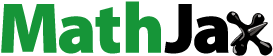
Abstract
Lingonberry (Vaccinium vitis-idaea L.) is a small northern fruit that has been harvested historically by native North Americans and Northern Europeans. These berries have been traditionally consumed as food and for medicinal use. Lingonberries are reported to be rich in vitamin C, benzoic acid, and anthocyanins, which have been implicated in health benefits associated with the consumption of lingonberries. We hypothesized that water-extractable polysaccharides, conjugated with proteins and/or phenolics may also serve as bioactive compounds with potential health benefits in northern Manitoba lingonberries. A hot water extraction was used to obtain water-extractable polysaccharides from lingonberries and the water-extractable polysaccharides were fractionated into water-eluted/neutral and NaCl-eluted/acidic fractions using chromatography. The water-extractable polysaccharides were evaluated for their chemical composition and structural features. The antioxidant activity (FRAP and ABTS assays) and potential of the polysaccharides to serve as α-glucosidase inhibitors were determined. The chemical composition and structural features of the water-extractable polysaccharides were influenced by fraction. The water-extractable polysaccharides and fractions all possessed antioxidant activity, while only the water-extractable polysaccharide conjugates and NaCl-eluted/acidic fraction demonstrated appreciable α-glucosidase inhibition. This work provides novel information indicating that water-extractable polysaccharide conjugates isolated from northern Manitoba lingonberry demonstrate bioactivity.
Public Interest Statement
Overall, this work strives to encourage the promotion of northern Manitoba lingonberry as a food source with beneficial health properties. This work presents information on the fruit quality of northern Manitoba lingonberry and examines whether water-extractable polysaccharide conjugates obtained from Manitoba lingonberry possess bioactive properties. Information on the chemical composition, molecular weight, and structural features from FT–IR spectra along with the in vitro antioxidant activity and α-glucosidase inhibitory activity of water-extractable polysaccharide conjugates and corresponding fractions isolated from Manitoba lingonberries is presented. This work provides information supporting the hypothesis that water-extractable polysaccharide conjugates from northern Manitoba lingonberry possess bioactivities.
Competing interests
The authors declare no competing interest.
1. Introduction
Lingonberry (Vaccinium vitis-idaea L.) is a circumboreal woody, rhizomatous evergreen dwarf shrub that produces bright red berries (Foley & Debnath, Citation2007). Lingonberry exists in two subspecies: the lowland race, V. vitis-idaea ssp. vitis-idaea (L.) Britton is the main subspecies and is found in Europe and Asia; and the dwarf arctic-montane race, V. vitis-idaea ssp. minus (Lodd) Hult is found in Iceland, Greenland, North America, northern Asia, and Scandinavia (Isaak, Petkau, O, Debnath, & Siow, Citation2015). As such, lingonberries are native to Scandinavia, Europe, the USA including Alaska and the Pacific Northwest, and several Canadian provinces such as British Columbia, Manitoba, and Newfoundland–Labrador (Isaak et al., Citation2015; Penhallegon, Citation2006). Lingonberry belongs to the same genus (Vaccinium) as lowbush blueberries (V. angustifolium), highbush blueberries (V. corymbosum L.), and cranberries (V. macrocarpum L.) and can be expected to grow well in regions where blueberries and cranberries are productive (Penhallegon, Citation2006). Lingonberry is a relatively popular fruit crop in Scandinavia where it is grown as a cultivated crop (Heidenreich, Citation2010), however, in North America, lingonberries are not widely cultivated (Lee & Finn, Citation2012). The commercial lingonberry acreage has been reported as 71 acres worldwide (Penhallegon, Citation2006).
Interest in lingonberries is increasing in North America as research has shown that berries in general may provide multiple benefits for cardiovascular, metabolic, brain, and gastrointestinal health and cancer prevention (Torronen, Kolehmainen, Sarkkinen, Mykkanen, & Niskanen, Citation2012). Lingonberry has found historical use as a medicinal food and it has been reported that lingonberries contain vitamin C, vitamin E, benzoic acid, polyphenolic compounds including anthocyanins and dietary fiber (Foley & Debnath, Citation2007). Lingonberry has also been shown to possess high antioxidant activity primarily due to high levels of polyphenolic compounds, including anthocyanins. Prevention of oxidative damage caused by reactive oxygen species and free radicals in the body has been primarily attributed to these polyphenolic compounds (Wang et al., Citation2005). Isaak et al. (Citation2015) have shown that northern Manitoba lingonberry possesses high antioxidant activity and high levels of anthocyanins. The phenolic-rich lingonberry extracts demonstrated protective effects against apoptosis induced by ischemia–reperfusion, which is of relevance for preventing cardiovascular disease, as high levels of oxidative stress and apoptosis characterize myocardial damage (Isaak et al., Citation2015).
There has been increasing interest in finding natural antioxidants (Ibrahim, Mahmoud, & Asker, Citation2014) and it is well established that the phenolic component of plant material may provide benefits to human health due to their antioxidant properties (Herken & Guzel, Citation2010). In recent years, water-extractable polysaccharides, which may be pure or complex in terms of being conjugated with proteins, lipids, or phenolics, have emerged as an important class of compounds present in plant material that possess biological activities (Pawlaczyk et al., Citation2011; Ross & Mazza, Citation2012). The antioxidant activity of water-extractable polysaccharides from cherry, cranberry, and kiwi fruits has been reported (Fan, Mazza, & Liao, Citation2010; Ross, Fukumoto, & Godfrey, Citation2015; Ross, Godfrey, & Fukumoto, Citation2015; Ross et al., Citation2015). The immunomodulating (Ross et al., Citation2015), cardioprotective (Ross et al., Citation2015), and α-glucosidase inhibition properties (Ross, Fukumoto, et al., Citation2015; Ross, Godfrey, et al., Citation2015) of complex water-extractable polysaccharides isolated from cherry, apples, raspberry, and ginseng berry fruit have also been reported. Water-extractable polysaccharides from wolfberry/goji berry (Lycium barbarum) have been reported to possess important bioactive functions, including hypoglycemic and hypolipidemic activities (Luo, Cai, Yan, Sun, & Corke, Citation2004), immunomodulating action (Gan, Zhang, Yang, & Xu, Citation2004), and antioxidant activity (Fan et al., Citation2010; Li, Li, & Zhou, Citation2007) along with the ability to be effective in reducing myocardial injury in ischemia/reperfusion of rat heart (Lu & Zhao, Citation2010).
