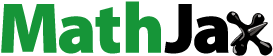
Abstract
In order to design equipment for improved processing of melon (Citrullus colocynthis lanatus) seed and kernel, some of the engineering properties need to be determined as a function of moisture content. The geometric (axial dimensions, mean diameters), gravimetric (bulk and true densities, 1,000 seed and kernel weight, and porosity) and frictional (angle of repose and static coefficient of friction) properties of melon seed and kernel in the moisture content range of 2.8–25% (d.b.) and 1.1–23% (d.b.), respectively, were investigated. The objectives of the study were to determine the effect of moisture on some moisture-dependent physical properties of melon seed and kernel, and to develop regression models for each of the properties investigated. The effect of moisture content was significant (p < 0.05) on all the physical properties investigated. The regression models developed all had high coefficient of determination, R 2 except for the sphericity and porosity of the melon seed with an R 2 of 0.3943 and 0.6570, respectively, and also, the porosity of the melon kernel with an R 2 of 0.5110. At all moisture content levels, plywood had the highest friction coefficient for the melon seed and kernel.
Public Interest Statement
Melon (Citrullus colocynthis lanatus) popularly referred to as “egusi” is one of the important oil seed crops widely grown and consumed in tropical Africa. Melon is mainly grown for its kernel, which could be eaten individually as snack when roasted and used extensively for cooking purposes, either as a soup additive or as cooking oil source. The physical and mechanical properties of seeds depend on their moisture content, and are important in the design of planting, harvesting, handling, transportation, storing, drying and processing equipment. It is important to note that studies on properties of melon seed as a function of moisture content are scarce which necessitated the need for this study. This study is aimed at providing relevant data on the moisture-dependent physical properties of melon seed (Citrullus colocynthis lanatus), and its kernel essential for the design of processing, handling and storage equipment. This study investigated some moisture-dependent physical properties of melon (Citrullus colocynthis lanatus) seed and its kernel in the moisture content range of 2.8–25% (d.b.) and 1.1–23% (d.b.), respectively. The result of the study showed that the moisture content of the seed and kernel significantly affects the properties of the melon. Models were developed to establish the relationship between the physical properties of the melon seed and kernel.
1. Introduction
Melon (Citrullus colocynthis lanatus) popularly referred to as “egusi” is one of the important oil seed crops widely grown and consumed in tropical Africa (Shittu & Ndirika, Citation2012). It belongs to the family of Cucurbitaceae, with excellent genetic diversity, vegetative and reproductive characteristics. It contains between 30 and 50% by weight of oil and offers valuable sources of vegetable oil for local and export trade (Oloko & Agbetoye, Citation2006). Melon is mainly grown for its kernel, which could be eaten individually as snack when roasted and used extensively for cooking purposes, either as a soup additive or as cooking oil source. It is a good source of amino acids, such as arginine, vitamins B1, vitamins B2, niacin, tryptophan and methionone, and minerals, such as zinc, iron, potassium, phosphorus, sulphur, manganese, calcium, lead, chloride and magnesium (Akobundu, Cherry, & Simmons, Citation1982; Eugene & Gloria, Citation2002). As was reported by Norton (Citation1993), the average protein and oil content of melon seeds are, respectively, 26.2 and 47.3%, and these values are relatively high compared with corresponding values of some common oil-bearing seeds as cotton with 20.2 and 21.2% and groundnut with 23.2 and 44.8%, respectively.
Various physical and mechanical properties of seeds are dependent on moisture content, and appear to be important in the design of planting, harvesting, handling operation, transportation, storing, drying and processing equipments (Bande, Adam, Azmi, & Jamarei, Citation2012; Srivastava, Mahoney, & West, Citation1990). Despite an extensive literature search, information on the physical and mechanical properties of melon seed and kernel and their dependence on moisture content are scare in the literature. Most biomaterials are hygroscopic and can easily interact with the moisture in the air risking mold growth and affecting their behaviour during processing. The major moisture-dependent physical properties of biological materials are shape, linear dimensions, mean diameters, surface area, sphericity, mass, true density, bulk density, porosity, angle of repose and static friction coefficient against various surfaces (Mohsenin, Citation1980). Size, shape and physical dimensions of seeds and kernels are important in sizing, sorting, sieving and other separation processes (Galedar, Jafari, & Tabatabaeefar, Citation2008). Bulk density determines the capacity of storage and transport systems, while true density is useful for separation equipment; porosity of the mass of seeds determines the resistance to airflow during aeration and drying of nuts and kernels (Galedar et al., Citation2008; Vilche, Gely, & Santalla, Citation2003). Angle of repose is a useful parameter for calculation of conveyor width and for designing the shape of storage, while frictional forces affect the amount of power required to convey the material (Galedar et al., Citation2008). Many researchers have determined the moisture-dependent properties of other seeds and grains, viz. pigeon pea (Shepherd & Bhardwaj, Citation1986), soybean (Deshpande, Bal, & Ojha, Citation1993), sorghum seed (Mwithiga & Masika, Citation2006, caper (Sessiz, Esgici, & Kızıl, Citation2007), pistachio nut (Razavi, Rafe, Mohammad, & Mohammmadi, Citation2007), pomegranate seeds (Kingsly, Singh, Manikantan, & Jain, Citation2006), jatropha seed (Garnayak, Pradhan, Naik & Bhatnagar, Citation2008) and Karanja kernel (Pradhan, Naik, Bhatnagar, & Swain, Citation2008).
