyxa belonging to this genus are poorly studied for their role in agriculture.
Importance of microbes in agriculture become popular since late 1970s and such plant beneficial bacteria are termed as PGPR (Kloepper & Schroth, Citation1978). But genus Paenibacillus was proposed much later and described in 2nd edition of Bergey’s manual of systematic bacteriology which was published in 2004 (Naing et al., Citation2014). Since then there are limited reports describing strains of Paenibacillus as PGPR. Strains of Paenibacillus have not received comparable importance as efficient PGPR as strains of Acinetobacter, Agrobacterium, Arthobacter, Azotobacter, Sinorhizobium, Azospirillum, Burkholderia, Bradyrhizobium, Rhizobium, Frankia, Serratia, Thiobacillus, Pseudomonads, and Bacillus have received (Jacobson, Pasternak, & Glick, Citation1994; Sunar, Dey, Chakraborty, & Chakraborty, Citation2013; Vessey, Citation2003). However, over the period of last 10 years, few strains of Paenibacillus were rediscovered as nitrogen fixers which helped them to prove superiority over other extensively studied PGPR. Few nitrogen-fixing Paenibacillus strains include P. polymyxa, P. macerans, P. durus, P. peoriae, P. borealis, P. brasilensis, P. graminis, P. odorifer, P. wynnii, P. massiliensis, and P. sabinae (Beneduzi et al., Citation2010; Xie et al., Citation2014). Thus, there is a huge scope in isolating new stains of Paenibacillus and studying them for their plant-growth-promoting traits which can help them to characterize as cogent PGPR. Such study will help to introduce new and efficient strains for agricultural applications.
To portray any new strain as PGPR, it is necessary to know the mechanics employed by PGPR to enhance plant growth. The augmentative effect of PGPR occurs through various mechanisms which are primarily distinguished in the direct and indirect mechanisms. Direct mechanisms include production of plant hormones such as indole acetic acid (IAA), gibberellins, and cytokinins (Dey, Pal, Bhatt, & Chauhan, Citation2004; Patten & Glick, Citation1996) along with asymbiotic nitrogen fixation (Kennedy et al., Citation1997); and solubilization of phosphates (Banerjee & Yasmin, Citation2002; de Freitas, Banerjee, & Germida, Citation1997; Richardson, Citation2001). On the other hand, indirect mechanisms are the production of iron chelators, siderophores, and cyanides (Ahmad, Ahmad, & Khan, Citation2008; Cattelan, Hartel, & Fuhrmann, Citation1999; Goswami, Dhandhukia, Patel, & Thakker, Citation2014) since they act as antagonists to plant pathogens.
Under present study one such strain N3, capable of growing in nitrogen-free medium belonging to Paenibacillus genus was isolated from fertile agricultural soil. Strain was identified using 16s rRNA gene sequencing which showed high similarity with Paenibacillus mucilaginosus. The aim of the research was to portray this strain capable of growing in nitrogen-free growth medium, isolated from fertile rhizospheric soil as a PGPR. In-vitro biochemical analysis was performed to assess its multiple plant–growth-promoting traits which included ammonia production, phosphate solubilization, nitrogen fixation, IAA production, siderophore production, hydrogen cyanide (HCN) production, and catalase production. Plant-growth-promoting ability of strain N3 was in vivo determined by preparing talc-based biofertilizer and checking its effect on the seedling growth of green gram (Vigna radiata).
2. Materials and methods
2.1. Isolation of bacterial strain
The rhizosperic soil samples were collected from the agricultural field 15 cm below the soil surface, located at Boriavi village, Anand, Gujarat, India (22°61′N, 72°93′E). Soil sample was aseptically suspended in sterile distilled water and after allowing it to stand for an hour, supernatant was spread on nitrogen-free medium (Glucose: 50 g l−1, K2HPO4: 0.2 g l−1, NaCl: 0.2 g l−1, K2SO4: 0.1 g l−1, CaCO3: 5.0 g l−1, Agar: 25 g l−1, pH 6.8, prepared in distilled water) with appropriate dilution and the plates were incubated at 27°C for 48 h.
2.2. Identification of the organism
Morphological analyses were performed by using a standardized method (Murray, Doetsch, & Robinow, Citation1994). Biochemical tests described in Bergy’s Manual of Systematic Bacteriology were performed as per the methods described by Shi, Takano, and Liu (Citation2012). All the media and reagents required to perform biochemical tests were obtained from HiMedia.
For identification of bacterial isolate, 16s rRNA gene sequence was determined and analyzed. Genomic DNA from strain N3 was extracted and 16s rRNA gene was amplified using polymerase chain reaction using universal forward primer (5′-AGAGTTTGATCCTGGCTCAG-3′) and reverse primer (5′-AAGGAGGTGATCCAGCCGCA-3′) which were procured from 1st BASE (Agile Life Science Technologies India Pvt. Ltd) (Goswami, Dhandhukia, et al., Citation2014; Goswami, Pithwa, Dhandhukia, & Thakker, Citation2014). The reaction was carried out in a 50 μl mixture containing 1.5 mM MgCl2, 0.2 mM each dNTP, 25 pmol of forward and reverse primers, 50 ng DNA template, and 5 U Taq DNA polymerase with its reaction buffer. Reaction was performed for 34 cycles using thermocycler at 94°C for 45 s, 58°C for 45 s, and 72°C for 105 s followed by a final extension of 10 min at 72°C. Amplified gene product of (1.6 Kb) was sequenced at 1st BASE (Agile Life Science Technologies India Pvt. Ltd). The BLASTn search program (http://www.ncbi.nlm.nih.gov) was used for sequence homology analysis. The gene sequences were also submitted to GenBank and accession numbers were assigned. The sequence obtained was then aligned by ClustalW using MEGA 4.0 software (Tamura, Dudley, Nei, & Kumar, Citation2007) and a neighbor-joining (NJ) tree with bootstrap value 1,000 was generated using the software.