Diabetes mellitus is a chronic metabolic disease. Oxidative stress and consequent damage to tissues have been identified as common end points of chronic diseases, including diabetes (Cui, Gu, Wang, Ouyang, & Wang, Citation2015). Water-extractable polysaccharides, which again may be complex and conjugated with chemical constituents such as proteins and/or phenolics, have been reported to play an important role as dietary free radical scavengers for preventing oxidative damage and should be explored novel antioxidants (Dou, Meng, Liu, Li, & Ren, Citation2015). α-Glucosidase can control postprandial hyperglycemia by slowing absorption of glucose by inhibition of enzymes that hydrolyze carbohydrates into sugars, such α-glucosidase enzyme. Treatment and control of type 2 diabetes through modulation of postprandial hyperglycemia by therapeutic targeting of inhibition of α-glucosidase has been suggested (Chen, Qu, Fu, Dong, & Zhang, Citation2009; Wang, Citation2011). The identification of natural sources of compounds with the ability to safely inhibit these enzymes has received increasing research efforts (Chen et al., Citation2009; Palanuvej, Hokputsa, Tunsaringkarn, & Ruangrungsi, Citation2009; Zhang et al., Citation2010).
Consumption of whole lingonberries or juice has been reported to improve postprandial glucose levels, due to delayed digestion of sucrose and consequent slower absorption of glucose, in healthy women (Torronen et al., Citation2012). These researchers attributed delayed digestion of sucrose by the enzymatic action of α-glucosidase and consequent slower absorption of glucose. There are reports in the literature of in vitro studies demonstrating that berries and their polyphenols inhibit intestinal a-glucosidase activity and glucose uptake (Hanhineva et al., Citation2010). However, there are also reports in the literature demonstrating that water-extractable polysaccharides inhibit the activity of α-glucosidase (Chen et al., Citation2009; Cui et al., Citation2015; Ross, Fukumoto, et al., Citation2015; Ross, Godfrey, et al., Citation2015; Wang, Yang, & Wei, Citation2010). It therefore appears different components in lingonberry may have similar bioactivity.
This may explain why a diet rich in naturally occurring bioactive compounds, such as phenolics, present in fruits and vegetables is more effective than the same bioactive compounds consumed as purified products or extracts (Boivin et al., Citation2009). Water-extractable polysaccharides may also contribute to the health effects of fruits and other plant-based materials and it should be noted again that the potential health benefits of water-extractable polysaccharides may be due to their conjugation with other compounds. Siu, Chen, and Wu (Citation2014) determined that the antioxidant activity of water-extractable polysaccharides from mushrooms was due to the phenolic and protein components instead of the carbohydrate component. This information connects with the concept of extractable and non-extractable phenolics. Extractable phenolics are phenolic compounds present in either the extracts of food subjected to solvent extraction or gastrointestinal digestate and non-extractable phenolics are phenolic compounds that remain unextracted from the residues of solvent extracted materials or those not released during gastrointestinal digestion and remain associated with the food matrix (Ross, Citation2014; Saura-Calixto, Citation2011). Assessing the bioactivity of complex polysaccharides such as water-extractable polysaccharides that may contain non-extractable phenolics and protein is important as it is essential for fully understanding the food components responsible for the demonstrated health benefits fruits. It likely that the polysaccharide (Pawlaczyk et al., Citation2011; Wang, Citation2011), phenolic component (Saura-Calixto, Citation2012; Siu et al., Citation2014), and protein component (Chen, Zhang, Qu, & Xie, Citation2008; Siu et al., Citation2014) contribute to certain bioactivities that are relevant for human health.
The present work provides information on the fruit quality of Northern Manitoba lingonberry and examines whether water-extractable polysaccharides obtained from Manitoba lingonberry possess bioactive properties. A hot water extraction was used to obtain complex polysaccharides from lingonberries which were separated into water-eluted/neutral and NaCl-eluted/acidic fractions using anion exchange column chromatography with the aim of identifying fractions with enhanced bioactivity This work provides information on the chemical composition, molecular weight, and structural features of FT–IR spectra along with the in vitro antioxidant activity and α-glucosidase inhibitory activity of water-extractable polysaccharides and corresponding fractions isolated from Manitoba lingonberries. This work provides information supporting the hypothesis that water-extractable polysaccharides from Northern Manitoba lingonberry possess bioactivity. This work also aims to encourage the promotion of Northern Manitoba lingonberry as a food source with beneficial health properties.
2. Methods and materials
2.1. Samples
Lingonberries (V. vitis-idaea ssp. minus) from wild populations growing in the Lynn Lake region of northern Manitoba were handpicked by local berry pickers and immediately frozen. Approximately 10 kg of berries were transported frozen from Manitoba to Summerland, BC in September 2013 for testing. A subsample of berries (~500 g) was removed for determination of moisture content, soluble solids content (°Brix), pH, and titratable acidity (% TA). To perform these analyses, ~500 g of berry sample was blended with a handblender (Braun Culinary Series MR 550) with chopper attachment at speed 5 for two 10 s intervals. For moisture content determination, ~15 g of puree was placed into aluminum weighing dishes and placed in a vacuum oven at 70°C for 24 h. The portion of the berry puree not used for moisture content analysis was transferred into 3 × 250-ml centrifuge bottles and centrifuged at 300 rpm for 5 min. The juice was poured off for soluble solids, pH, and titratable acidity (TA) analysis. For soluble solids determination, the refractive indices of the solutions were observed in °Brix temperature-corrected mode on a digital refractometer (Mettler-Toledo, Refracto 30PX, 13/02, LXC13237, Japan). The pH of samples were recorded on a microprobe meter (VWR International, West Chester, PA) after a 3-point calibration with pH 7.01, 4.01, and 10.01 buffers. A pH meter and manual titration was used to measure titratable acidity (TA%) of 10 mL of juice to an endpoint of 8.1 with 0.1 N NaOH. All analyses were performed in triplicate.
For extraction of the complex polysaccharides from the lingonberry, the frozen samples were freeze-dried (Virtis Freeze Dryer, Model 50-SRC-5, Gardiner, NY), placed in polyethylene bags and stored at −20°C until being subjected to water extraction. All chemicals for analyses, unless explicitly stated otherwise, were obtained from Sigma-Aldrich Co. (St. Louis, MO).
2.2. Extraction, purification, and fractionation of water-extractable polysaccharides
Water-extractable polysaccharides, which again may be complex and conjugated with proteins and/or phenolics, were isolated from the fruits samples using the method described in Ross, Fukumoto, et al. (Citation2015). A filtration method based on the work of He et al. (Citation2013) and Ross, Fukumoto, et al. (Citation2015) was employed for solid–liquid separations. Figure shows the extraction scheme for obtaining water-extractable polysaccharides from lingonberry samples. Briefly, the freeze-dried material was ground to a fine powder. The ground lingonberry sample was first subjected to an 80% ethanol pre-extraction to remove simple sugars, extractable phenolics, and other low molecular weight compounds. The ethanol extractions were performed three times. The first ethanol extraction was performed at a 1:5 solid-to-solvent ratio for 1 h and subsequent extractions were performed at a 1:2.5 solid-to-solvent ratio for 1 h. The ethanol-extracted fruit residue was ground using a hammer mill (Ika, Wilmington, NC) equipped with a 2-mm screen and subjected to 70°C water extractions. The warm water extractions were performed 2× at a 1:20 solid-to-solvent ratio for 2 h. Filtration using a nylon filter bag (55 μm pore size, 76 cm length × 18 cm diameter) and a 40-L bladder press (Hydropress, Bosa Grape Winery Supplies, Burnaby, BC) was used to separate the solid residue from the water solution. The filtrates of the first and second warm water extractions were pooled. Due to large volumes (~20 L), the pooled aqueous filtrates were subjected to freeze-drying to remove the water. The water-soluble freeze-dried solid material was dissolved in 90% ethanol at a 1:75 (solids:ethanol) ratio and was mixed for 3 h with a mixer, (Lightnin, Rochester, NY). The mixture was kept overnight at 4°C to precipitate the polysaccharides. Separation of the insoluble polysaccharide (IPS) precipitate from the 90% ethanol solvent was performed by filtration through a nylon filter bag. The solid residue/retentate was collected as water-extractable crude polysaccharide (CPS) product and dried in a vacuum oven overnight at 60°C.