Reports on physical characteristics of various seeds of the Cucurbitaceae family are available. Davies (Citation2010) investigated the engineering properties of three varieties of melon seed at the moisture content of 6.25, 6.33 and 5.21% dry basis, respectively. It was reported that the length, width, thickness, arithmetic and geometric diameter, sphericity, surface area and 1000 unit mass ranged from 12.81 to 14.50 mm, 7.02 to 8.42 mm, 2.22 to 2.49 mm, 7.36 to 8.31 mm, 5.84 to 6.54 mm, 0.47 to 0.53, 134.64 to 192.23 mm² and 94.0 to 110.0 g respectively. Milani et al. (Citation2007) investigated some physical properties of three common Iranian varieties of cucurbit seeds (Riz, Chiny and Gushty), as a function of moisture content in the range of 5.18–42.76% (w.b.). The results showed that the mean values of all geometric properties increased with increasing moisture content. Razavi and Milani (Citation2006) investigated several physical properties of three dried watermelon seed varieties. Their results showed that the Red variety had the maximum volume, length, width, arithmetic mean diameter, geometrical mean diameter, sphericity, surface area and funnelling angle of repose among the varieties, while the greatest thickness was obtained for Sarakhsi variety and the highest accounted bulk density and true density belonged to Kolaleh variety. Makanjuola (Citation1972) determined the size and shape of the seeds of two melon varieties and correlated the dimensions of the seeds and kernels. He measured the bending properties of melon seed as a function of moisture content and orientation of loading.
Aviara, Gwandzang, and Haque (Citation1999) noted that the moisture-dependent characteristics of the physical properties of agricultural products have an effect on the adjustment and performance of processing machines. Understanding the effect of moisture on the physical and mechanical properties of melon seed is essential in the processing of melon seed, particularly on the shelling efficiency and percentage seed damage of the seed (Okokon, Ekpenyong, Nwaukwa, Akpan, & Abam, Citation2010; Shittu & Ndirika, Citation2012). Furthermore, Galedar et al. (Citation2008) noted that in order to design equipment for improved processing of seeds, some of the engineering properties need to be determined as a function of moisture content. It is important to note that studies on properties of melon seed as a function of moisture content is scarce. This study is aimed at providing relevant data on the moisture-dependent physical properties of melon seed (C. colocynthis lanatus), and its kernel essential for the design of processing, handling and storage equipment. The specific objectives were (1) to determine some physical properties of melon seed and its kernel at different moisture content ranging from 2.8 to 25% (d.b.) and 1.1 to 23% (d.b.), respectively; (2) to determine the effect of moisture content on the properties of the seed and the kernel; and (3) to develop predictive models for each of the properties investigated as a function of moisture content.
The following moisture-dependent physical properties were investigated in this study: linear dimensions, arithmetic mean diameter, geometric mean diameter, sphericity, surface area, volume, 1,000 seed weight, bulk and true densities, porosity, angle of repose and static coefficient of friction on four structural surfaces. All the physical properties of melon seed and its kernel were determined at five moisture levels with three replications. Mean and standard deviations were calculated using Microsoft Excel software (2003). The effect of moisture content on the properties of the seed and its kernel were determined using the analysis of variance (ANOVA) method at 5% significance level using GenStat Analytical Software. The best relationship between the moisture content and the physical properties were determined using linear and non-linear regression analysis of GenStat Analytical Software. Coefficient of determination (R 2) and residual plotting were used to evaluate the fitting of a model to experimental data. The best model was chosen as the one with the highest coefficient of determination and randomly distributed residual about the zero axis.
2. Materials and methods
2.1. Sample preparation and moisture content determination
Melon seed and kernel were obtained from a local market in Enugu state, south-eastern Nigeria. The seed sample was cleaned manually to remove all foreign materials, such as broken seeds, stones and leaves. The initial moisture content of seed and the kernel were determined using the oven dry method at 103 ± 2°C until a constant weight was reached (Kashaninejad, Mortazavi, Safekordi, & Tabil, Citation2005). The moisture content was calculated on dry basis as shown in Equation 1:(1)
(1)
where, MCDB is the moisture content in dry basis of the material, M w is the initial weight of the material, M D is the weight of the material after drying.
In view of the significance of moisture content in the properties of biomaterial, the physical properties of the melon were assessed at five moisture content levels of 2.8, 7, 12, 17 and 25% (d.b.) for the seed and 1.1, 6, 11, 16 and 23% (d.b.) for the kernel. In order to attain the desired moisture contents, the seed and kernel samples having initial moisture content of 2.8 and 1.1% (d.b.), respectively, were conditioned by adding a calculated amount of distilled water, Q, sealing them in separate polyethylene bags and storing in a refrigerator at 3°C for about one week for proper moisture distribution. This rewetting technique was used to attain the desired moisture content needed for the study (Bulent Coşkun, Yalçin, & Özarslan, Citation2006; Enoch et al., Citation2008). The quantity of distilled water was calculated from the following equation:(2)
(2)
where, W 1 is the weight of the sample, M 1 is the initial moisture content of the sample, M 2 is the desired moisture content of the sample.
Before each experiment, the required sample was taken out and kept in ambient environment for 24 h to equilibrate with the ambient temperature.
