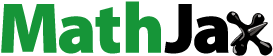
Abstract
Flours of two local cowpea cultivars namely Red cowpea and Black cowpea were analysed for proximate composition, pasting and functional properties. The crude protein and fat contents were higher for Black cultivar than the Red cultivar. The apparent amylose content was significantly higher for Red cowpea (25.54%) than Black cowpea (15.09%). Polyphenols were significantly higher for Red cowpea than Black cowpea. The pasting profile of two cultivars revealed significantly higher values of peak viscosity (1873 cP), setback viscosity (701.00 cP), breakdown viscosity (705.33 cP) and final viscosity (1868.67 cP) for Red cowpea than Black cowpea. Water absorption capacity and oil absorption capacity were found in the range of 1.22–1.39 g/g and 0.71–0.72 g/g, respectively, for the two cultivars. Emulsifying properties varied significantly between the two cultivars. Foaming capacity (198.67%) and foaming stability (84.85%) were significantly higher for Red cowpea than Black cowpea (73.81% and 75.43%). Swelling power was observed significantly higher for Black cowpea than Red cowpea at 90°C. Solubility index was also significantly higher for Black cowpea than Red cowpea throughout the temperature range.
Keywords:
Public Interest Statement
The cowpea flour can be used where functional properties like foaming and emulsification are required. However, its use is not good in foods where refrigerated storage is practiced as it may lead to syneresis in the food.
Competing interests
The authors declare no competing interests.
1. Introduction
Cowpea (Vigna unguiculata/sinensis) is an edible legume belonging to the family Fabaceae (Appiah, Asibuo, & Kumah, Citation2011). It is popularly known by various names such as Southern pea, China pea, Black-eyed bean or Cow gram. It originated in Africa and is widely distributed in tropical and temperate climates and differs in shape, size and colour of seed coat (Ashogbon & Akintayo, Citation2013). Nigeria, the largest producer of Cowpea, where about 2.1 million tonnes are produced per annum accounts for 61% of production in Africa and 58% worldwide (IITA). Cowpea plant is highly valued for its ability to tolerate drought. It is a nutritious crop which provides protein-rich diet at comparatively lower costs than animal proteins and hence is a choice crop in developing countries. Cowpea is rich in potassium with good amount of calcium, magnesium and phosphorus. It also has small amount of iron, sodium, zinc, copper, manganese and selenium. Cowpea is rich in vitamin A and C and also has appreciable amount of thiamin, riboflavin, niacin, vitamin B6 and pantothenic acid (Asare, Agbemafle, Adukpo, Diabor, & Adamtey, Citation2013). Besides, it contains starch, dietary fibre, protective phytochemicals etc.
Like other legumes, cowpea has longer cooking time and some anti-nutritional factors present which restrict its usage rendering it in underutililized category of legumes. Meanwhile, research done on various varieties of cowpea has come up with the claim that it is a potent industrial crop that could be used as an effective functional ingredient in various food systems. Research has thus stressed expanding the utilization of cowpeas in the form of meal and flour (McWatters, Citation1990) for use as functional ingredients in food products. Cowpea seeds can be processed into value added products like protein concentrate and food- grade starch (Ashogbon & Akintayo, Citation2013) since the flour is rich in protein (Mune, Minkaa, & Mbome, 2013) and starch. While it is the nutritional quality that eventually decides the cowpea flour as a food ingredient, its successful performance depends largely on functional characteristics imparted to the final products.
As far as its anti-nutritional factors (trypsin inhibitors, oligosaccharides, etc.) are concerned, these compounds are reduced considerably during processing of the seeds (Odedeji & Oyeleke, Citation2011). The polyphenols present were earlier considered as anti-nutritional factors due to their interaction with proteins that could decrease the protein quality and thereby affect the functional properties. But, they have been found as excellent antioxidants and protective phytochemicals and such beneficial roles of these polyphenols cannot be denied. According to Mune et al. (Citation2013), low levels of polyphenol are desirable. The objective of this work was to investigate the proximate composition, and functional characteristics of flours of two cowpea cultivars (Red cowpea and Black cowpea) of J & K, India.
2. Materials and methods
2.1. Materials
The certified seeds of two cowpea cultivars (Red cowpea and Black cowpea) were procured from the local market of Srinagar, J&K, India. Seeds were cleaned from the dirt, foreign matter and damaged ones.