2.3. Qualitative and quantitative estimation of phosphate solubilization
Qualitative determination of phosphate solubilization was performed on Pikovskaya’s agar plate. Isolate was spot inoculated and incubated at 27 ± 2°C, and the size of the halo corresponding to phosphate solubilization was measured after 7 days of incubation. Quantitative estimation of tri-calcium phosphate solubilization was performed by growing the strain in Pikovaskya’s broth. The concentration of the soluble phosphate was determined from the culture supernatant at 10th, 15th, and 20th day after inoculation by stannous chloride method (King, Citation1932). Organic acid produced due to glucose metabolism (in Pikovaskya’s broth) was determined by monitoring the change in the pH of the medium.
2.4. IAA production
Indole-3-acetic acid (IAA) production was estimated using the method described by Bric, Bostock, and Silverstone (Citation1991). About 10% of exponentially grown culture of strain N3 was inoculated in 100 ml nitrogen-free medium with varying concentration of L-tryptophan which ranged from 0 to 500 μg ml−1 in different flasks. Bacterial cell-free supernatant was taken at 24, 48, and 72 h for performing quantative estimation of IAA. Briefly, 1 ml of cell-free supernatant was mixed vigorously with 1 ml of Salkowsky’s reagent (1 ml of 0.5 M FeCl3 in 50 ml of 35% HClO4) followed by the addition of two drops of orthophosphoric acid and assay system was kept at 27 ± 2°C in dark for 20 min till pink color developed. Optical density of this colored solution was measured spectrophotometrically at 535 nm. The concentration of IAA in each sample was determined from the standard curve of IAA ranging from 10 to 100 μg ml−1.
2.5. Siderophore estimation
Siderophore production was determined in defferated MM9 medium supplemented with 1% (w/v) Glucose, using CAS-shuttle assay (Payne, Citation1994). Strain N3 was grown in defferated MM9 medium with 1% (w/v) glucose. About 10 ml sample was withdrawn and centrifuged at 2,700 g for 15 min; 0.5 CAS assay solution was added to 0.5 ml of culture supernatant and mixed. This mixture was allowed to stand for 20 min. Siderophore (if present in the culture supernatant) will remove the iron from the dye complex, resulting in a loss of blue color of the solution. Decrease in the intensity of blue color was determined by measuring absorbance at 630 nm. Siderophore produced was calculated using the formula: [(A r − A s )/A r ] × 100 = % siderophore units.
where, A r is the absorbance of reference (minimal media + CAS assay solution) and A s is the absorbance of sample (culture supernatant + CAS assay solution).
For qualitative estimation of catecholate type of siderophores, 1 ml of culture supernatant was mixed with 1 ml of 0.5 N HCl, followed by the addition of 1 ml of sodium molybdate reagent (10 g of sodium nitrite and 10 g of sodium molybdate in 100 ml of distilled water), and allowed to stand for 15 min. To this mixture, 1 ml of 1 N NaOH was added. Pink color, if develops in this solution, indicates the presence of catecholate type of siderophores (Carson, Holliday, Glenn, & Dilworth, Citation1992).
2.6. Non-symbiotic nitrogen fixing ability and ammonia production
Nitrogen-fixing ability was qualitatively studied by allowing the strain N3 to grow in nitrogen-free bromothymol blue (NfB) medium (Sucrose: 10 g l−1, K2HPO4: 0.6 g l−1, MgSO4: 0.20 g l−1, NaCl: 0.2 g l−1, K2SO4: 0.1 g l−1, CaCO3: 2.0 g l−1, pH 6.8, 2.0 ml of 0.5% Bromothymol blue solution). Here, the strain possessing the ability to fix nitrogen will increase the pH of the medium which is determined by the color change of the medium from green to blue (Jha et al., Citation2010).
Ammonia production by the strain N3 was determined in peptone water broth. Briefly, strain N3 was inoculated into the medium and was allowed to grow at 27 ± 2°C, where the assay for ammonia production was performed for 4 days at an interval of every 24 h. Broth was collected, centrifuged, and the amount of ammonia in the supernatant was estimated by means of Nesslerization reaction where, 1 ml Nessler’s reagent was added to 1 ml of supernatant and volume of this mixture was made up to 10 ml by addition of ammonia-free distilled water. Development of brown to yellow color indicated positive result for ammonia production and its optical density was measured by spectrophotometer at 450 nm (Cappucino & Sherman, Citation1992). The concentration of ammonia was estimated based on a standard curve of ammonium sulfate ranging from 0.1 to 1 μmol ml−1.
2.7. Catalase test
Presence of catalase was checked qualitatively using the method described by Goswami, Patel, et al. (Citation2014). Solution of 6% H2O2 was added on the colonies grown on nitrogen-free agar plates; effervescences of O2 released from the bacterial colonies indicate the presence of catalase activity.