2.2. Geometric properties
2.2.1. Seed and kernel size
The physical dimensions of the seed and kernel were determined by randomly picking 100 seeds and kernels from the bulk of the material and measuring the seed length, width and thickness (Figure ) at different moisture contents using a SKOLE digital vernier calliper measuring to an accuracy of 0.001 mm. The geometric mean diameter, D
g
, and the arithmetic mean diameter, D
a
, of the seed and kernel were calculated using the relationship given by Mohsenin (Citation1986):(3)
(3)
(4)
(4)
where, L, W and T are the length, width and thickness.
2.2.2. Surface area, sphericity, aspect ratio and volume
The surface area, S
a
(mm2), was determined from the geometric mean diameter as shown in Equation 5 (Garnayak et al., Citation2008; Sharma et al., Citation2011).(5)
(5)
The degree of sphericity, S
p
, as expressed by Mohsenin (Citation1986), was used to calculate the sphericity of melon as follows:(6)
(6)
The aspect ratio (R
a
) was calculated as follows (Tabatabaeefar, Citation2003):(7)
(7)
The principal dimensions were used to calculate the volume (V) of the melon seed and kernel expressed as (Eissa & Gamea, Citation2003):(8)
(8)
where B = (WL)1/2.
2.3. Gravimetric properties
2.3.1. A 1,000 seed and kernel weight
To obtain the 1,000 seed and kernel weight, the seed and kernel were weighed using a precision electronic balance (SHIMADZU BZ 32 OH, Japan) reading to an accuracy of 0.001 g. The one thousand (1,000) seed weight, by sample size of 250 seeds and kernels randomly selected, were weighed and multiplied by four (Sharma et al., Citation2011; Vilche, Gely, & Santalla, Citation2003).
2.3.2. Bulk and true densities, and porosity
The bulk density was determined by filling a cylindrical container of known volume with the material and then weighing the cylinder. Dropping the seeds from a known height produces a tapping effect in the container to reproduce the settling effect during storage. The bulk density, ρ
b
was calculated as the ratio of weight of the material to the volume of the cylinder (Sharma et al., Citation2011).(9)
(9)
where, V c is the volume of cylinder (m3), W s is the weight of seed/kernel (kg).
To calculate the true density, ρ
t
, the unit mass of each sample was determined by weighing using an electronic balance reading to an accuracy of 0.001 g. The true volume, V (m3), as a function of moisture content was determined using the liquid displacement method (Pliestic, Dobricevic, Filipovic, & Gospodaric, Citation2006). True density, ρ
t
(kg m−3) of the samples was calculated by dividing the unit mass of each sample by its true volume. The porosity, P, was determined in terms of bulk density (ρ
b
) and true density (ρ
t
) using the following equation (Mohsenin, Citation1986):(10)
(10)
2.4. Frictional properties
2.4.1. Angles of repose
The filling angle of repose is the angle with the horizontal at which the material will stand when piled. This was determined using a topless and bottomless cylinder of 15 cm diameter and 25 cm height. The cylinder was placed at the centre of a raised circular plate having a diameter of 35 cm and filled with the grain. The cylinder was raised slowly until it formed a cone on the circular plate. The height of the cone was measured and the filling angle of repose, (θ
f
), calculated using the following equation (Razavi et al., Citation2007):(11)
(11)
where H is the height of the cone; and D is the diameter of the cone.
The funnelling angle of repose was determined using the method described by Milani et al. (Citation2007). A fibreglass box of 20 × 20 × 20 cm having a removable front panel was used. The box was filled with the material, and then the front panel quickly removed allowing them to flow and assume a natural slope (Joshi, Das, & Mukherjee, Citation1993). The funnelling angle of repose θ
e
was calculated from the measurement of the depth of the free surface of the sample at the centre, using the following equation (Paksoy & Aydin, Citation2004):(12)
(12)
where, H is the height of flow and X is the distance of flow.
2.4.2. Static coefficient of friction
The static coefficient of friction, μ, was determined for four different structural surfaces, namely plywood, galvanized sheet, rubber and foil material using the method described by Dutta, Nema, and Bhardwaj (Citation1988). A plastic cylinder of 100 mm diameter and 50 mm height was placed on an adjustable tilting plate having the test surface firmly placed on it. The plastic cylinder was filled with the experimental sample and raised slightly so as not to touch the surface (about 5 mm). The structural surface with the cylinder resting on it was inclined gradually, using a screw device, until the cylinder just started to slide down. The angle of tilt was read from a graduated scale and the tangent of this angle was recorded as the static coefficient of friction on that surface.(13)
(13)
3. Results and discussion
3.1. Geometric properties
The result of the geometric properties of the melon seed and the kernel which include the length, L (mm), width, W (mm), thickness, T (mm), geometric mean diameter, D g (mm), arithmetic mean diameter, D a (mm), surface area, S a (mm2), sphericity, S p (%), aspect ratio, R a (%), and the volume V, (mm3) are shown in Tables and , respectively. The moisture content level investigated for the melon seed ranged 2.8–25% (d.b.), while the moisture content level investigated for the kernel ranged 1.1–23% (d.b.). Generally, the mean value recorded for the geometric properties of the seed increased rapidly as the moisture content increased from 2.8 to 12% (d.b.), and afterward the increase became gradual. And for the kernel, the increases in the mean value of the geometric properties were rapid as the moisture content increased from 1.1 to 11% (d.b.), after which the increase became gradual.