2.2. Methods
2.2.1. Flour preparation
To produce cowpea flour, whole seeds of each cultivar were ground in a household grinder. The resulting flour was then passed through 212 micrometre sieve. Flour samples were packaged in sealed low-density polyethylene bags and stored until further use at 20°C. All the reagents used in the study were of analytical grade.
2.2.2. Proximate composition
Protein (method 960.10), fat (method 920.85), ash (method 923.03), moisture (method 925.10) contents were determined according to standard methods of Association of Official Analytical Chemists (Citation1990). Carbohydrate was calculated from the difference (100 − % protein + %fat + %ash + %moisture).
2.2.3. Apparent amylose content
Apparent amylose contents of the flour samples were determined by the method of Williams, Wu, Tsai, and Bates (Citation1970). The flour sample (20 mg) was taken, 10 mL of 0.5 M KOH was added and the suspension was mixed thoroughly. The dispersed sample was transferred to a 100 mL volumetric flask and the volume was made up to the mark with distilled water. An aliquot of the test flour solution (10 mL) was pipetted into a 50-mL volumetric flask and 5 ml of 0.1 M aqueous HCl was added followed by 0.5 mL of iodine reagent. The volume was diluted to 50 ml and allowed to stand for 5 min. The absorbance was measured at 625 nm (UV Spectrophotometer, U-2900, Hitachi, Tokyo, Japan). The content of amylose was determined from a standard curve developed using standard amylose and amylopectin blends from potato starch.
2.2.4. Polyphenols
Polyphenol content of two cultivars was evaluated using the method of Chandler and Dodds (Citation1993). 1 g of flour sample, 1 mL of 95% ethanol, 5 mL of distilled water and 05 mL of 50% folin-ciocalteac reagent were added. This mixture was allowed to react for 5 min and then 1 mL of sodium carbonate was added and mixed completely. After 1 h incubation at room temperature, the absorbance of the solution at 725 nm was measured (UV Spectrophotometer, U-2900, Hitachi, Tokyo, Japan). Quantitation was based on the standard curve of gallic acid (10 mg %) which was dissolved in methanol/water (6:40 v/v, 0.3% HCl). The concentration of polyphenols was expressed in terms of mg/100 mL of sample.
2.2.5. Colour
The surface colour of flours was measured using a portable Hunter Lab spectrocolorimeter (Mini Scan XETM, Hunter Associates Laboratory Inc., Reston VA., USA). Colour measurement was done in the values of Hunter L (lightness), a (redness to greenness) and b (yellowness to blueness).
2.2.6. Bulk density
Bulk density was measured as a ratio of mass to volume. A 10 mL graduated cylinder previously tarred was gently filled up to the 10 ml mark with flour. The sample was then packed by gently tapping the cylinder on the bench top from a height of 5 cm until there was no further diminution of the sample level. The weight of the filled cylinder was taken and the bulk density calculated as the weight of sample per unit volume of sample (g/mL).
2.2.7. Swelling & solubility index
Swelling power and solubility of the flours were determined using 2% w/v (dry basis-db) aqueous flour suspension at 60, 70, 80 and 90°C by the method of Wani, Wani, Hussain, et al. (Citation2015).
2.2.8. Syneresis
Flour suspensions (6%, w/w db) were heated at 90°C for 30 min in a water bath (SWB-10L-1-Taiwan) with constant stirring at 75 rpm. The flour samples were stored for 1, 2, 3, 4 and 5th days at 4°C in separate tubes for each day. Syneresis was measured as % amount of water released after centrifugation at 3,000 × g for 10 min (5810R, Eppendoaf, Hamburg, Germany).
2.2.9. Pasting properties
The pasting properties of the flours were measured using a Rapid Visco analyser (Tech Master, Pertain Instruments Pty Ltd, Australia) according to the method described by Wani, Wani, Gani, et al. (Citation2015).
2.2.10. Functional properties
2.2.10.1. Water absorption capacity (WAC) and oil absorption capacity (OAC)
To determine WAC and OAC, 2.5 g (db) of sample was weighed into 25 ml pre-weighed centrifuge tubes and then stirred into 20 ml of double distilled water or mustard oil. The slurry was then centrifuged at 3,000 × g for 10 min (5810R, Eppendoaf, Hamburg, Germany). The water or oil released on centrifugation was drained and gain in weight was expressed as percentage of water/oil absorption capacity (Sofi, Wani, Masoodi, Saba, & Muzaffar, Citation2013).