Figure 1. Regime for extraction, removal of free proteins, and fractionation of water-extractable polysaccharides from northern Manitoba lingonberry
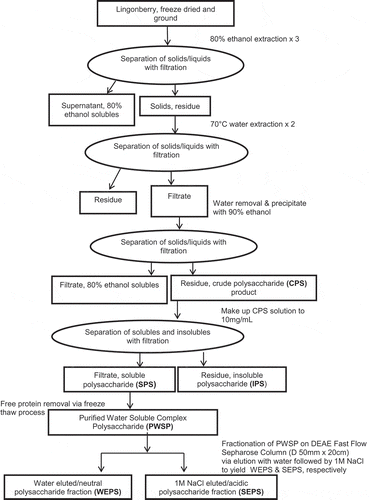
To remove substances potentially insoluble at temperatures lower than the 70°C water extraction temperature and thereby ensure solubility at conditions (temperature and concentrations) used for chemical characterization and bioactivity testing, the CPS product was dissolved in deionized distilled water at a concentration of 10 mg mL−1 and the solution was covered and stirred for 16–24 h. After stirring, the solution was filtered through a nylon filter bag (55 μm pore size, 76 cm length × 18 cm diameter). Both the filtrate and solid residue/retentate was collected. The retentate was gel-like (i.e. not solid) and was termed the IPS product. The filtrate was freeze-dried to obtain a water-soluble complex polysaccharide (SPS) product.
In accordance with He et al. (Citation2013), Ross, Fukumoto, et al. (Citation2015), and Yang, Zhao, Wang, Wang, and Mei (Citation2005), the SPS product was subjected to a freeze–thaw process to remove any free protein that may have been associated with the water-extractable soluble polysaccharide. The SPS product was redissolved at a 1% concentration in distilled water and was mixed for 16 h to ensure dissolution. The solution was poured into plastic bags and was frozen on a tray at −20°C. After freezing for 24 h, the bags were removed and thawed for 24 h. This freeze–thaw process was performed 10 times. Upon the final thawing stage, the aqueous SPS solution was filtered through 55um nylon filter sheet fitted to a Buchner funnel under vacuum. The retentate/residue contained any free proteins that were removed from the freeze–thaw process. The filtrate contained the purified water-soluble complex polysaccharides (PWSP) and was freeze-dried. After freeze-drying, the PWSP was vacuum-dried overnight at 60°C to ensure complete dryness. The yield of the PWSP was determined. In preparation for chemical characterization and bioactivity testing, the PWSP product was cryomilled for 1.5 min (Cryomill, SpexCerti Prep, Model 6800-115) to obtain a product with uniform particle size. After cryomilling, the samples were placed in polyethylene bags and stored at −20°C.
Fractionation of the purified water-soluble complex polysaccharides (i.e. PWSP product) was performed using a medium-pressure chromatography system (BioLogic DuoFlow Chromatography System, Bio-Rad Laboratories, USA) using the method based on the work of Ross, Godfrey, et al. (Citation2015) and Sun, Liu, and Kennedy (Citation2010). Samples were made up at 1 mg mL−1 concentration and fractionated by anion exchange chromatography on a DEAE Sepharose Fast Flow column (inside diameter 50 mm, length 20 cm). The column was first equilibrated with water (750 mL or 5 column volumes). Sample, 950 mL at a concentration of 1 mg mL−1, was loaded onto the column. The column was first eluted with water (750 mL or 5 column volumes) and followed by a stepwise change from water (0 M NaCl) to 1 M NaCl (1,500 mL or 10 column volumes) using a flow rate of 5 mL min−1. The water-eluted/neutral polysaccharide (WEPS) and NaCl-eluted/acidic polysaccharide (SEPS) fractions were collected with a fraction collector. The fractions were collected as 30 mL volumes in each tube. The carbohydrate content of the tubes was measured at 490 nm using the phenolic sulfuric acid method (DuBois, Gilles, Hamilton, Rebers, & Smith, Citation1956) and the protein content of each tube was determined using the Bradford’s Coomassie Brilliant Blue method (Bradford, Citation1976). The fractions containing carbohydrates were pooled and dialyzed using dialysis tubing (Spectra/Por) with a molecular weight cut-off (MWCO) of 1,000 Da. The tubing dimensions were 45 × 29 mm. The length of the tubing used was 90 cm as the capacity of the tubing was 6.4 mL cm−1. The water was changed every 6–16 h and four water changes were used during the dialysis period. Upon completion of the dialysis, the fractions were freeze-dried. After freeze-drying, the fractions were cryomilled (Cryomill, SpexCerti Prep, Model 6800-115) to obtain a product with uniform particle size. After cryomilling, the samples were placed in polyethylene bags and stored at −20°C until chemical and bioactivity analysis.
2.3. Chemical analyses
2.3.1. Carbohydrate content and uronic acid determination
As described by Ross, Fukumoto, et al. (Citation2015), Ross, Godfrey, et al. (Citation2015), and Ross et al. (Citation2015), total carbohydrates content in the complex polysaccharide samples was assayed with the phenol-sulfuric acid method of DuBois et al. (Citation1956) using glucose as a standard. Uronic acid content of the isolated polysaccharides was quantified by the Scott method (Scott, Citation1979) as described by Ross, Fukumoto, et al. (Citation2015), Ross, Godfrey, et al. (Citation2015), Ross et al. (Citation2015). The uronic acid content was determined by the difference in absorbance at 400 and 450 nm and comparing it to a standard curve of galacturonic acid. Experiments were performed in duplicate and results are expressed on a dry weight basis.