2.8. Preparation of talc-based biofertilizer and seed bacterization
The talc-based formulations of N3 isolate was prepared by the method described by Goswami, Vaghela, Parmar, Dhandhukia, and Thakker (Citation2013). Briefly, talc powder was taken in a sterilized metal tray and its pH was adjusted to neutral by addition of 15 g of CaCO3 per kg of talc. To this, 10 g of CMC was added and the mixture was autoclaved. About 400 ml of 48 h grown bacterial suspension was mixed with carrier-cellulose mixture under aseptic conditions. After drying (approximately 35% moisture content) overnight under sterile conditions, it was used for seed bacterization. Slurry of talc-based biofertilizer was prepared and surface-sterilized seeds (seeds dipped in 70% ethanol for 3 min and rinsed with sterile distilled water) were soaked overnight for the biofertilizer to get coated on seeds. Efficacy of biofertilizer was tested on wild-type seeds of green gram (V. radiata). These seeds treated with biofertilizer were sown in the pots sized (10 × 6 cm) and the growth parameters of seedlings were analyzed. All the pot experiments were carried out during the month of May where the average temperature was 37.0 ± 2.0°C and average humidity was 45%. Further, the soil used for the growth for chick pea and green gram was tested for its physical and chemical properties. Physical attributed Sieve analysis and Atterberg limit (Plastic limit, Liquid Limit and Plasticity Index; Dhandhukia, Goswami, Thakor, & Thakker, Citation2013). For chemical properties, total nitrogen, conductivity, TDS, pH, phosphates, sodium, potassium, calcium and magnesium were estimated from the soil sample (Ibekwe, Poss, Grattan, Grieve, & Suarez, Citation2010).
2.9. Statistical analysis
After the germination of test plants, the difference resulted by the biofertilizer treatment on the seedling growth was analyzed using statistical analysis. For this, analysis of variance (ANOVA) was carried out using triplicate value to identify significant difference in each vegetative parameter between treated and non-treated seeds. Mean values of triplicates were compared at significance levels of 5 and 1%.
3. Results
3.1. Isolation of bacterial strain
From the soil sample used to isolate bacterial strains on nitrogen-free medium, total three types of colonies were obtained. The most dominant strain growing on the minimal medium was isolated and purified. Strain was designated as N3, where “N” stands for ability of the strain to grow in absence of nitrogen. Colonies were big, round, entire, transparent, colorless, sticky, and odorless. Image of colonies of strain N3 growing on nitrogen-free agar medium is shown in Figure .
3.2. Identification of the organism
Microscopic observation of strain N3 showed that it was gram negative and produced large capsules. Strain showed optimum growth at 30°C, with constant agitation at neutral pH of the growth medium. Biochemical tests showed that strain N3 was positive for oxidase, catalase, and nitrite reduction test; however, strain was found negative for amylase, gelatinase, urease, indole production, methyl-red test, and Vogus–Proskauer (VP) test. Strain showed acid production when grown with glucose, galactose, glycerol, sucrose, and mannitol; whereas, fructose, lactose, starch, sorbitol, maltose, citrate were not utilized by the strain (Table ).
Table 1. Biochemical characterization of P. mucilaginosus strain N3
Phylogenetic analysis was done by comparing 16s rRNA gene sequence of N3 and the sequences of other type strains of genus Paenibacillus from GenBank to identify the isolated strain. Accession numbers of Paenibacillus type strains were obtained from “Bergey’s Manual of Systematic Bacteriology, Second Edition, Volume Three: The Firmicutes” to construct phylogenetic tree. Phylogenetic analysis showed that 16s rRNA gene sequence of strain N3 matched best with gene sequence of type strain P. mucilaginosus AS1.231T under GenBank ID: DQ898308 (Figure ). Based on biochemical characterization and phylogenetic analysis, strain N3 was designated as P. mucilaginosus strain N3and its 16s rRNA gene sequence was submitted to nucleotide sequence database under GenBank ID: JX154206.
Figure 2. The phylogenetic analysis based on 16s rRNA gene sequences available from European Molecular Biology Laboratory (EMBL) library constructed after multiple alignments of data by ClustalX
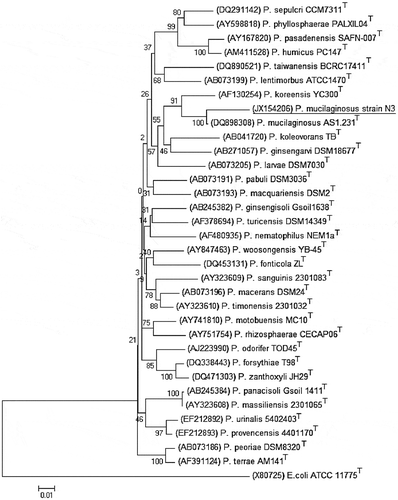
3.3. Estimation of phosphate solubilization
It was observed that strain N3 could solubilize phosphate on Pikovaskya’s agar where the zone diameter of phosphate solubilization was 20 mm and diameter of spot inoculant was 12 mm after its growth for 7 days. Quantitative estimation of phosphate solubilization was carried out in liquid Pikovaskya’s medium which contained glucose as a carbon source. Here, quantative estimation of phosphate solubilized was determined at 10th, 15th, and 20th day of its growth. Strain N3 solubilized maximum of 11 μg ml−1 of tri calcium phosphate after 15 days of incubation, though on 20th day the determined phosphate solubilized was less than 11 μg ml−1 which may be due to utilization of solubilized phosphate by the strain. Analysis of pH altered in the growth medium by the bacterial metabolism suggested that the pH decreases from neutral to acidic due to organic acid production as a product of glucose metabolism (Figure ).