Table 1a. Mean value and standard deviation of some geometric properties of melon seed at different moisture content levels
Table 1b. Mean value and standard deviation of some geometric properties of melon kernel at different moisture content levels
3.1.1. Length, width and thickness
For all the geometric properties investigated, it was observed that the mean values recorded for both the melon seed and the kernel increased with increasing moisture content. The length, width and thickness of the seed varied from 13.91 to 14.37 mm, 8.49 to 8.88 mm and 1.71 to 2.16 mm, respectively, as the moisture content increased from 2.8 to 25% (d.b.) (Table ). While the length, width and thickness of the melon kernel varied from 13.24 to 14.08 mm, 7.71 to 8.21 mm and 1.47 to 1.92 mm, respectively, as the moisture content increased from 1.1 to 23% (d.b.) (Table ). Similar trend was reported by Koocheki et al. (Citation2007) for different varieties of watermelon seed. ANOVA carried out on the mean values recorded for the axial dimensions of the melon seed and the kernel showed that the effect of moisture content on the axial dimensions was significant at 5% significant level (p < 0.05). Regression analysis carried out on the mean axial dimensions of the melon seed and kernel to establish the relationship between the axial dimensions and the moisture content showed a very strong correlation, R 2 (Table ). The mean values were fitted into polynomial regression equations as shown in Table . The coefficient of determination R 2 for the regression models developed for the seed ranged from 0.9478 to 0.9888, while that of the kernel ranged from 0.9756 to 0.9990. The high coefficients of determination suggest that upon absorption of water, the melon seed and kernel expand along the axial dimensions (Tavakoli, Tavakoli, Rajabipour, Ahmadi, & Gharib-Zahedi, Citation2009).
Table 2. Equations representing the relation between the axial dimensions (L, W and T) and moisture content for melon seed and its kernel
3.1.2. Mean diameters, surface area, sphericity, aspect ratio and volume
The result of the mean diameters (geometric and arithmetic mean diameters), surface area, sphericity, aspect ratio and volume of the melon seed and kernel in the moisture content range of 2.8–25% (d.b.) and 1.1–23% (d.b.), respectively, is shown in Tables and . It was observed that these properties increased with increasing moisture content level as was reported for different varieties of watermelon seed by Koocheki et al. (Citation2007). The geometric and the arithmetic mean diameters of the seed ranged from 5.81 to 6.44 mm and 8.04 to 8.41 mm, respectively, as the moisture content increased from 2.8 to 25% (d.b.) while that of the kernel ranged from 5.30 to 6.01 mm and 7.47 to 8.07 mm, respectively, as the moisture content increased from 1.1 to 23% (d.b.). The surface area, sphericity, aspect ratio and volume of the seed increased from 106.87 to 132.09 mm2, 0.42 to 0.45, 61.15 to 61.94% and 42.40 to45.57 mm3, respectively. For the melon kernel, the surface area, sphericity, aspect ratio and volume of the seed increased from 88.53 to 114.40 mm2, 0.40 to 0.43, 58.35 to 58.56% and 37.45 to 42.37 mm3, respectively. Koocheki et al. (Citation2007), Paksoy and Aydin (Citation2004), Sacilik, Öztürk, and Keskin (Citation2003) and Baryeh (Citation2002) reported similar behaviour for watermelon seed, edible squash seeds, hemp seed and millet seed, respectively. However, Hsu, Mannapperuma, and Singh (Citation1991) reported that the surface area of pistachios decreased with increasing grain moisture content. The minimal increase in the sphericity of the seed and kernel suggests that the differential dimensional changes of their three major dimensions were minimal as they gained moisture (Tavakoli et al., Citation2009).
The ANOVA carried out showed a significant effect (p < 0.05) of moisture content level on the mean diameters, surface area, sphericity, aspect ratio and volume of the melon seed and kernel. Regression analysis was used to developed regression models for the mean diameters, surface area, sphericity, aspect ratio and volume of the seed and kernel as a function of moisture content (Table ). The coefficient of determination, R 2, for the regression models developed ranged from 0.3930 to 0.9930 for the seed and 0.8430 to 0.9997 for the kernel. The regression model developed for the sphericity had a low coefficient of determination, R 2, 0.3930. This reflects a very weak correlation with the moisture content. The regression models could be used to predict the value of any of the properties, given the moisture content within the investigated range.
Table 3. Equations representing the relation between the mean diameters, surface area, sphericity, aspect ratio and volume of melon seed and kernel as a function of moisture level
3.2. Gravimetric properties
The mean value recorded for the gravimetric properties, which include the bulk and true densities, 1,000 seed weight and porosity of melon seed and kernel in the moisture range of 2.8–25% (d.b.) and 1.1–23% (d.b.), respectively, is shown in Tables and . The properties were observed to increase as the moisture content of the seed and kernel increased. Generally, for the melon seed and kernel, the increase in the mean value of the gravimetric properties was rapid as the moisture content increased from 2.8–12% (d.b.) and 1.1–11% (d.b.), respectively. However, as the moisture content increased further, the increase in the mean value of the gravimetric properties of the melon seed and kernel became less rapid. The bulk and true densities of the melon seed ranged from 408.04 to 500.00 kg/m3 and 820.00 to 1189.00 kg/m3 (Table ), respectively, as the moisture content increased from 2.8 to 25% (d.b.), while the bulk and true densities of the kernel ranged from 474.80 to 539.00 kg/m3 and 1039.40 to 1229.50 kg/m3 (Table ), respectively, as the moisture content increased from 1.1 to 23% (d.b.). The increase in the bulk and true density of the seed and kernel was due to the fact that an increase in mass owing to moisture gain in the sample was higher than the accompanying volumetric expansion (Pliestic et al., Citation2006; Tavakoli et al., Citation2009).