2.3.2. Neutral sugars monomer content determination
The neutral sugar monomer content of the complex polysaccharide samples was determined using the methods of Blakeney, Harris, Henry, and Stone (Citation1983) and Fan et al. (Citation2010). Briefly, as described by Ross, Fukumoto, et al. (Citation2015), Ross, Godfrey, et al. (Citation2015), and Ross et al. (Citation2015), the samples were first hydrolyzed with 1 M H2SO4 at 100°C (212°F) for 3 h in a convection laboratory oven into monosaccharides before derivitization into alditol acetates. The alditol acetate derivatives were analyzed via gas–liquid chromatography on an Agilent DB-225 glass-capillary column (30 m × 0.32 mm, i.d., film: 0.25 μm) in an Agilent 6890 N Network GC System (Agilent Technologies, Mississauga, ON), equipped with a FID detector. Different concentrations of neutral sugar standard mixtures (rhamnose (Rha), fucose (Fuc), arabinose (Ara), xylose (Xyl), mannose (Man), galactose (Gal), and glucose (Glu)) were prepared, converted to their derivatives, and analyzed. Sugar concentrations were calculated as the anhydro sugars relative to myo-inositol (used as an internal standard). Experiments were performed in duplicate and results are expressed on a dry weight basis.
2.3.3. Protein content determination
Protein content was estimated from the nitrogen content of the complex polysaccharide samples using the method of Tamaki and Mazza (Citation2010). Briefly, as described by Ross, Fukumoto, et al. (Citation2015), Ross, Godfrey, et al. (Citation2015), Ross et al. (Citation2015), nitrogen content was determined by combusting the dried samples at 950°C using a Leco FP-528 nitrogen analyzer (Leco Corporation, St. Joseph, MI). Approximately 100 mg of a sample was analyzed. Ethylenediaminetetraacetic acid (EDTA) was used as the standard. Protein content was estimated by multiplying the nitrogen content (%) by a factor of 6.25. Experiments were performed in duplicate and results are expressed on a dry weight basis.
2.3.4. Total phenolics
The total phenolics content of the complex polysaccharide samples was determined by the Folin-Ciocalteu colorimetric method based on the procedure described by Singleton and Rossi (Citation1965). As described by Ross, Fukumoto, et al. (Citation2015), Ross, Godfrey, et al. (Citation2015), and Ross et al. (Citation2015), quantification was determined based on a standard curve for gallic acid by measuring absorbance at 765 nm on a spectrophotometer (Cary 50, Agilent Technologies, Mississauga, ON). The total phenolic content was expressed as mg gallic acid equivalent per gram polysaccharide sample (mg GAE g−1 polysaccharide sample). Experiments were performed in duplicate and results are expressed on a dry weight basis.
2.3.5. Molecular weight determination
The molecular weight of the complex polysaccharide samples was determined with high-performance size-exclusion chromatography (HPSEC). As described by Ross, Fukumoto, et al. Citation2015), Ross, Godfrey, et al. (Citation2015), and Ross et al. (Citation2015), molecular weights of polysaccharides were estimated by comparison of the retention/elution times with those of the dextran and glucose standards by use of a standard curve which is in agreement with the method of Carnachan, Bootten, Mishra, Monro, and Sims (Citation2012). The standard curve represented the linear relationship of retention time and the logarithm of the respective molecular weights (Log MW) of the standards. In order to ensure the strongest linear relationship (i.e. R 2 value), a standard curve containing the larger molecular weights ranging from 6,300–43 kDa (R 2 = ~0.99) and a standard curve containing the smaller molecular weights ranging from 43–0.18 kDa (R 2=~0.98) were used for molecular weight calculations. Experiments were performed in duplicate.
2.3.6. FT–IR analysis
FT-IR analysis was performed using the methods of Tamaki and Mazza (Citation2011). Briefly, as described by Ross et al. (Citation2015), all of the Fourier transform mid-infrared (FT-IR) spectra were measured using a Nicolet 380 spectrometer (Thermo Fisher Scientific Inc., Madison, WI) with SMART iTR diamond-attenuated total reflectance with a 45° incident angle generating one bounce. The spectrometer was equipped with a deuterated triglycine sulfate detector scanning over the wavenumber range of 4,000–650 cm−1 at a resolution of 4 cm−1. For each spectrum, a total of 32 repetitive scans was accumulated using OMNIC 8.0 software (Thermo Fisher Scientific Inc.). For analysis, approximately 2–3 mg of polysaccharide sample was placed on the head of the ATR crystal (2 mm diameter) and pressed using a pressure tower. Spectra were collected in triplicate for each sample and then averaged to one spectrum.
2.4. Bioactivity tests
2.4.1. Antioxidant activity-chemical in vitro assays
The antioxidant activity of the polysaccharides was investigated by the Ferric Reducing Antioxidant Power (FRAP) assay and the ABTS•+ radical scavenging assay, respectively. The methods were provided by Ross, Fukumoto, et al. (Citation2015), Ross, Godfrey, et al. (Citation2015), and Ross et al. (Citation2015). Briefly, for both of these assays, 5 mg mL−1 solutions of the complex polysaccharide samples were prepared and mixed on a stirplate overnight. Solutions were frozen at −20°C until the assays could be performed. The thawed samples were then centrifuged at 13,400 rpm for 5 min in Eppendorf Minispin (Eppendorf, Mississauga, ON) and the supernatant was recovered for testing antioxidant activity. The supernatant of each aqueous polysaccharide supernatant was serially diluted for use in the antioxidant assays. Trolox made up in 10% ethanol at various concentrations was used as the standard.
2.4.1.1. ABTS•+ radical scavenging activity
The radical scavenging activity of the complex polysaccharides was determined using the ABTS (2,2′-azino-bis(3-ethylbenzothiazoline-6-sulfonic acid) decolourization assay as described by Mateos-Aparicio, Mateos-Peinado, Jiménez-Escrig, and Rupérez (Citation2010). As described by Ross, Fukumoto, et al. (Citation2015), Ross, Godfrey, et al. (Citation2015), and Ross et al. (Citation2015) for analysis, 50 μL of the aqueous polysaccharide supernatant was added to a well on a 96-well plate. To each polysaccharide sample in each well, 250 μL of the diluted ABTS•+ solution was added. After incubating for 5 min at room temperature, the absorbance at 734 nm was measured using a plate reader (SpectraMax M2, Molecular Devices, Sunnyvale, CA). Absorbance was measured for each sample in triplicate for each dilution. The results were expressed as Trolox equivalents (TE)/g polysaccharide on a dry weight basis.
2.4.1.2. Ferric reducing antioxidant power (FRAP)
The antioxidant activity, in terms of ferric-reducing antioxidant (FRAP), of the complex polysaccharides was evaluated using the method of Mateos-Aparicio et al. (Citation2010). As described by Ross, Fukumoto, et al. (Citation2015), Ross, Godfrey, et al. (Citation2015), and Ross et al. (Citation2015), for analysis, 50 μL of the aqueous polysaccharide supernatant was added to a well on a 96-well plate. To each polysaccharide sample in each well, 250 μL of FRAP was added. After incubating for 5 min at room temperature, the absorbance at 593 nm was measured using a plate reader (SpectraMax M2, Molecular Devices, Sunnyvale, CA). Absorbance was measured for each sample in triplicate for each dilution. The results were expressed as Trolox equivalent (TE)/g polysaccharide on a dry weight basis.