2.2.10.2. Foaming capacity and stability
Aqueous dispersions (2% w/v) of the flour samples were whipped by an electric blender (Double-M, Germany) for about 3 min and the contents were transferred in a measuring cylinder. After 30 s, the volume of the foam was calculated. The samples were allowed to stand for 60 min at room temperature and the volume of foam after standing was again calculated. The foam capacity and Foam stability were calculated as follows:
2.2.10.3. Emulsifying properties
Emulsifying properties were determined by the method adopted by Sridaran, Karim, and Bhat (Citation2012). 1% flour suspension was homogenized with 5 ml of refined oil. The emulsions were then centrifuged at 1,100 g for 5 min (5810R, Eppendoaf, Hamburg, Germany). Subsequently, the height of the emulsified layer and the total contents in the tube were determined. The emulsion capacity was obtained through the following calculation.
Emulsion stability was evaluated by heating the emulsion for 30 min at 80°C and centrifuging for 5 min at 1,100 g.
2.3. Statistical analysis
Data reported are average of triplicate observations. Means were compared using Paired comparison t test using commercial statistical package SPSS (SPSS, Inc., Chicago, IL, USA).
3. Results and discussions
3.1. Proximate composition of flour
Proximate composition of cowpea flours is presented in Table . The moisture content was found in the range of 9.97–11.11%. There was no significant difference in the moisture contents of two cultivars. Crude protein was observed in the range of 19.6–23.23% and was found significantly higher for Black cultivar (23.23%) than Red cultivar (19.6%). Likewise, crude fat was found significantly higher for Black cultivar (1.94%) than Red cultivar (0.97%). Carbohydrate content was observed significantly higher for Red cultivar (65.52%) than Black cultivar (62.05%). However, significant difference was not observed for ash contents (2.80–2.81%) between the two cultivars. The variations in the proximate composition could be attributed to environmental conditions, type of soil and genetic factors.
Table 1. Proximate composition and colour of cowpea flour (n = 3)
3.2. Amylose content
The amylose content varied significantly for two cultivars (Table ). The amylose content was found in the range of 15.05–25.54%. Red cowpea showed significantly higher amylose content (25.54%) than Black cowpea with amylose content of 15.05%. Results observed are in accordance with Lecuona-Villanueva, Torruco-Uco, Chel-Guerrero, and Betancur-Ancona (Citation2006) who have reported amylose content of 22.9% for Mexican cowpea. Odunayo and Vasudeva (2008) have reported amylose content of the range of 13.9–15.5% and 17.0–18.7% for the whole grain and decorticated flour of two cowpea cultivars.
3.3. Polyphenols
The polyphenol content (Table ) was found significantly higher for Red cultivar (0.27%) than the Black cultivar (0.24%). The polyphenol content was also observed for the seeds of both the cultivars and it was observed that sieved flour had lesser concentrations of polyphenols than their respective seeds [data not reported]. This decrease in polyphenol content in both the cultivars on sieving the flour reveals that polyphenols are concentrated more in seed coats as portion of seed coat got screened out during sieving.
3.4. Colour
Colour values of cowpea flours are presented in Table . Higher value of ‘L’ was observed for Red cowpea flour (85.25) than Black cowpea flour (82.6) indicating that Red cowpea flour is whiter than Black cowpea flour. Red cowpea flour also appeared whiter than Black cowpea flour when seen through naked eye. “a” value in the range from 0.34 to 0.48 and “b” value from 11.38 to 13.14 was observed. Colour of flour is important as per its industrial application because any pigmentation would be carried to the final product in which it is to be used and its quality and thus its acceptance could be reduced.
3.5. Bulk density
Red cowpea flour showed higher bulk density (0.82 g/mL) than Black cowpea flour (0.67 g/mL) as depicted in Table . These values are in accordance with the values obtained by Appiah et al. (Citation2011), who reported comparable bulk density (0.69–0.80 g/mL) for certain varieties of cowpea. Also, Chinma, Emelife, and Alemede (Citation2008) have observed bulk density of 0.29–0.40 g/mL for Nigerian cowpea varieties. Higher bulk density of Red cowpea flour gives indication that it is heavier than Black cowpea and would occupy lesser space per unit weight and hence packaging cost would also be lesser when compared with Black cultivar (Appiah et al., Citation2011). Furthermore, higher bulk density as of Red cowpea flour is desirable for greater ease of dispersibility of flours. In contrast, however, lower bulk density as of Black cowpea flour would be an advantage in the formulation of complementary foods (Appiah et al., Citation2011). Black cowpea flour could also be favourable in infant feeding where less bulk density is desirable (Iwe & Onalope, Citation2001).