Table 4a. Mean value and standard deviation of some gravimetric properties of melon seed at different moisture content levels
Table 4b. Mean value and standard deviation of some gravimetric properties of melon kernel at different moisture content levels
The same trend observed for the bulk density have also been reported by Koocheki et al. (Citation2007), Paksoy and Aydin (Citation2004), Aydın and Özcan (Citation2002), Aviara et al. (Citation1999) for watermelon seed, squash seed, terebinth fruits and guna seeds, respectively. However, Al-Mahasneh and Rababah (Citation2007) and Dursun and Dursun (Citation2005) found a negative relationship between the bulk density and the moisture content for green wheat and for caper seeds, respectively. Koocheki et al. (Citation2007) noted that such discrepancies could be due to the cell structure, the volume and mass increase characteristics of the seeds as moisture content increases. The increase in true density of the melon seed and kernel as the moisture content increased was similar to those reported by Gupta and Das (Citation1997) for sunflower, Ogut (Citation1998) for white lupin and Chandrasekar and Viswanathan (Citation1999) for coffee. However, it was contrary to the results of Mwithiga and Masika (Citation2006), Al-Mahasneh and Rababah (Citation2007), Sessiz et al. (Citation2007) and Cetin (Citation2007), who found the true density to decrease with increase in the moisture content for sorghum seeds, green wheat, caper fruit and barbunia bean, respectively. These seeds have been reported to have lower weight increase in comparison to volume increase as their moisture content increase (Koocheki et al. Citation2007).
The 1,000 seed weight increased from 43.60 to 168.43 g as the moisture content of the seed increased from 2.8 to 25% (d.b.), while the 1,000 kernel weight of melon increased from 87.33 to 105.33 g as the moisture content increased from 1.1 to 23% (d.b.). The mean observed values recorded for the porosity of the melon seed were sinusoidal as the moisture content increased, whereas for the melon kernel, it increased from 54.33 to 56.61% as the moisture content increased from 1.1 to 23% (d.b.). The porosity is an important factor in packing and it affects the resistance to airflow through bulk seeds and kernels. Koocheki et al. (Citation2007) reported a decrease in the porosity of watermelon seed as the moisture content increased owing to the change in true and bulk density of the seed. The ANOVA carried out on the gravimetric properties of the melon seed and kernel revealed that the effect of moisture content level was significant (p < 0.05) on all the gravimetric properties investigated. Regression models were developed to establish the relationship between the gravimetric properties and the moisture content (Table ). The coefficient of determination, R 2, ranged from 0.6570 to 0.9570 for melon seed, while it ranged from 0.5110 to 0.9990 for the melon kernel. All the gravimetric properties investigated for the melon seed and kernel showed a strong correlation with the moisture content; however, the porosity showed a weak correlation with the moisture content for the seed and melon with R 2 of 0.6570 and 0.5110, respectively (Table ).
Table 5. Equations representing the relationship between the gravimetric properties and moisture content of melon seed and its kernel
3.3. Frictional properties
The mean values recorded for the frictional properties of melon seed and kernel at different moisture content levels are shown in Tables and , respectively. The frictional properties of the melon seed and kernel generally increased as the moisture content increased from 2.8 to 25% (d.b.) and 1.1 to 23% (d.b.), respectively. The filling and the funnelling angles of repose of the melon seed ranged from 20.67 to 40.66° and 30.73 to 57.35°, while the static coefficient of friction of the seed on plywood, rubber, galvanized sheet and foil surfaces ranged from 0.46 to 0.67, 0.36 to 0.44, 0.37 to 0.43 and 0.40 to 0.49, respectively. For the kernel, on the other hand, the filling and the funnelling angles of repose ranged from 30.52 to 38.04° and 45.62 to 67.19°, respectively. The static coefficient of friction of the melon kernel on the following structural surfaces – plywood, rubber, galvanized sheet and foil varied from 0.52 to 0.70, 0.45 to 0.69, 0.51 to 0.59 and 0.47 to 0.65, respectively. Similar trend has been reported for watermelon seed (Koocheki et al., Citation2007), millet (Baryeh, Citation2002), almond nut (Aydin, Citation2003), pistachio nut and kernel (Razavi et al., Citation2007), caper fruit (Sessiz et al., Citation2007) and barbunia bean (Cetin, Citation2007).