2.4.2. Assay for determination of inhibition of α-glucosidase activity
The assay for determining inhibition of α-glucosidase activity was performed based on the method of Wu et al. (Citation2012) and Zhang et al. (Citation2010), with modifications as described by Ross, Fukumoto, et al. (Citation2015), Ross, Godfrey, et al. (Citation2015). The ability of the purified water-soluble complex polysaccharides and water-eluted/neutral and 1 M NaCl-eluted/acidic fractions to inhibit α-glucosidase activity was assayed using p-nitrophenyl-α-D-glucopyranoside (pNPG) as the substrate, which is hydrolyzed by α-glucosidase to release p-nitrophenol. The formation of p-nitrophenol can be determined by measuring absorbance 405 nm and this absorbance is an indicator of α-glucosidase activity (Zhang et al., Citation2010). Absorbance at 405 nm was measured for each sample in triplicate for each sample. A blank reaction was used without the test sample. Acarbose was used as the positive control inhibitor. As in the work of Ross, Fukumoto, et al. (Citation2015), Ross, Godfrey, et al. (Citation2015), for α-glucosidase activity, the calculation of results is presented as:
For the α-glucosidase activities, the overall results are expressed as % Relative Inhibition which is the inhibition provided by the sample compared to the inhibition provided by 5 mg mL−1 acarbose. As in the work of Ross, Fukumoto, et al. (Citation2015), Ross, Godfrey, et al. (Citation2015), the calculation of these results is presented as:
2.5. Statistical analysis
Statistical analysis was conducted using SAS Institute Inc. Software, version 9.1. Data were subjected to analysis of variance (ANOVA) with replication using the SAS PROC GLM procedure. Least square (LS) means and Least Significant Difference (LSD) at 5% significance level were generated using the SAS PROC GLM procedure.
3. Results and discussion
3.1. Quality parameters of northern Manitoba lingonberry
Table shows the quality parameters of the Northern Manitoba lingonberry samples. The moisture content, pH, soluble solids (°Brix), and titratable acidity were determined to be 86.2%, 2.51, 12.0, and 3.189, respectively. For comparison, the pH and soluble solids (°Brix) of cranberries which belongs to the same genus as lingonberries (Vaccinium) have been reported to be ~2.5 and ~9.0°Brix, respectively (Harrison, Oomah, Diarra, & Ibarra-Alvarado, Citation2013), while the pH, soluble solids (°Brix), and titratable acidity of blueberies which also belongs to the genus Vaccinium have been reported as ~2.9, ~10.7–12.18 °Brix, and 0.46–0.85%, respectively (Kalt & McDonald, Citation1996; Reque et al., Citation2014). Lingonberry cultivars grown for experimental purposes with the aim of commercial cultivation in the Pacific Northwest of the USA were reported to have °Brix values ranging from ~10–19 °Brix (Finn & Mackey, Citation2006; Penhallegon, Citation2006), along with pH and TA values ranging from 3.1–3.39 and 1.44–2.3%, respectively (Penhallegon, Citation2006). Although wild lingonberries from Northern Manitoba exhibited higher soluble solids, an indication of sugar content, than cranberries and comparable levels to blueberries; the TA, an indication of organic acid content, of these lingonberries were higher than values for blueberry, indicating that Northern Manitoba lingonberries will likely possess a tart/acidic flavor compared to the sweet flavor associated with ripe blueberries. Lingonberries are cultivated commercially in Scandinavia and typically preserved and/or consumed with sugar, which is added for sweetness to lessen the acidic taste caused by organic acids (Torronen et al., Citation2012). Sucrose in the diet induces increases in postprandial blood glucose and insulin concentrations and repeated postmeal hyperglycemia may promote oxidative stress (Torronen et al., Citation2012). This fact makes characterization of the α-glucosidase inhibitory activity of lingonberry particularly relevant.
Table 1. Quality parameters of northern Manitoba lingonberries
3.2. Chemical characterization of northern Manitoba lingonberry water-extractable polysaccharides and corresponding fractions
Table shows the yields and chemical characteristics of the purified water-soluble complex polysaccharides from lingonberry (PWSP) and corresponding water-eluted/neutral (WEPS) and NaCl-eluted (SEPS) fractions. The yield of PWSP was 2.14% (dry matter basis). This yield is comparable to the yields presented by Ross, Godfrey, et al. (Citation2015) for water-extractable polysaccharides obtained from raspberry. The yield of the WEPS and SEPS were 1.56 and 0.57% (dry matter basis), respectively. The water-eluted/neutral fraction was the main component of the purified water-soluble complex polysaccharide at a proportion of 73% (1.56 g WEPS/2.14 g PWSP × 100 = 73%) and the NaCl/acidic fraction was the minor component of the purified water-soluble complex polysaccharide at a proportion of 26% (0.57 g SEPS/2.14 g PWSP × 100 = 26%). The values of the percent of each fraction obtained after purification on DEAE Sepharose column indicate that recovery was near 100%.
Table 2. Yield, total carbohydrates, protein, total phenolics contents and molecular weights of water-extractable polysaccharides from northern Manitoba lingonberry
The total carbohydrate content of the PWSP sample was ~36% and the WEPS and SEPS fractions had total carbohydrate contents of ~23 and 55%, respectively. All of these values were significantly different at p ≤ 0.05. These results are comparable to those obtained by Ross, Godfrey, et al. (Citation2015), which reported that water-eluted/neutral polysaccharide fractions isolated from cherries, raspberries, and ginseng berry pulp all presented the lowest carbohydrates contents (~10–28%) and the corresponding NaCl-eluted fractions possessed the highest carbohydrate contents (~44–72%). The protein content of the PWSP and WEPS samples were not significantly different (p ≤ 0.05) at ~4.7 and 4.9%. The SEPS fraction had significantly lower (p ≤ 0.05) protein content at ~1.5%. These results indicate that the water-extractable polysaccharides obtained from northern Manitoba lingonberry were likely conjugated with protein moieties.