3.6. Swelling power
The swelling power was found significantly different for the two cultivars (Table ). The swelling power for both the cultivars was observed to show an increasing trend with the increasing temperature from 60 to 90°C. The Red cowpea showed swelling powers in the range of 4.13–6.93 g/g whereas Black cowpea showed the swelling power in the range of 3.88–8.03 g/g in the temperature range of 60–90°C. On observing swelling powers at temperature 60 and 70°C, Red cowpea showed higher swelling power (4.13 and 4.87 g/g, respectively) than the Black cowpea (3.88 and 4.19 g/g) at the respective temperatures. This could be attributed to the dominance of amylose–protein interaction in the Black cultivar that resulted in complex formation and hence resisted swelling in Black cultivar. This is as per Pomeranz (Citation1991), who stated that formation of protein–amylose complex in native starches and flours may be the cause of decrease in swelling power. However, from 80°C onwards Black cowpea was observed to lead in the swelling power. At 80 and 90°C, the swelling power for Red cultivar was observed 6.51 and 6.93 g/g, respectively, whereas for Black cultivar the respective values are 6.59 and 8.03 g/g. The reason for the higher swelling power of Black cowpea at 80 and 90°C could be attributed to the lower amylose content of Black cultivar than Red cowpea (Clement & Singh, Citation2008). Starch granules become increasingly susceptible to shear disintegration as they swell and starches with lower amylose content (higher amylopectin content) swell more than those with higher amylose content (Ashogbon & Akintayo, Citation2012). Furthermore, according to Moorthy and Ramanujam (Citation1986), the swelling power of granules reflects the extent of the associative forces within the granules. The higher swelling power in Black cultivar thus might be indicative of the weak bonding forces within the starch granules of Black cultivar (Ashogbon & Akintayo, Citation2012). Also, swelling and solubility indices have been stated to provide an evidence of the magnitude of interaction between starch chains within the amorphous and crystalline domains Wani, Sogi, and Gill (Citation2012). The amount of this interaction has been reported to be influenced by the amylose/amylopectin ratio (Blazek & Copeland, Citation2008).
Table 2. Swelling power and solubility index of cowpea flours (n = 3)
Swelling powers showed an abrupt increase for both the cultivars at 80°C, indicating that just after the temperature reaches the onset gelatinization point, the starch granule undergo rapid swelling. This also indicates that high water penetration can be achieved at elevated temperatures (Ikegwu, Okechukwu, & Ekumankana, Citation2010). Similar relationships between swelling behaviour and gelatinization temperature were found for cowpea starch in a study conducted by Junrong et al. (Citation2007).
3.7. Solubility index
Solubility indices also varied significantly between the Red and Black cultivars of cowpea (Table ) which were studied over a range of temperature (60–90°C) and were found in the range of 0.31–0.37 g/g for Red cultivar and 0.34–0.45 g/g for the Black cultivar. Solubility index was found to increase with increase in temperature for both the cultivars of cowpea. Solubility index was significantly higher for Black cultivar throughout the temperature range as compared to Red cowpea cutivar. At 90°C, Black cowpea and Red cowpea showed highest solubility index of 0.45 and 0.37 g/g, respectively. According to Fasasi, Adeyemi, and Fagbenro (Citation2007), high temperature causes disruption of structure of starch granule and leaching out of soluble components that may include soluble sugars, amino acids etc. and thus results in improved solubility.
3.8. Syneresis
The per cent syneresis varied significantly between the two cultivars of cowpea (Table ). Results obtained reveal increase in syneresis with increase in refrigerated storage. The increase in syneresis during storage is due to increased intra- and intermolecular hydrogen bonding that tends to occur between the aggregating chains of starch on fall of temperature, resulting in increased exudation of water from starch gels (Wani et al., Citation2012). Red cowpea showed more water loss rate or syneresis rate than Black cowpea. Red cultivar showed abrupt increase in syneresis after 48 h of refrigeration (51.92% after 24 h and 55.59% after 48 h) which later on increased gradually. After 120 h of refrigeration, syneresis of 58.13% was observed for Red cowpea. Black cowpea flour gel had water loss of 45.49% after 24 h of refrigeration which gradually increased to 50.97% after 120 h of refrigeration. Junrong et al. (Citation2007) have related lower syneresis rate of cowpea starch with the suitability for use in products that are frozen and thawed for consumption. From this we can conclude that Black cultivar would be more acceptable than Red cowpea for industries to use in such products.