3.4. IAA production
It was observed that strain N3 could produce IAA only when L-tryptophan was supplemented in the medium. IAA production by N3 isolate was determined at an interval of every 24 h after incubation (Figure ). Maximum IAA produced was 13 μg ml−1 after 72 h of incubation when L-tryptophan concentration in the medium was maximum (500 μg ml−1). In the growth medium with absence of L-tryptophan, IAA was not detected even after 72 h. This shows that there is a direct correlation in IAA production and supplemented L-tryptophan in the medium.
3.5. Siderophore and catalase production
Strain N3 showed production of siderophore in the iron-free MM9 minimal medium supplemented with 1% glucose which was detected by CAS-Shuttle assay. Maximum siderophore produced was 32% units (Figure ). The culture supernatant when mixed with CAS solution, its inherent blue color was gradually lost which indicated presence of siderophore (Figure (a)). Further, it was found that strain N3 could produce catecholate type of siderophores which was determined by the reaction of culture supernatant with sodium molybdate reagent under standard reaction condition produced pink color in the solution (Figure (b)). Strain N3 was also found to produce catalase as strong effervescences of O2 evolved when 6% H2O2 solution was flooded on the colonies grown on nitrogen-free agar.
Figure 5. Production of ammonia in peptone water broth and siderophore production in iron-free MM9 medium supplemented with 1% glucose
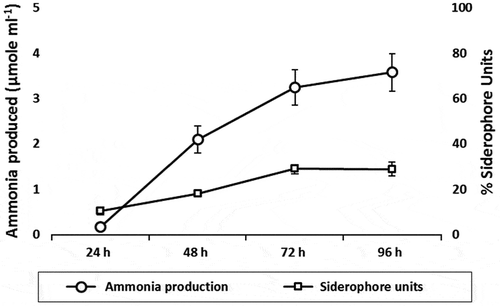
Figure 6. (a) The image of CAS assay for detection of siderophores, where when CAS dye is mixed with culture supernatant loses its blue color. (b) The production of catecholate type of siderophore in the growth medium (MM9 with 1% (w/v) glucose) when culture supernatant was reacted with sodium molybdate reagent as per the standard protocol. (c) The image where the color of the NfB medium has changed to blue from green indicating an increase in the pH by the process of nitrogen fixation (d) The image which detects ammonia produced in growth medium (peptone broth) by Nesslerization reaction
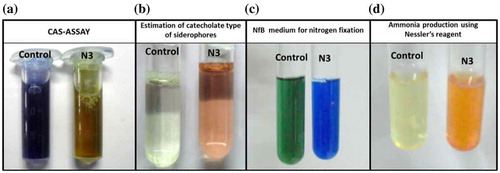
3.6. Non-symbiotic nitrogen fixation and ammonia production
Ammonia production by strain N3 was determined in peptone water broth by Nesslerization reaction (Figure (d)). Maximum ammonia produced by the strain was 3.6 μmol ml−1 after 96 h of growth (Figure ). There are two evidences that prove strain N3 can fix nitrogen. First, the strain can easily grow in absence of any nitrogen source, indicating the nitrogen required for its growth must be available from the environment. Second, NfB medium which is nitrogen-free medium containing limiting amount of carbon source shows an increase in the pH when strain N3 is grown in it. The pH increase in the medium is visualized by the color change of the medium from green to blue, as the NfB contains pH sensitive dye bromothymol blue (Figure (c)). The increase in the pH caused by the metabolic activity of strain N3 in NfB medium is due to nitrogen fixation. Thus, these experiments are suggestive that the strain can fix atmospheric nitrogen.
3.7. Effect of bio-fertilizer on seedling growth of green gram
P. mucilaginosus strain N3 showed multiple traits of PGPR including production that included IAA, ammonia, siderophore, nitrogen fixation, and solubilization of phosphate; its efficacy as a PGPR was tested in vivo on the growth of green gram. Chemical properties of soil used for pot study are shown in Table . Green gram seeds were treated with biofertilizer prepared from P. mucilaginosus strain N3 and seeded in pots. After 15 days, the germinated seedlings were uprooted and their vegetative parameters including stem and root mass (Fresh mass and Dry mass), total length, root length, and stem length were measured. It was observed that biofertilizer-treated seedlings showed 29.0% increase in total length and 26.9% increase in the total fresh mass as compared to non-treated control (Figure ). ANOVA analysis showed significant effect of biofertilizer treatment on vegetative parameters of the plant.