The water-soluble complex polysaccharides along with the water-eluted/neutral and NaCl-eluted/acidic fractions were analyzed for total phenolics content. Table shows that the PWSP samples demonstrated the highest phenolic content (~36 mg GAE/g polysaccharide, p ≤ 0.05), while the WEPS and SEPS fractions contained lower levels of phenolics (p ≤ 0.05). The SEPS fractions contained lower level of phenolics compared to the WEPS fraction, ~2 and 13 mg GAE/g polysaccharide, respectively (p ≤ 0.05). These results are in agreement with those in the literature. Ross, Fukumoto, et al. (Citation2015), Ross, Godfrey, et al. (Citation2015), and Ross et al. (Citation2015) have reported that water-extractable polysaccharides obtained from cherries, apples, raspberries, and ginseng berry pulp contain phenolic compounds. The work of Siu et al. (Citation2014) indicated the presence of phenolics in water-extractable polysaccharides isolated from mushrooms. The phenolics present in water-extractable polysaccharides represent non-extractable phenolics, which are phenolic compounds that remain unextracted in the residues of solvent-extracted materials and remain associated with the food matrix (Ross, Citation2014; Saura-Calixto, Citation2011). The concept of non-extractable phenolics associated with polysaccharides is important in terms of fully defining the bioactive and health-related properties of food (Ross, Citation2014; Saura-Calixto, Citation2011). Based on the literature, is it likely that the polysaccharide (Pawlaczyk et al., Citation2011; Wang, Citation2011), phenolic component (Saura-Calixto, Citation2012; Siu et al., Citation2014) and protein component (Chen et al., Citation2008; Siu et al., Citation2014) contribute to certain bioactivities.
The molecular weight data contained in the high-performance size-exclusion (HPSEC) chromatograms of the purified water-soluble complex polysaccharides and corresponding water-eluted/neutral and NaCl-eluted/acidic fractions are presented in Table . These molecular weights for the PWSP and WEPS samples were associated with multimodal distributions from HPSEC results which indicated heterogeneity, while the molecular weight for the SEPS samples showed a unimodal distribution which indicated homogeneity. All molecular weights were contained within the range of molecular weight standards (6,300–0.18 kDa) tested. The molecular weight values presented represent the main molecular weight population residing in this class size and values in parentheses represent the proportion of population associated with the reported molecular weight. The PWSP and WEPS samples showed the largest molecular weights, 111 and 136 kDa, respectively. The SEPS showed the smallest molecular weight of 21 kDa (p ≤ 0.05).
Table shows results for the neutral sugars content, uronic acid content, and sugar monomer profiles of the water-soluble polysaccharides and corresponding water-eluted/neutral and NaCl-eluted/acidic fractions. The neutral sugars content of the PWSP was the highest of ~48% followed by the neutral sugars content of the WEPS samples (~34%). The SEPS samples exhibited the highest neutral sugars content of ~77%. The PWSP samples presented a uronic acid content of ~31% and the uronic acid content of the WEPS and SEPS fractions were ~36 and 10%, respectively. Unexpectedly, the uronic acid content of the water-eluted/neutral fraction was higher than that of the NaCl-eluted/acidic fractions. This result has been reported in the literature. Pawlaczyk et al. (Citation2011) used anion exchange chromatography to fractionate polysaccharides isolated from the medicinal plant Erigeron canadensis L. and found that some of the fractions eluted at higher salt concentrations contained less uronic acid. Ni et al. (Citation2010) examined the chemical composition of polysaccharides fractionated using anion exchange chromatography from the leaves of Panax ginseng (C.A. Meyer). They found that the acidic fraction eluted at the highest salt concentration contained lower levels of uronic acid and galacturonic acid compared to fractions eluted at lower salt concentrations.
Table 3. Neutral sugars, uronic acid, and sugar monomer composition of water-extractable polysaccharides from northern Manitoba lingonberry
The sugar monomer content of the PWSP and corresponding fractions is presented in Table . All samples contained rhamnose, fucose, arabinose, xylose, mannose, galactose, and glucose. The main sugars found in all of the water-extractable polysaccharides were arabinose, xylose, galactose, and glucose. Arabinose was the main sugar found in each sample and the SEPS sample exhibited the highest amount of arabinose, which accounted for ~62% of the neutral sugars present (p ≤ 0.05). The WEPS sample contained the lowest amount of arabinose which accounted for ~39% of the neutral sugars present (p ≤ 0.05). The PWSP sample contained ~44% arabinose. Glucose was the second most predominant sugar present in the water-extractable polysaccharides with levels ranging from 15.04–26.02%. Glucose was present in the lowest amount (15.04%) in the SEPS sample and the highest amount (26.04%) in the WEPS sample (p ≤ 0.05). Xylose and galactose were present in comparable amounts in all of the water-extractable polysaccharide samples in amounts ranging from 10.33–16.74% and 9.48–14.46%, respectively. The WEPS sample contained the highest amount of xylose (13.92%), while the SEPS sample contained the lowest amount of xylose (10.33%) (p ≤ 0.05). For galactose, the PWSP and WEPS samples contained comparable amounts of galactose (14.05 and 14.46%, respectively), while the SEPS sample contained the lowest amount (9.48%) (p ≤ 0.05). Rhamnose and mannose were present in the water-extractable polysaccharide samples in lower amounts ranging from 1.79–2.34% and 0.28 and 1.38%, respectively. No significant differences were observed between the samples for rhamnose content, but the SEPS sample contained significantly lower levels of mannose (0.28%) Fucose was present in the lower amounts ranging from 0.16–0.43% for all samples and there was no significant differences (p ≤ 0.05) observed between the samples.
3.3. FT-IR spectra of northern Manitoba lingonberry water-extractable polysaccharides and corresponding fractions
FT-IR spectra of the purified water-soluble complex polysaccharides and fractions obtained for lingonberry samples are shown in Figure (A)–(C). All of the water-extractable polysaccharide samples showed bands of absorption around 3,400–3,200 cm−1, which can be attributed to hydroxyl group stretching in hydrogen bonds and is indicative of strong inter and intramolecular interactions between the polysaccharide chains (He, Ru, Dong, & Sun, Citation2012). The N–H bonds of amino groups also show stretching vibrations in this region which may also indicate the presence of proteins (Yi et al., Citation2011). This is in agreement with the chemical composition data presented in Table . The PWSP, WEPS, and SEPS samples, all exhibited absorption peaks around 2,600–2,900 cm−1, which could be attributed to the C–H stretching vibrations (Dou et al., Citation2015; He et al., Citation2012; Yi et al., Citation2011). Only the PWSP and WEPS samples showed absorption bands around 1,730 cm−1, which may correspond to C=O stretching vibrations from the presence of a carboxylic ester band (He et al., Citation2012). This is in agreement with Table which indicates higher amounts of uronic acid in the PWSP and WEPS samples compared to the SEPS sample. The PWSP, WEPS, and SEPS samples, all showed absorption peaks around ~1,600 cm−1 which may be indicative of a protein as bending vibrations of N-H bonds occur at this wavelength (Yi et al., Citation2011). Phenolic compounds also show absorptions at this wavelength due to the aromatic C=C skeletal stretching of an aromatic ring (Sene, McCann, Wilson, & Grinter, Citation1994). All of the samples showed absorptions between 1,220–1,440 cm−1. Absorptions around 1,400 cm−1 are characteristic of an O-H bond in a carboxylic acid functional group. Absorptions around 1,380 cm−1 are characteristic of a symmetrical stretching vibration of a C=O bond in a carboxyl group (Yi et al., Citation2011) or a C–H bond (Sene et al., Citation1994) and absorptions around 1,230 cm−1 are characteristic of O–H bending vibrations in carboxyl groups (Yi et al., Citation2011). All samples presented absorptions in the 1,200–1,000 cm−1 region, which denotes pyranose ring vibrations overlapped with stretching vibrations of (C–OH) side groups and (C–O–C) glycosidic band vibrations (Thetsrimuang, Khammuang, & Sarnthima, Citation2011; Yi et al., Citation2011). Differences in absorptions for the PWSP, WEPS, and SEPS samples were also observed in the 960–650 cm−1 region of the FTIR spectra. Only the SEPS sample demonstrated absorption around 895 cm−1, which may be attributed to a C-H bond in the β configuration (Thetsrimuang et al., Citation2011). All of the samples showed absorptions around 830 and 805 cm−1, while only the PWSP and WEPS samples exhibited absorptions around 760 cm−1. Absorptions around 855–830 cm−1 have been reported to be due to stretching vibrations of α-D glucose, and β-D-or α-D arabinose pyranoses (Yang & Zhang, Citation2009). Absorptions at about 770 cm−1 have been noted to suggest symmetrical ring vibration of D-glucopyranose rings (Yi et al., Citation2011). These results seem to indicate that the presence of D-glucopyranose rings in the PWSP and WEPS samples. The SEPS samples displayed absorption around 891 cm−1, which is indicative of a β-configuration. The SEPS samples also showed absorption around 855–830 cm−1 which is characteristic of β-D-or α-D arabinose pyranoses and may indicate a high proportion of arabinose pyranoses present, which is in agreement with Table . For complete elucidation of chemical structure of the polysaccharides and in order to clearly identify the configurations as α or β, NMR analysis is required.