Table 3. Syneresis of cowpea flours (n = 3)
3.9. Pasting properties
The peak viscosity which is the ability of starch to swell freely before their physical breakdown varied significantly for the two cultivars and was found in the range of 1,184.67–1,873 cP (Table ). The Red cowpea showed higher value of peak viscosity (1,873 cP) than Black cowpea (1,184.67 cP). The higher value of peak viscosity for Red cowpea flour could be attributed to its higher value of amylose content. Likewise, trough viscosity (hot paste viscosity), breakdown viscosity, setback viscosity and final viscosity varied significantly between the two cultivars and were observed in the range of 1,069.33–1,167 cP, 115.33–705.33 cP, 389.00–701 cP and 1458.33–1,868.67 cP, respectively. The trough viscosity was observed higher for Red cowpea (1,167 cP) than Black cowpea (1,069.33 cP). Trough viscosity has been reported to be influenced by the extent of amylose leaching, amylose–lipid complex formation, friction between swollen granules, granule swelling and competition between leached amylose and remaining granules for free water (Olkku & Rha, Citation1978).
Table 4. Pasting properties of cowpea flour (n = 3)
Trough viscosity was followed by decreased viscosity known as Breakdown viscosity and the values were 705.33 cP for Red cowpea and 115.33 cP for Black cowpea. According to Wani et al. (Citation2012), breakdown viscosity is the measure of the vulnerability of cooked starch to disintegration. The higher the breakdown viscosity, lower is the ability of the starch sample to withstand heating and shear stress during cooking (Adebowale, Adeyemi, & Oshodi, Citation2005). Black cowpea showed more breakdown viscosity than Red cultivar indicating that Black cultivar starch is more susceptible to disintegration upon heating than that of Red cultivar starch. At breakdown, swollen granules disrupt further and amylose molecules leach into solution (Zaidul, Norulaini, Omar, Yamauchi, & Noda, Citation2007). As the temperature falls, leached amylose and amylopectin molecules tend to associate again resulting in setback viscosity. Higher setback value was found for Red cowpea (701.00 cP) than the Black (389.00 cP) and is attributed to the higher amylose content of the Red cultivar. Since setback viscosity is an index of retrogradation, the higher set back value of Red cowpea flour gives indication that it has faster retro gradation tendency than Black cowpea flour, and is favourable for food products such as gluten-free oriental noodles.
The final viscosity also called cold past viscosity is used to define a particular quality of starch and also indicates the stability of cooked starch paste in actual use. The increased final viscosity for both the cultivars could be attributed to aggregation of the amylose molecules on cooling (Kaur, Singh, Ezekiel, & Guraya, Citation2007). On the other hand, differences between the cultivars in final viscosity could be associated with differences in amylose contents. High amylose starches have been found to reassociate more readily than high amylopectin starches. This is because the linear chains (amylose molecules) can orient parallel to each other, moving close enough together to bond (Shimelis, Meaza, & Rakishit, Citation2006).
Pasting temperature refers to the temperature at which a starch starts to thicken. Pasting temperature was found significantly higher for Black cowpea (82.48°C) than the Red cultivar (76.93°C). The higher pasting temperature of Black cowpea than that of Red cultivar indicates that starch granules in Black cultivar resisted swelling during the initial heating. The lower pasting temperature for Red cowpea flour may be related to its higher amylose content. The results obtained are in the accordance with Junrong et al. (Citation2007) observations who have reported that cowpea starch showed much higher pasting temperature (80.7°C) than chickpea (70.9°C) and yellow pea (70.5°C) starches.
3.10. Functional properties
3.10.1. Water absorption and oil absorption capacities
Water and oil absorption capacity values are presented in Table . The water absorption capacities for two flours were significantly different. Red cowpea had a lower water absorption capacity (1.22 g/g) than Black cowpea (1.39 g/g). Appiah et al. (Citation2011) in a study of three cultivars of cowpea have reported water absorption capacity ranging between 1.89 and 2.15 g/g. Similar results have been reported by Chinma et al. (Citation2008) with water absorption capacity varying between 1.60 and 1.94 g/g for certain cowpea cultivars. The lower water absorption capacity of Red cowpea flour may be attributed to lower protein content than Black cowpea (Moure, Sineiro, Domínguez, & Parajó, Citation2006). Increase in water absorption in soy products (flour, concentrate and isolate) with increase in protein content reported by Fleming, Sosulski, Kilara, and Humbert (Citation1974) give the evidence of direct relationship between protein content and water absorption capacity. Also, greater water absorption was noted for protein isolates than for concentrates indicating a relationship between water absorption and protein content. According to Butt and Batool (Citation2010), protein has both hydrophilic and hydrophobic properties, and so can interact with water in foods. The flours of these cowpea cultivars could therefore have functional uses in foods such as sausage production. The major chemical compositions that enhance the water absorption capacity of flours are proteins and carbohydrates, since these constituents contain hydrophilic parts, such as polar or charged side chains.