Table 2. Chemical and physical properties of soil used to study growth of green gram
Figure 7. The growth analysis of green gram when treated with N3-based talc biofertilizer with non-treated control
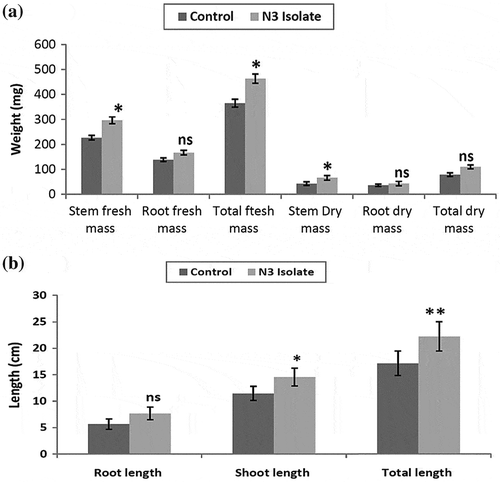
4. Discussion
Large soil microbial diversity is yet to be explored (Glick, Citation2014). Rhizosphere possess 100 folds higher bacterial density than in bulk soil as 5–21% of plant photosynthetic product is secreted by roots in form of different sugars which is in turn utilized by microbial populations (Govindasamy et al., Citation2011; Jha, Patel, Rajendran, & Saraf, Citation2010). Thus, the rhizospheric soil was selected for present study to isolate efficient strain (P. mucilaginosus strain N3) that can be categorized as efficient PGPR.
Over the period of last 10 years, new Paenibacillus strains are identified as nitrogen fixers (Xie et al., Citation2014). Lu, Xue, Cao, Yang, and Hu (Citation2014) reported various strains of P. mucilaginosus could fix nitrogen and suggested its potential application in agriculture. Under present study, nitrogen fixing ability detected by P. mucilaginosus strain N3 supports the findings of Lu et al. (Citation2014). Despite nitrogen fixing ability possessed by Paenibacillus strains they are poorly characterized for other traits aiding plant growth promotion. This lack of research has opened a new horizon to portray on the Paenibacillus strains as PGPR for development of sustainable agriculture. To overcome this constrain, present study is focused to demonstrate P. mucilaginosus strain N3 as nitrogen fixing bacteria along with possessing other plant-growth-promoting traits like IAA production, phosphate solubilization, siderophore production, ammonia, and catalase production.
Nitrogen and phosphorous are the most essential nutrient required for plant growth where, phosphorous is the second-most limiting nutrient for plants. Plants can absorb only mono and dibasic phosphate which are the soluble forms of phosphate (Jha, Patel, & Saraf, Citation2012). Phosphate-solubilizing microbes mineralize organic phosphorous in soil by solubilizing complex insoluble phosphates (Tri-calcium phosphate, Rock phosphate, Aluminum phosphate, etc.) and aid the phosphate availability to plants. Under present study, P. mucilaginosus strain N3 could efficiently solubilize tri-calcium phosphate in Pikovaskya’s medium. It was also observed that strain could produce organic acids which increases acidity of the medium. Here, phosphate solubilized by the strain is ascribed by acid produced; as under acidic environment complex form of phosphates brakes in to simple form, which is considered primary mechanism for phosphate solubilization by microbes (Goswami, Pithwa, et al., Citation2014). Stains of Paenibacillus that are known to solubilize phosphate include P. telluris PS38, P. polymyxa B1-4, P. brasilensis PB177, and P. kribensis CX-7 (Ai-min, Gang-yong, Shuang-feng, Rui-ying, & Bao-cheng, Citation2013; Arthurson, Hjort, Muleta, Jäderlund, & Granhall, Citation2011; Lee, Kim & Yoon, Citation2011).
PGPRs are known to produce several phytohormones such as gibberellins, cytokinins, auxins, etc. IAA-an auxin is an efficient molecule known to enhance plant growth by stimulating apical dominance and root growth (Bal, Das, Dangar, & Adhya, Citation2013). Majority of PGPR produce IAA form L-tryptophan as a primary precursor, whereas some does not require L-tryptophan (Idris, Iglesias, Talon, & Borriss, Citation2007). P. mucilaginosus strain N3 showed IAA production only when L-tryptophan was supplemented in the growth medium indicating the pathway used to produce IAA was L-tryptophan dependent. The most widely studied strain for IAA production among the genus Paenibacillus is P. polymyxa. Similar study was also performed by Lebuhn, Heulin, and Hartmann (Citation1997) where they showed IAA production by P. polymyxa was directly dependent on supplementation of L-tryptophan. They also claimed that indole-3-ethanol, indole-3-lactic acid, and indole-3-carboxylic acid were also produced by P. polymyxa in addition to IAA on L-tryptophan supplementation.
P. mucilaginosus strain N3 also showed production of siderophores. Siderophores are produced in the rhizosphere by PGPRs to quench iron. Iron-deficient condition in the rhizosphere caused by siderophore producing PGPR inhibits the growth of unwanted organisms. Thus, siderophores-producing microbes antagonize the growth of plant pathogens in the rhizosphere eventually helps plant to remain healthy (Haas & Défago, Citation2005; Jan, Azam, Ali, & Haq, Citation2011).
To portray any rhizobacterial strain as PGPR, it should be recognized to IAA production, phosphate solubilization, ammonia production, nitrogen fixation, and siderophore production, further, it is inevitable for strain to show plant growth promotion in vivo under pot trials (George, Gupta, Gopal, Thomas, & Thomas, Citation2013; Goswami et al., Citation2013). Our previous reports suggest that Pseudomonas spp. strain OG which showed several traits of PGPR also enhanced growth of green gram (V. radiata). Similarly for Bacillus licheniformis strain A2 and Kocuria turfanensis 2M4 isolated from saline soil, showed PGPR traits determined by in vitro biochemical tests could enhance growth of groundnut (Arachis hypogaea L.; Goswami, Pithwa, et al., Citation2014). Similarly, Mehta, Walia, Kulshrestha, Chauhan, and Shirkot (Citation2014) described phosphate solubilizing, Bacillus circulans CB7 can enhance the growth of tomato (Solanum lycopersicum L.) and described the strain as an efficient PGPR. Urease-producing Pseudomonas aeruginosa strain BG isolated from marine water was reported to show several traits of PGPR-enhanced growth of chick pea (Cicer arietinum L.; Goswami, Patel, et al., Citation2014). Under present study, we can categorize P. mucilaginosus strain N3 as an efficient PGPR as it possesses important PGPR traits and also shows growth promotion in green gram can be considered as an efficient PGPR.