3.4. Antioxidant activity and inhibition of α-glucosidase activity of northern Manitoba lingonberry water-extractable polysaccharides and corresponding fractions
The results of the antioxidant activity of the water-soluble complex polysaccharides and corresponding water-eluted/ neutral and NaCl-eluted/neutral fractions, as determined by the Ferric Reducing Antioxidant Power (FRAP) assay and the ABTS•+ radical scavenging assay, are presented in Figure (A). Although the absolute values obtained for the antioxidant activities using these assays were different, the trends of antioxidant activity were the same for both assays. The PWSP samples demonstrated the highest antioxidant activities, 215.9 μmole Trolox eq/g polysaccharide (PS), as determined with the ABTS•+ radical scavenging assay, and 101.3 μmole Trolox eq/g polysaccharide, as determined using the FRAP assay (p ≤ 0.05). These values are comparable to those reported by Ross et al. (Citation2015) for water-extractable polysaccharides isolated from raspberry which were 185 μmole Trolox/g polysaccharide using the ABTS•+ radical scavenging assay and 96 μmole Trolox/g polysaccharide as obtained using the FRAP assay. The SEPS samples contained the lowest antioxidant activity which were 8.3 μmole Trolox/g polysaccharide using the ABTS•+ radical scavenging assay and 5.02 μmole Trolox/g polysaccharide as obtained using the FRAP assay (p ≤ 0.05).
Figure 3. Antioxidant activity-ABTS & FRAP (A) and inhibition of α-glucosidase activity (B) of water-extractable polysaccharides from northern Manitoba lingonberry
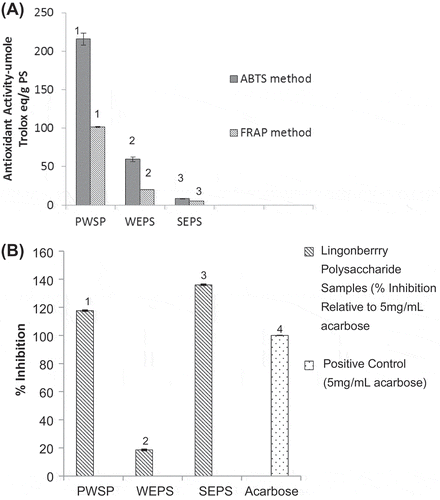
The water-extractable polysaccharides obtained from lingonberry exhibited different antioxidant activities along with different chemical characteristics. Chemical components, molecular mass, structure, conformation, extraction and isolation methods have all been implicated as factors affecting the bioactive properties of polysaccharides (Li et al., Citation2010; Mateos-Aparicio et al., Citation2010; Ross, Godfrey, et al., Citation2015). It is well known that antioxidant activity often correlates with total phenolics content (Thetsrimuang et al., Citation2011) and this was observed in our results. The polysaccharide samples with the highest phenolics content exhibited the highest antioxidant activities. This suggests that phenolic compounds are contributing to the antioxidant activity. Siu et al. (Citation2014) did report a positive relationship between phenolic compounds present in water-extractable polysaccharides from mushrooms and antioxidant activity.
Protein content (Siu et al., Citation2014) and uronic acid content (Chen, Zhang, & Xie, Citation2004) have both been implicated as being directly correlated to antioxidant activity. The direct relationship between both uronic acid content and antioxidant activity along with protein content and antioxidant activity was observed in this work with SEPS samples possessing both the lowest uronic acid and protein contents (p ≤ 0.05) and the lowest antioxidant activity (p ≤ 0.05). However, WEPS samples which had both the highest protein and uronic acid content levels did not show the highest antioxidant activity; the PWSP samples had the highest antioxidant activity with only just slightly lower protein and uronic acid content. The PWSP sample had the highest phenolics content however, which must be more significant in affecting antioxidant activity.
Monosaccharide composition of polysaccharides has been related to antioxidant ability (Tsiapali et al., Citation2001). However, Siu et al. (Citation2014) did not observe this in their work, explicitly noting the direct relationship between both phenolics content and protein content with antioxidant activity but a lack of antioxidant activity associated with the carbohydrate fraction of the water-extractable polysaccharides.
Molecular weight of polysaccharides has also been shown to affect antioxidant activity (Chen et al., Citation2008). Li et al. (Citation2010) noted that low molecular weight polysaccharides extracted from pumpkin demonstrated greater antioxidant activity. This result was not observed in this work as the PWSP and WEPS samples demonstrated the highest molecular weights and the highest antioxidant activities.
Overall, the results from this work denote the importance of the phenolic and possibly protein and uronic acid constituents on the bioactive properties of water-extractable polysaccharides. Water-extractable polysaccharides that are conjugated with phenolics and/or proteins could represent a class of compounds with bioactivity. Although it is noted that the these complex polysaccharides are likely not absorbed at the small intestine during digestion and the phenolics will likely remain associated with the polysaccharides and not become be absorbed at the small intestine and not become bioavailable (Carnachan et al., Citation2012; Ross, Fukumoto, et al., Citation2015), the concept of exposure of the gastrointestinal tract to high levels of bioactive compounds is becoming increasingly more important (Halliwell, Zhao, & Whiteman, Citation2000; Shimizu, Citation2010). The intestinal epithelial cells of the small intestine are continually exposed to food components and modulation of the intestinal functions by bioactive food components is essential in promoting health (Shimizu, Citation2010). Assessing the bioactivity of complex polysaccharides such as those containing non-extractable phenolics and protein is important as it is essential for fully understanding the food components responsible for the demonstrated health benefits of fruits.