Table 6a. Mean value and standard deviation of some frictional properties of melon seed at different moisture content levels
Table 6b. Mean value and standard deviation of some frictional properties of melon kernel at different moisture content levels
The increasing trend of the angle of repose with increasing moisture content could be attributed to the surface layer of moisture surrounding the particle holding the aggregate of seed and kernel together by the surface tension reducing the ease with which they slide over each other (Tavakoli et al., Citation2009). This means that the forces of solid friction at the seed/material interface generally increased when the seed and kernel moisture content increased. The angle of repose is important in designing equipment for mass flow and structures for storage. The increase in the static coefficient of friction of the seed and kernel as the moisture content increased could be due to the water present, offering a cohesive force on the surface of contact, resulting in higher stickiness of the surface of the seeds/kernels that confines the ease of sliding (Koocheki et al., Citation2007; Tavakoli et al., Citation2009). In all of the frictional properties investigated, the lowest value was recorded at the 2.8 and 1.1% (d.b.) moisture levels for the seed and kernel, respectively, while the highest value was recorded at the 25 and 23% (d.b.) moisture levels for the seed and the kernel, respectively. At all moisture levels for the melon seed and kernel, plywood surface offered the highest static coefficient of friction. Garnayak et al. (Citation2008) and Koocheki et al. (Citation2007) also reported that plywood surface offered the highest static coefficient of friction for Jatropha and watermelon seeds, respectively. This might be due to the surface roughness which is largest in the case of plywood, reducing the sliding characteristics of the seed and kernel, such that the static coefficient of friction is increased. Static coefficient of friction is important in the design of conveyors as friction is necessary to hold the grains to the conveying surface without slipping or sliding backward (Tavakoli et al., Citation2009).
The ANOVA carried out on the data reported for the frictional properties of melon seed and kernel showed that mean values reported were significantly influenced (p < 0.05) by the moisture level. Regression analysis was carried out to develop predictive models for the frictional properties investigated as a function of moisture content (Table ).
Table 7. Equations representing the relationship between the frictional properties and moisture content of melon seed and its kernel
The models had high coefficient of determination, R 2, ranging from 0.8210 to 0.9982 for the melon seed and 0.8470 to 0.9990 for the melon kernel. Aviara et al. (Citation1999) and Bart-Plange and Baryeh (Citation2003) reported that the funnelling angle of repose increased non-linearly with increase in moisture content for guna seeds and cocoa beans, respectively. Also, Konak, Çarman, and Aydin (Citation2002), Kaleemullah and Gunasekar (Citation2002), Baryeh and Mangope (Citation2002) and Coşkuner and Karababa (Citation2007) found that the static coefficient of friction has non-linear relationship with moisture content for chick pea, Arecanut, hazelnut, QP-38 pigeon pea and coriander seeds, respectively.
4. Conclusions
This study investigated some moisture-dependent physical properties of melon (C. colocynthis lanatus) seed and its kernel in the moisture content range of 2.8–25% (d.b.) and 1.1–23% (d.b.), respectively. The following conclusions were arrived at from the results of the study:
The effect of moisture content on the geometric, gravimetric and frictional properties of melon seed and kernel were significant at 5% significant level (p < 0.05).
It was evidenced that the increase in moisture content of melon seed and kernel increased the mean values of the geometric, gravimetric and frictional properties investigated.
Increase in the mean values of the properties investigated was rapid as the moisture content of the seed and kernel increased from 2.8 to 12% (d.b.) and 1.1 to 11% (d.b.), respectively, after which the increase in the mean values became gentle or less rapid.
The relationship between the physical properties investigated and the moisture content were mostly quadratic and polynomial in nature except for the sphericity, true density and porosity of the melon seed which were linear in nature.
The regression models developed to establish the relationship between the physical properties of the melon seed and kernel and the moisture content had high correlation coefficients, R 2 except for the sphericity and porosity of the melon seed (0.3943 and 0.6570, respectively) and the porosity of the kernel (0.5110).
At all moisture content levels, plywood showed the highest static coefficient of friction for the melon seed and kernel as the moisture content increased.
Additional information
Funding
Notes on contributors
Okey Francis Obi
The authors are dedicated to conducting innovative and cutting-edge research in two broad research themes: (1) biomass energy and (2) agricultural product processing. These research themes entail biomaterial handling and processing, conversion of biomass feedstocks into fuels and bio-based products, agro-products processing for food and biofuels, and post-harvest handling of agro-products. The activities of the group range from theoretical investigations to experimental research and field tests as well as demonstration programmes and consultation services. The study on the moisture-dependent physical properties of melon (Citrullus colocynthis lanatus) seed and kernel relevant in bulk handling is one of the series of studies on melon fruit aimed at promoting and optimizing its industrial processing. Although, melon (Citrullus colocynthis lanatus) presents large-scale potential, it is still presently processed manually and at micro scale. The manual and micro-scale processing of the melon has been observed to impact negatively on the quality properties of the melon kernel which has necessitated the need for this study.
References
- Akobundu, E. N. T. , Cherry, J. P. , & Simmons, J. G. (1982). Chemical, functional, and nutritional properties of Egusi (Colocynthis citrullus L.) seed protein products. Journal of Food Science , 47 , 829–835.10.1111/jfds.1982.47.issue-3
- Al-Mahasneh, M. A. , & Rababah, T. M. (2007). Effect of moisture content on some physical properties of green wheat. Journal of Food Engineering , 79 , 1467–1473.10.1016/j.jfoodeng.2006.04.045
- Aviara, N. A. , Gwandzang, M. I. , & Haque, M. A. (1999). Physical properties of guna seeds. Journal of Agricultural Engineering Research , 73 , 105–111.10.1006/jaer.1998.0374
- Aydin, C. (2003). Physical properties of almond nut and kernel. Journal of Food Engineering , 60 , 315–320.10.1016/S0260-8774(03)00053-0
- Aydın, C. , & Özcan, M. (2002). Some physico-mechanic properties of terebinth (Pistacia terebinthus L.) fruits. Journal of Food Engineering , 53 , 97–101.10.1016/S0260-8774(01)00145-5
- Bande, Y. M. , Adam, N. M. , Azmi, Y. , & Jamarei, O. (2012). Determination of selected physical properties of Egusi melon (Citrullus Colocynthis Lanatus) seeds. Journal of Basic and Applied Sciences , 8 , 257–265.