Acknowledgement
Authors are thankful to Charotar University of Science and Technology (CHARUSAT) management for providing necessary facilities.
Additional information
Funding
Notes on contributors
Dweipayan Goswami
Dweipayan Goswami is a PhD research scholar at PDPIAS, CHARUSAT. He completed his MSc (Biotechnology) in 2011 from Gujarat University and BSc (Biochemistry with vocational Biotechnology) in 2009 from St. Xavier’s College, Ahmedabad. His research interests’ lies in understanding the role of rhizobacteria in growth promotion of plants and developing bacterial biocontrol agents to combat fungal infections caused in plants.
Janki N. Thakker
Janki N. Thakker is an assistant professor, Department of Biotechnology, P.D. Patel Institute of Applied Sciences (PDPIAS), Charotar University of Science and Technology (CHARUSAT) since 2007. She holds PhD (Biotechnology), MSc (Botany), and BSc (Microbiology) from Sardar Patel University, Vallabh Vidyanagar, Gujarat. Her research interest lies in isolation and purification of bioactive components from marine microbes, developing biocontrol agents for phytopathogens and study of plant–microbe interaction, identifying novel microbial strains capable of producing pigments, and studying the role of microbes and plants in biosynthesis of Nanoparticles.
References
- Ahmad, F. , Ahmad, I. , & Khan, M. S. (2008). Screening of free-living rhizospheric bacteria for their multiple plant growth promoting activities. Microbiological Research , 163 , 173–181. doi:10.1016/j.micres.2006.04.001
- Ai-min, Z. , Gang-yong, Z. , Shuang-feng, Z. , Rui-ying, Z. , & Bao-cheng, Z. (2013). Effect of phosphorus and potassium content of plant and soil inoculated with Paneibacillus kribensis CX-7 strain antioxidant and antitumor activity of Phyllanthus emblica in colon cancer cell lines. International Journal of Current Microbiology and Applied Science , 2 , 273–279. Retrieved from http://ijcmas.com/vol-2-6/Zhang%20Ai-min,%20et%20al.pdf
- Arthurson, V. , Hjort, K. , Muleta, D. , Jaderlund, L. , & Granhall, U. (2011). Effects on Glomus mosseae root colonization by Paenibacillus polymyxa and Paenibacillus brasilensis strains as related to soil P-availability in winter wheat. Applied and Environmental Soil Science , 2011 . Article ID: 298097. doi:10.1155/2011/298097
- Ash, C. , Priest, F. G. , & Collins, M. D. (1993). Molecular identification of rRNA group 3 bacilli (Ash, Farrow, Wallbanks and Collins) using a PCR probe test. Antonie van Leeuwenhoek , 64 , 253–260. doi:10.1007/BF00873085
- Bal, H. B. , Das, S. , Dangar, T. K. , & Adhya, T. K. (2013). ACC deaminase and IAA producing growth promoting bacteria from the rhizosphere soil of tropical rice plants. Journal of Basic Microbiology , 53 , 972–984. doi:10.1002/jobm.201200445
- Banerjee, M. R. , & Yasmin, L. (2002). Sulfur oxidizing rhizobacteria: An innovative environment friendly soil biotechnological tool for better canola production. In Proceeding of AGROENVIRON (pp. 1–7). Cairo, Egypt.
- Beneduzi, A. , Costa, P. B. , Parma, M. , Melo, I. S. , Bodanese-Zanettini, M. H. , & Passaglia, L. M. (2010). Paenibacillus riograndensis sp. nov., a nitrogen-fixing species isolated from the rhizosphere of Triticum aestivum . International Journal of Systematic and Evolutionary Microbiology , 60 , 128–133. doi:10.1099/ijs.0.011973-0
- Bric, J. M. , Bostock, R. M. , & Silverstone, S. E. (1991). Rapid in situ assay for indoleacetic acid production by bacteria immobilized on a nitrocellulose membrane. Applied and Environmental Microbiology , 57 , 535–538. Retrieved from http://www.ncbi.nlm.nih.gov/pmc/articles/PMC182744/
- Cappucino, J. C. , & Sherman, N. (Eds.). (1992). Microbiology: A laboratory manual (3rd ed.). New York, NY: Benjamin/Cumming.