Table 5. Functional properties of cowpea flour (n = 3)
Oil absorption capacity did not show significant differences between two cowpea cultivars. Oil absorption capacity was 0.71 g/g for Black cowpea and 0.72 g/g for Red cowpea. These results show that both these cultivars may have similar lipid or hydrophobic groups present. Chinma et al. (Citation2008) have reported oil absorption capacity of the range of 0.35 to 0.54 g/g for different Nigerian cowpea cultivars. Appiah et al. (Citation2011) have reported oil absorption capacities for the three cowpea cultivars in the range between 1.95 and 2.31 g/g. The oil absorption capacity makes the flours suitable in food preparations where it helps facilitating appropriate flavour and mouth feel.
3.10.2. Foaming properties
The foaming properties were found significantly different for the two cultivars as presented in Table . Red cowpea flour showed significantly higher foaming capacity (198.67%) and higher foaming stability (84.85%) than the Black cultivar which showed foaming capacity of 73.81% and foaming stability of 75.43%. The higher foaming properties of Red cowpea than Black cowpea could be attributed to the higher albumin fractions in Red cultivar. Furthermore, Black cowpea may have highly ordered globular proteins present that resist the unfolding of the tertiary structure of these proteins at the interface and thus may be less surface active than Red cowpea (Adebowale et al., Citation2005).
Foaming properties are exhibited by the proteins present. The basic requirements of proteins as good foaming agents have been related to the ability to adsorb rapidly at air–water interface during bubbling, undergo rapid conformational change and rearrangement at the interface, and form a cohesive viscoelastic film via intermolecular interactions. The first two factors are vital for better foam ability while the third one is imperative for the stability of the foam (Adebowale et al., Citation2005).
3.10.3. Emulsifying properties
Emulsification properties were found significantly different for the two cultivars of cowpea as shown in Table . Emulsion capacity and emulsion stability were found higher for Red cowpea (35.04 and 96.04%, respectively) than the Black cultivar (34.06 and 73.81, respectively). The difference in emulsion properties between the two cultivars may be attributed to specificity of proteins. According to Denkov, Tcholakova, and Ivanov (Citation2006), there is a considerable effect of heating and aging of the emulsions on their coalescence and flocculation stability. These are controlled by the interactions between the adsorbed protein molecules, such as the formation of disulphide, hydrogen and hydrophobic bonds, and thus are strongly protein specific. The formation and stabilization of emulsions is important for many applications in food products like cake, coffee whiteners and frozen desserts (Adobewale et al., Citation2005).
4. Conclusion
The findings of this study show that the cowpea cultivars (Red and Black cowpea) are rich in proteins and carbohydrates and have good physicochemical properties which could be exploited for nutrition and food formulation. The good functional properties make them useful in many food systems where they could play functional roles. Furthermore, Black cowpea had lower rate of syneresis indicating that it could be used in the food products that require frozen storage and thawed consumption. On the other hand, Red cowpea exhibited excellent foaming capacity and foaming stability indicating its role as aerating agent in food systems that require gas entrapment for maintaining their texture. Also, the water absorption and oil absorption capacity of two cultivars make them useful for various products that require water and oil retention for their textural integrity as in sausages, dough etc. Also, oil retention capability helps retain flavour and provides good mouth feel. Higher retrogradation shown by Red cowpea due to higher setback value makes this cultivar useful for noodle making. Red cowpea flour appears whiter than Black cowpea flour indicating that the former would be more acceptable in the consumer’s eye. Adding to this, these cowpea flours could be used to fortify conventional flours which are low in protein. Consumption of foods formulated or fortified with cowpea flours would be an important step towards relieving protein malnutrition in the poor countries of the world.
Acknowledgement
The authors are thankful to Department of Biotechnology, Government of India, New Delhi.