- Bart-Plange, A. , & Baryeh, E. A. (2003). The physical properties of category B cocoa beans. Journal of Food Engineering , 60 , 219–227.10.1016/S0260-8774(02)00452-1
- Baryeh, E. A. (2002). Physical properties of millet. Journal of Food Engineering , 51 , 39–46.10.1016/S0260-8774(01)00035-8
- Baryeh, E. A. , & Mangope, B. K. (2002). Some physical properties of QP-38 variety pigeon pea. Journal of Food Engineering , 56 , 59–65.
- Bulent Coşkun, M. , Yalçin, I. , & Özarslan, C. (2006). Physical properties of sweet corn seed (Zea mays saccharata Sturt.). Journal of Food Engineering , 74 , 523–528.
- Cetin, M. (2007). Physical properties of barbunia bean (Phaseolus vulgaris L. cv. ‘Barbunia’) seed. Journal of Food Engineering , 80 , 353–358.10.1016/j.jfoodeng.2006.06.004
- Chandrasekar, V. , & Viswanathan, R. (1999). Physical and thermal properties of coffee. Journal of Agricultural Engineering Research , 73 , 227–234.10.1006/jaer.1999.0411
- Coşkuner, Y. , & Karababa, E. (2007). Physical properties of coriander seeds (Coriandrum sativum L.). Journal of Food Engineering , 80 , 408–416.10.1016/j.jfoodeng.2006.02.042
- Davies, R. M. (2010). Engineering properties of three varieties of melon seeds as potentials for development of melon processing machines. Advance Journal of Food Science and Technology , 2 , 63–66.
- Deshpande, S. D. , Bal, S. , & Ojha, T. P. (1993). Physical properties of soybean. Journal of Agricultural Engineering Research , 56 , 89–98.10.1006/jaer.1993.1063
- Dursun, E. , & Dursun, I. (2005). Some physical properties of caper seed. Biosystems Engineering , 92 , 237–245.10.1016/j.biosystemseng.2005.06.003
- Dutta, S. K. , Nema, V. K. , & Bhardwaj, R. K. (1988). Physical properties of gram. Journal of Agricultural Engineering Research , 39 , 259–268.10.1016/0021-8634(88)90147-3
- Eissa, A. H. , & Gamea, G. R. (2003). Physical and mechanical properties of bulb onions. Miser Journal of Agricultural Engineering , 20 , 661–676.
- Enoch, G. A. , Rose, F. , Harmane, T. A. , Raymond, S. A. , Osmane, C. , & Adam, A. (2008). Importance and practices of bitter melon crops in Sociolinguistic areas in Benin. Biotechnology, Agronomy, Society and Environment Journal , 12 , 393–403.
- Eugene, N. O. , & Gloria, N. A. (2002). Chemical composition of selected Nigerian oil seeds and physicochemical properties of the oil extracts. Journal of Food Chemistry , 77 , 431–437.
- Galedar, M. N. , Jafari, A. , & Tabatabaeefar, A. (2008). Some physical properties of wild pistachio (Pistacia vera L.) nut and kernel as a function of moisture content. International Agrophysics , 22 , 117–124.
- Garnayak, D. K. , Pradhan, R. C. , & Naik, S. N. , & Bhatnagar, N. (2008). Moisture-dependent physical properties of Jatropha seed (Jatropha curcas L.). Industrial Crops and Products , 27 , 123–129.10.1016/j.indcrop.2007.09.001
- Gupta, R. K. , & Das, S. K. (1997). Physical properties of sunflower seeds. Journal of Agricultural Engineering Research , 66 , 1–8.10.1006/jaer.1996.0111
- Hsu, M. H. , Mannapperuma, J. D. , & Singh, R. P. (1991). Physical and thermal properties of pistachios. Journal of Agricultural Engineering Research , 49 , 311–321.10.1016/0021-8634(91)80047-I
- Joshi, D. C. , Das, S. K. , & Mukherjee, R. K. (1993). Physical properties of pumpkin seeds. Journal of Agricultural Engineering Research , 54 , 219–229.10.1006/jaer.1993.1016
- Kaleemullah, S. , & Gunasekar, J. (2002). PH—Postharvest technology. Biosystems Engineering , 82 , 331–338.10.1006/bioe.2002.0079
- Kashaninejad, M. , Mortazavi, A. , Safekordi, A. , & Tabil, L. G. (2005). Some physical properties of pistachio (Pistacia vera L.) nut and its kernel. Journal of Food Engineering , 72 , 30–38.