- Carson, K. C. , Holliday, S. , Glenn, A. R. , & Dilworth, M. J. (1992). Siderophore and organic acid production in root nodule bacteria. Archives of Microbiology , 157 , 264–271. doi:10.1007/BF00245160
- Cattelan, A. J. , Hartel, P. G. , & Fuhrmann, J. J. (1999). Screening for plant growth-promoting rhizobacteria to promote early soybean growth. Soil Science Society of America Journal , 63 , 1670–1680. doi:10.2136/sssaj1999.6361670x
- de Freitas, J. R. , Banerjee, M. R. , & Germida, J. J. (1997). Phosphate-solubilizing rhizobacteria enhance the growth and yield but not phosphorus uptake of canola (Brassica napus L.). Biology and Fertility of Soils , 24 , 358–364. doi:10.1007/s003740050258
- Dey, R. , Pal, K. K. , Bhatt, D. M. , & Chauhan, S. M. (2004). Growth promotion and yield enhancement of peanut (Arachis hypogaea L.) by application of plant growth-promoting rhizobacteria. Microbiological Research , 159 , 371–394. doi:10.1016/j.micres.2004.08.004
- Dhandhukia, P. , Goswami, D. , Thakor, P. , & Thakker, J. N. (2013). Soil property apotheosis to corral the finest compressive strength of unbaked adobe bricks. Construction and Building Materials , 48 , 948–953. doi:10.1016/j.conbuildmat.2013.07.043
- Eastman, A. W. , Weselowski, B. , Nathoo, N. , & Yuan, Z. C. (2014). Complete genome sequence of Paenibacillus polymyxa CR1, a plant growth-promoting bacterium isolated from the corn rhizosphere exhibiting potential for biocontrol, biomass degradation, and biofuel production. Genome Announcements , 2 (1), e01218–13. doi:10.1128/genomeA.01218-13
- George, P. , Gupta, A. , Gopal, M. , Thomas, L. , & Thomas, G. V. (2013). Multifarious beneficial traits and plant growth promoting potential of Serratia marcescens KiSII and Enterobacter sp. RNF 267 isolated from the rhizosphere of coconut palms (Cocos nucifera L.). World Journal of Microbiology and Biotechnology , 29 , 109–117. doi:10.1007/s11274-012-1163-6
- Glick, B. R. (2014). Bacteria with ACC deaminase can promote plant growth and help to feed the world. Microbiological Research , 169 , 30–39. doi:10.1016/j.micres.2013.09.009
- Goswami, D. , Dhandhukia, P. , Patel, P. , & Thakker, J. N. (2014). Screening of PGPR from saline desert of Kutch: Growth promotion in Arachis hypogea by Bacillus licheniformis A2. Microbiological Research , 169 , 66–75. doi:10.1016/j.micres.2013.07.004
- Goswami, D. , Patel, K. , Parmar, S. , Vaghela, H. , Muley, N. , Dhandhukia, P. , & Thakker, J. N. (2014). Elucidating multifaceted urease producing marine Pseudomonas aeruginosa BG as a cogent PGPR and bio-control agent. Plant Growth Regulation . doi:10.1007/s10725-014-9949-1
- Goswami, D. , Pithwa, S. , Dhandhukia, P. , & Thakker, J. N. (2014). Delineating Kocuria turfanensis 2M4 as a credible PGPR: A novel IAA-producing bacteria isolated from saline desert. Journal of Plant Interactions , 9 , 566–576. doi:10.1080/17429145.2013.871650
- Goswami, D. , Vaghela, H. , Parmar, S. , Dhandhukia, P. , & Thakker, J. N. (2013). Plant growth promoting potentials of Pseudomonas spp. strain OG isolated from marine water. Journal of Plant Interactions , 8 , 281–290. doi:10.1080/17429145.2013.768360
- Govindasamy, V. , Senthilkumar, M. , Magheshwaran, V. , Kumar, U. , Bose, P. , Sharma, V. , & Annapurna, K. (2011). Bacillus and Paenibacillus spp.: Potential PGPR for sustainable agriculture. In D. K. Maheshwari (Ed.), Plant growth and health promoting bacteria (pp. 333–364). Dordrecht: Springer-Verlag Berlin Heidelberg.
- Haas, D. , & Défago, G. (2005). Biological control of soil-borne pathogens by fluorescent pseudomonads. Nature Reviews Microbiology , 3 , 307–319. doi:10.1038/nrmicro1129
- Ibekwe, A. M. , Poss, J. A. , Grattan, S. R. , Grieve, C. M. , & Suarez, D. (2010). Bacterial diversity in cucumber (Cucumis sativus) rhizosphere in response to salinity, soil pH, and boron. Soil Biology and Biochemistry , 42 , 567–575. doi:10.1016/j.soilbio.2009.11.033
- Idris, E. E. , Iglesias, D. J. , Talon, M. , & Borriss, R. (2007). Tryptophan-dependent production of indole-3-acetic acid (IAA) affects level of plant growth promotion by Bacillus amyloliquefaciens FZB42. Molecular Plant-Microbe Interactions , 20 , 619–626. doi:10.1094/MPMI-20-6-0619
- Jacobson, C. B. , Pasternak, J. J. , & Glick, B. R. (1994). Partial purification and characterization of 1-aminocyclopropane-1-carboxylate deaminase from the plant growth promoting rhizobacterium Pseudomonas putida GR12-2. Canadian Journal of Microbiology , 40 , 1019–1025. doi:10.1139/m94-162
- Jan, A. T. , Azam, M. , Ali, A. , & Haq, Q. M. R. (2011). Novel approaches of beneficial Pseudomonas in mitigation of plant diseases—An appraisal. Journal of Plant Interactions , 6 , 195–205. doi:10.1080/17429145.2010.541944