Additional information
Funding
Notes on contributors
Idrees Ahmed Wani
Idrees Ahmed Wani did his MSc (Food Technology) and PhD (Food Technology) from prestigious Indian Universities. He is presently working as an assistant professor at University of Kashmir, Srinagar (India) and has about 10 years of research experience in the discipline of Food Science and Technology. He has been working on plant polymers especially on starch and proteins. Non-conventional starches like pulse starch, Indian Horse Chestnut starch, Arrowhead tuber starch etc. have been characterized by the author. He has 38 international publications to his credit.
References
- Adebowale, A. , Adeyemi, I. A. , & Oshodi, A. A. (2005). Functional and physicochemical properties of flours of six Mucuna species. African Journal of Biotechnology , 4 , 1461–1468.
- Appiah, F. , Asibuo, J. Y. , & Kumah, P. (2011). Physicochemical and functional properties of bean flours of three cowpea (Vigna unguiculata L. Walp) varieties in Ghana. African Journal of Food Science , 5 , 100–104.
- Asare, A. T. , Agbemafle, R. , Adukpo, G. E. , Diabor, E. , & Adamtey, K. A. (2013). Assessment of functional properties and nutritional composition of some cowpea (Vigna unguiculata L.) genotypes in Ghana. Journal of Agricultural and Biological Science , 8 , 465–469.
- Ashogbon, A. O. , & Akintayo, E. T. (2012). Morphological, functional and pasting properties of starches separated from rice cultivars grown in Nigeria. International Food Research Journal , 19 , 665–671.
- Ashogbon, A. O. , & Akintayo, E. T. (2013). Isolation and characterization of starches from two cowpea (Vigna unguiculata) cultivars. International Food Research Journal , 20 , 3093–3100.
- Association of Official Analytical Chemists . (1990). Official methods of analysis (15th ed.). Washington, DC: Author.
- Blazek, J. , & Copeland, L. (2008). Pasting and swelling properties of wheat flour and starch in relation to amylose content. Carbohydrate Polymers , 71 , 380–387.10.1016/j.carbpol.2007.06.010
- Butt, M. S. , & Batool, R. (2010). Nutritional and functional properties of some promising legumes protein isolates. Pakistan Journal of Nutrition , 9 , 373–379.
- Chandler, S. F. , & Dodds, J. H. (1993). The effect of phosphate, nitrogen and sucrose on the production of phenolics and solasidine in callus cultures of Solanum laciniation . Plant Cell Report , 2 , 105–110.
- Chinma, C. E. , Emelife, I. C. , & Alemede, I. G. (2008). Physicochemical and functional properties of some Nigerian cowpea varieties. Pakistan Journal of Nutrition , 7 , 186–190.10.3923/pjn.2008.186.190
- Clement, A. O. , & Singh, V. (2008). Physico-chemical properties of the flours and starches of two cowpea varieties (Vigna unguiculata (L.)Walp). Innovative Food Science and Emerging Technologies , 9 , 92–100.
- Denkov, N. D. , Tcholakova, S. , & Ivanov, I. B. (2006, October 3–6). Globular proteins as emulsion stabilizers—Similarities and differences with surfactants and solid particles. In The Proceedings of the 4th World Congress on Emulsions . Lyon.
- Fasasi, O. S. , Adeyemi, I. A. , & Fagbenro, O. A. (2007). Functional and pasting characteristics of fermented maize and Nile Tilapia (Oreochromis niloticus) flour diet. Pakistan Journal of Nutrition , 6 , 304–309.10.3923/pjn.2007.304.309
- Fleming, S. E. , Sosulski, F. W. , Kilara, A. , & Humbert, E. S. (1974). Viscosity and water absorption charactercstics of slurries of sunflower and soybean flours, concentrates and isolates. Journal of Food Science , 39 , 188–192.10.1111/jfds.1974.39.issue-1
- Ikegwu, O. J. , Okechukwu, P. E. , & Ekumankana, E. O. (2010). Physichochemical and pasting characteristics of flour and starches from Achi Brachystegia eurycoma seed. Journal of Food Technology , 8 , 58–66.
- Iwe, M. O. , & Onalope, O. O. (2001). Effect of extruded full- fat soy flour into sweet potato flour on functional properties of the mixture. Journal of Sustainable Agriculture and Environment , 3 , 109–117.
- Junrong, H. , Schols, A. H. , Van Soest, J. G. J. , Jin, Z. , Sulmann, E. , & Voragen, A. G. J. (2007). Physicochemical properties and amylopectin chain profiles of cowpea, chickpea and yellow pea starches. Food Chemistry , 101 , 1338–1345.