- Kingsly, A. R. P. , Singh, D. B. , Manikantan, M. R. , & Jain, R. K. (2006). Moisture dependent physical properties of dried pomegranate seeds (Anardana). Journal of Food Engineering , 75 , 492–496.10.1016/j.jfoodeng.2005.04.033
- Konak, M. , Çarman, K. C. , & Aydin, C. (2002). PH—Postharvest technology. Biosystems Engineering , 82 , 73–78.10.1006/bioe.2002.0053
- Koocheki, A. , Razavi, S. M. A. , Milani, E. , Moghadam, T. M. , Abedini, M. , Alamatiyan, S. , & Izadkhah, S. (2007). Physical properties of watermelon seed as a function of moisture content and variety. International Agrophysics , 21 , 349–359.
- Makanjuola, G. A. (1972). A study of some of the physical properties of melon seeds. Journal of Agricultural Engineering Research , 17 , 128–137.10.1016/S0021-8634(72)80023-4
- Milani, E. , Seyed, M. , Koocheki, A. , Nikzadeh, V. , Vahedi, N. , Moeinfard, M. , & Gholamhosseinpour, A. (2007). Moisture dependent physical properties of cucurbit seeds. International Agrophysics , 007 , 157–168.
- Mohsenin, N. N. (1980). Physical properties of plant and animal materials (pp. 51–87). New York, NY: Gordon and Breach Science Publishers.
- Mohsenin, N. N. (1986). Physical properties of plant and animal materials . New York, NY: Gordon and Breach Science Publishers.
- Mwithiga, G. , & Sifuna, M. M. (2006). Effect of moisture content on the physical properties of three varieties of sorghum seeds. Journal of Food Engineering , 75 , 480–486.10.1016/j.jfoodeng.2005.04.053
- Norton, G. (1993). Guna seed analysis . Applied Biochemistry and Food Science Department, The University of Nottingham Press, Nottingham.
- Ogut, H. (1998). Some physical properties of white lupin. Journal of Agricultural Engineering Research , 69 , 237–277.
- Okokon, F. B. , Ekpenyong, E. , Nwaukwa, C. , Akpan, N. , & Abam, F. I. (2010). Impact forces of melon seeds during shelling. Agricultural Engineering International: CIGR Journal , 12 , 182–188.
- Oloko, S. A. , & Agbetoye, L. A. S. (2006). Development and performance evaluation of a melon depodding machine. Agricultural Engineering International (The CIGR E Journal) , 7 , PM 06018.
- Paksoy, M. , & Aydin, C. (2004). Some physical properties of edible squash (Cucurbita pepo L.) seeds. Journal of Food Engineering , 65 , 225–231.10.1016/j.jfoodeng.2004.01.019
- Pliestic, S. , Dobricevic, N. , Filipovic, D. , & Gospodaric, Z. (2006). Physical properties of filbert nut and kernel. Biosystems Engineering , 93 , 173–178.10.1016/j.biosystemseng.2005.11.008
- Pradhan, R. C. , Naik, S. N. , Bhatnagar, N. , & Swain, S. K. (2008). Moisture-dependent physical properties of Karanja (Pongamia pinnata) kernel. Industrial Crops and Products , 28 , 155–161.10.1016/j.indcrop.2008.02.006
- Razavi, S. M. A. , & Milani, E. (2006). Some physical properties of the watermelon seeds. African Journal of Agricultural Research , 1 , 65–69.
- Razavi, S. M. A. , Rafe, A. , Mohammad, A. , & Mohammmadi, M. T. (2007). The physical properties of pistachio nut and its kernel as a function of moisture content and variety Part 11. Gravimetrical properties. Journal of Food Engineering , 81 , 218–225.
- Sacilik, K. , Öztürk, R. , & Keskin, R. (2003). Some physical properties of hemp seed. Biosystems Engineering , 86 , 191–198.10.1016/S1537-5110(03)00130-2
- Sessiz, A. , Esgici, R. , & Kızıl, S. (2007). Moisture-dependent physical properties of caper (Capparis ssp.) fruit. Journal of Food Engineering , 79 , 1426–1431.10.1016/j.jfoodeng.2006.04.033
- Sharma, V. , Das, L. , Pradhan, R. C. , Naik, S. N. , Bhatnagar, N. , & Kureel, R. S. (2011). Physical properties of tung seed: An industrial oil yielding crop. Industrial Crops and Products , 33 , 440–444.10.1016/j.indcrop.2010.10.031
- Shepherd, H. , & Bhardwaj, R. K. (1986). Moisture dependent physical properties of pigeon pea. Journal of Agricultural Engineering Research , 35 , 227–234.10.1016/S0021-8634(86)80060-9
- Shittu, S. K. , & Ndirika, V. I. O. (2012). Development and performance tests of a melon (Egusi) seed shelling machine. Agricultural Engineering International: CIGR Journal , 14 , 157–164.
- Srivastava, A. K. , Mahoney, W. T. , & West, N. L. (1990). The effect of crop properties on combine performance. Transactions of the ASAE , 33 , 0063–0072.10.13031/2013.31295
- Tabatabaeefar, A. (2003). Moisture-dependent physical properties of wheat. International Agrophysics , 12 , 207–211.
- Tavakoli, M. , Tavakoli, H. , Rajabipour, A. , Ahmadi, H. , & Gharib-Zahedi, S. M. T. (2009). Moisture-dependent physical properties of barley grains. International Journal of Agricultural and Biological Engineering , 2 , 84–91.
- Vilche, C. , Gely, M. , & Santalla, E. (2003). Physical properties of quinoa seeds. Biosystems Engineering , 86 , 59–65.10.1016/S1537-5110(03)00114-4