- Jha, C. K. , Patel, B. , & Saraf, M. (2012). Stimulation of the growth of Jatropha curcas by the plant growth promoting bacterium Enterobacter cancerogenus MSA2. World Journal of Microbiology and Biotechnology , 28 , 891–899. doi:10.1007/s11274-011-0886-0
- Jha, C. K. , Patel, D. , Rajendran, N. , & Saraf, M. (2010). Combinatorial assessment on dominance and informative diversity of PGPR from rhizosphere of Jatropha curcas L. Journal of Basic Microbiology , 50 , 211–217. doi:10.1002/jobm.200900272
- Kennedy, I. R. , Pereg-Gerk, L. L. , Wood, C. , Deaker, R. , Gilchrist, K. , & Katupitiya, S. (1997). Biological nitrogen fixation in non-leguminous field crops: Facilitating the evolution of an effective association between Azosirillum and wheat. Plant and Soil , 194 , 65–79. doi:10.1023/A:1004260222528
- King, E. J. (1932). The colorimetric determination of phosphorus. Biochemical Journal , 26 , 292–297. Retrieved from http://www.ncbi.nlm.nih.gov/pmc/articles/PMC1260904/
- Kloepper, J. W. , & Schroth, M. N. (1978, August). Plant growth-promoting rhizobacteria on radishes. In Proceedings of the 4th International Conference on plant pathogenic bacteria ( Vol. 2, pp. 879–882). Gilbert-Clarey: Tours
- Lebuhn, M. , Heulin, T. , & Hartmann, A. (1997). Production of auxin and other indolic and phenolic compounds by Paenibacillus polymyxa strains isolated from different proximity to plant roots. FEMS Microbiology Ecology , 22 , 325–334. doi:10.1111/j.1574-6941.1997.tb00384.x
- Lee, J. C. , Kim, C. J. , & Yoon, K. H. (2011). Paenibacillus telluris sp. nov., a novel phosphate-solubilizing bacterium isolated from soil. The Journal of Microbiology , 49 , 617–621. doi:10.1007/s12275-011-0471-0
- Lu, J. J. , Xue, A. Q. , Cao, Z. Y. , Yang, S. J. , & Hu, X. F. (2014). Diversity of plant growth-promoting Paenibacillus mucilaginosus isolated from vegetable fields in Zhejiang, China. Annals of Microbiology . doi:10.1007/s13213-014-0818-y
- Mehta, P. , Walia, A. , Kulshrestha, S. , Chauhan, A. , & Shirkot, C. K. (2014). Efficiency of plant growth-promoting P-solubilizing Bacillus circulans CB7 for enhancement of tomato growth under net house conditions. Journal of Basic Microbiology . doi:10.1002/jobm.201300562
- Murray, R. G. E. , Doetsch, R. N. , & Robinow, F. (1994). Determinative and cytological light microscopy. In P. Gerhardt , R. G. E. Murray , W. A. Wood , & N. R. Krieg (Eds.), Methods for general and molecular bacteriology (pp. 21–42). Washington, DC: American Society for Microbiology.
- Naing, K. W. , Anees, M. , Kim, S. J. , Nam, Y. , Kim, Y. C. , & Kim, K. Y. (2014). Characterization of antifungal activity of Paenibacillus ehimensis KWN38 against soilborne phytopathogenic fungi belonging to various taxonomic groups. Annals of Microbiology , 64 , 55–63. doi:10.1007/s13213-013-0632-y
- Patel, D. , Jha, C. K. , Tank, N. , & Saraf, M. (2011). Growth enhancement of chickpea in saline soils using plant growth-promoting rhizobacteria. Journal of Plant Growth Regulation , 31 , 53–62. doi:10.1007/s00344-011-9219-7
- Patten, C. L. , & Glick, B. R. (1996). Bacterial biosynthesis of indole-3-acetic acid. Canadian Journal of Microbiology , 42 , 207–220. doi:10.1139/m96-032
- Payne, S. M. (1994). Detection, isolation, and characterization of siderophores. Methods in Enzymology , 235 , 329–344. doi:10.1016/0076-6879(94)35151-1
- Richardson, A. E. (2001). Prospects for using soil microorganisms to improve the acquisition of phosphorus by plants. Functional Plant Biology , 28 , 897–906. doi:10.1071/PP01093
- Shi, W. , Takano, T. , & Liu, S. (2012). Anditalea andensis gen. nov., sp. nov., an alkaliphilic, halotolerant bacterium isolated from extreme alkali–saline soil. Antonie van Leeuwenhoek , 102 , 703–710. doi:10.1007/s10482-012-9770-7
- Sunar, K. , Dey, P. , Chakraborty, U. , & Chakraborty, B. (2013). Biocontrol efficacy and plant growth promoting activity of Bacillus altitudinis isolated from Darjeeling hills, India. Journal of Basic Microbiology , 53 , 1–14. doi:10.1002/jobm.201300227
- Tamura, K. , Dudley, J. , Nei, M. , & Kumar, S. (2007). MEGA4: Molecular evolutionary genetics analysis (MEGA) software version 4.0. Molecular Biology and Evolution , 24 , 1596–1599. doi:10.1093/molbev/msm092
- Vessey, J. K. (2003). Plant growth promoting rhizobacteria as biofertilizers. Plant and Soil , 255 , 571–586. doi:10.1023/A:1026037216893
- Xie, J. B. , Du, Z. , Bai, L. , Tian, C. , Zhang, Y. , Xie, J. Y. , & Wang, T. (2014). Comparative genomic analysis of N2-fixing and non-N2-fixing Paenibacillus spp.: Organization, evolution and expression of the nitrogen fixation genes. PLoS Genetics , 10 , e1004231. doi:10.1371/journal.pgen.1004231