- Kaur, A. , Singh, N. , Ezekiel, R. , & Guraya, H. (2007). Physicochemical, thermal and pasting properties of starches separated from different potato cultivars grown at different locations. Food Chemistry , 101 , 643–651.10.1016/j.foodchem.2006.01.054
- Lecuona-Villanueva, A. , Torruco-Uco, J. , Chel-Guerrero, L. , & Betancur-Ancona, D. (2006). Physicochemical characterization of Mexican cowpea (Vigna unguiculata) tailing starch. Starch - Stärke , 58 , 25–34.10.1002/(ISSN)1521-379X
- McWatters, K. H. (1990). Functional characteristics of cowpea flours in foods. Journal of the American Oil Chemists’ Society , 67 , 272–275.10.1007/BF02539675
- Moorthy, S. N. , & Ramanujam, T. (1986). Variation in properties of starch in cassava varieties in relation to age of the crop. Starch - Stärke , 38 , 58–61.10.1002/(ISSN)1521-379X
- Mune, M. A. M. , Minkaa, S. R. , & Mbome, I. L. (2013). Chemical composition and nutritional evaluation of a cowpea protein concentrate. Global Advanced Research Journal of Food Science and Technology , 2 , 35–43.
- Odedeji, J. O. , & Oyeleke, W. A. (2011). Comparative studies on functional properties of whole and dehulled cowpea seed flour (Vigna unguiculata). Pakiistan Journal of Nutrition , 10 , 899–902.
- Moure, A. , Sineiro, J. , Domínguez, H. , & Parajó, J. C. (2006). Functionality of oilseed protein products: A review. Food Research International , 39 , 945–963.10.1016/j.foodres.2006.07.002
- Olkku, J. , & Rha, C. K. (1978). Gelatinisation of starch and wheat flour starch—A review. Food Chemistry , 3 , 293–317.10.1016/0308-8146(78)90037-7
- Pomeranz, Y. (1991). Functional properties of food components ( 2nd ed., pp. 27–28). New York, NY: Academic Press.
- Shimelis, E. , Meaza, M. , & Rakishit, S. (2006). Physicochemical properties, pasting behaviour and functional characteristics of flours and starches from improved bean (Phaseolus vulgaris L) varieties grown in East Africa. Agricultural Engineering International , 3 , 1–18, FP 05015.
- Sofi, B. A. , Wani, I. A. , Masoodi, F. A. , Saba, I. , & Muzaffar, S. (2013). Effect of gamma irradiation on physicochemical properties of broad bean (Vicia faba L.) starch. LWT-Food Science and Technology , 54 , 63–72.10.1016/j.lwt.2013.05.021
- Sridaran, A. , Karim, A. A. , & Bhat, R. (2012). Pithecellobium jiringa legume flour for potential food applications: Studies on their physico-chemical and functional properties. Food Chemistry , 130 , 528–535.10.1016/j.foodchem.2011.07.062
- Wani, I. A. , Sogi, D. S. , & Gill, B. S. (2012). Physicochemical properties of acetylated starches from some Indian kidney bean (Phaseolus vulgaris L.) cultivars. International Journal of Food Science and Technology , 47 , 1993–1999.10.1111/ijfs.2012.47.issue-9
- Wani, I. A. , Wani, A. A. , Gani, A. , Muzzaffar, S. , Gul, K. M. , Masoodi, F. A. , & Wani, T. A. (2015). Effect of gamma-irradiation on physico-chemical and functional properties of arrowhead (Sagittaria sagittifolia L.) tuber flour. Food Bioscience , 11 , 23–32.10.1016/j.fbio.2015.04.003
- Wani, A. A. , Wani, I. A. , Hussain, P. R. , Gani, A. , Wani, T. A. , & Masoodi, F. A. (2015). Physicochemical properties of native and γ-irradiated wild arrowhead (Sagittaria sagittifolia L.) tuber starch. International Journal of Biological Macromolecules , 77 , 360–368.10.1016/j.ijbiomac.2015.03.012
- Williams, V. R. , Wu, W. T. , Tsai, H. Y. , & Bates, H. C. (1970). Varietal differences in amylose content of rice starch. Journal of Agriculture Food Chemistry , 119 , 995–999.
- Zaidul, I. , Norulaini, N. , Omar, A. , Yamauchi, H. , & Noda, T. (2007). RVA analysis of mixtures of wheat flour and potato, sweet potato, yam and cassava starches. Carbohydrate Polymers , 69 , 784–791.10.1016/j.carbpol.2007.02.021