Figure (B) shows the inhibitory effects of PWSP, WEPS, and SEPS samples on α-glucosidase activity at a test concentration of 5 mg mL−1. Acarbose was used as a positive control at a test concentration of 5 mg mL−1 and all inhibition measurements were expressed relative to the inhibition of the acarbose which was normalized to 100%. The PWSP and SEPS samples inhibited α-glucosidase activity at levels higher than the acarbose control with levels of ~118 and 136% inhibition determined, while the WEPS sample presented the lowest level (p ≤ 0.05) of α-glucosidase activity inhibition (18.50%). The PWSPS and SEPS samples demonstrating higher levels of α-glucosidase inhibition presented statistically significant lower levels of uronic acid, and xylose acid and statistically significant higher levels of neutral sugars content and arabinose (p ≤ 0.05). However, the differences between the levels of these constituents in the PWSP and WEPS samples were not as striking as the differences between the levels of α-glucosidase inhibition, 118 and 18.5%, respectively. Unlike the antioxidant activity levels, the level of α-glucosidase inhibition was not directly correlated with phenolics content. In fact, the SEPS sample exhibited the lowest phenolics content and the highest level of α-glucosidase inhibition. The work of Zhang et al. (Citation2010) reported that phenolic extracts of certain raspberry varieties showed high levels α-glucosidase inhibition, yet the inhibition of α-glucosidase activity was not correlated with total levels of phenolics. However, the presence of certain phenolic compounds, ellagic acid, cyanidin-diglucoside, pelargonidin-3-rutinoside, and catechin was noted as the reason for raspberries demonstrating α-glucosidase inhibitory activity.
The SEPS sample possessed a lower molecular weight (~21 kDa) compared to PWSP and WEPS samples which possessed comparably high molecular weights (111 and 136 kDa, respectively,) yet both the SEPS and PWSP samples showed high α-glucosidse inhibitory activity. The work of Chen et al. (Citation2009) indicated that the low molecular weight of black tea may have contributed to its high α-glucosidase inhibition activity, yet the work of Cui et al. (Citation2015) showed that the polysaccharides obtained from apricot pulp with higher molecular weights showed higher levels of α-glucosidase inhibition.
A clear correlation between chemical composition and α-glucosidase activity was not identified. It has been noted that many factors of chemical and structural characteristics influence bioactive properties (Wang et al., Citation2010) and more study is required to define the relationship between the chemical composition and structure of complex polysaccharides and bioactivity, including glycosyl linkage analysis and structural elucidation with NMR (Cantu-Jungles et al., Citation2014). It may be beneficial to refractionate the NaCl-eluted/acidic fractions using a different column to further separate the NaCl-eluted/acidic fraction such as reported in the work Siu et al. (Citation2014) and Sun et al. (Citation2010). The influence of the phenolics present in the water-extractable polysaccharides on α-glucosidase inhibitory activity could also be assessed. Mild acid hydrolysis as reported by Pawlaczyk et al. (Citation2011) could be performed to remove the phenolic aglycone component strongly bound (e.g. covalent) from the polysaccharide and identification of the phenolic fraction could be performed. This would help to elucidate the moiety responsible for, or determine whether both components contribute to bioactivity. Further, Moorthy, Ramos, and Fernandes (Citation2012) have stated that aromatic amino acids, especially histidine, phenylalanine, and tyrosine along with the polar (glutamic and aspartic acids) amino acids, may influence α-glucosidase inhibition and more detailed analysis of the protein component in terms of amino acid composition could be performed. Nevertheless, this work provides novel results showing that water-extractable polysaccharides from lingonberry possess α-glucosidase inhibitory activity which is of great relevance in terms of identifying a natural source of α-glucosidase inhibitors for treating/managing type 2 diabetes via control of postprandial hyperglycemia by ultimately retarding absorption of glucose.
4. Conclusion
Quality parameters for northern Manitoba lingonberries were determined and the moisture content, pH, soluble solids (°Brix) and titratable acidity were measured to be 86.2%, 2.51, 12.0, and 3.189, respectively. This work also evaluated and compared the chemical, structural, and molecular characteristics along with the bioactive properties of purified water-soluble complex polysaccharides from Northern Manitoba lingonberry and corresponding water-eluted/neutral and NaCl-eluted/acidic fractions. The polysaccharides, all contained between ~23–55% carbohydrate content and a uronic acid content between ~10–36%, also the water-extractable polysaccharides, all contained protein (~1.5–5%) and phenolic compounds (~2–36 mg GAE/g PS). All of the water-extractable polysaccharides from northern Manitoba lingonberry possessed antioxidant activity and a direct relationship between antioxidant activity and phenolics content was noted. This does suggest that phenolic compounds may be contributing to the antioxidant activity associated with the polysaccharides. Thus, this work shows that phenolics are associated with the water-extractable polysaccharides from northern Manitoba lingonberry and this assessment is essential for fully understanding the food components responsible for the demonstrated health benefits in fruits. These results showed that water-extractable lingonberry polysaccharide conjugates possessed α-glucosidase inhibitory activity, which is of great relevance in terms of identifying a natural source of α-glucosidase inhibitors for treating/managing type 2 diabetes via control of postprandial hyperglycemia by retarding absorption of glucose. A clear correlation between chemical characteristics of the water-extractable northern Manitoba lingonberry polysaccharides and bioactivity could not be established based on the present results as many factors of the chemical characteristics of the studied fractions may influence bioactive properties. In order to define the relationship between these complex polysaccharides and bioactivity, more study is required. However, this work does provide novel information supporting the hypothesis that water-extractable polysaccharide conjugates present in northern Manitoba lingonberry possess bioactivities which may play a role in enhancing human health.
Acknowledgments
The authors are grateful for funding provided by Agriculture and Agri-Food Canada through A-base funding supporting project number 1129-Lingonberry: Characterization and Preserving an Emerging Bioresource for Canada. The Principal Lead for project number 1129 is Dr. Yaw “Chris” Siow of Canadian Centre for Agri-Food Research in Health and Medicine and Agriculture and Agri-Food Canada. The authors are grateful to Dr. Siow for inclusion as collaborators on this project.
Additional information
Funding
Notes on contributors
Kelly A. Ross
Kelly A. Ross joined Agriculture and Agri-Food Canada as a research scientist in October 2008.She received her PhD from the Department of Food Science at Purdue University. Prior to joining AAFC, at the Department of Food Science and the Department of Agricultural and Biological Engineering at the University of Manitoba, she performed her postdoctoral research evaluating chemical components of pulses and determining how storage and processing conditions affected chemical changes and their influence on product quality. Her research interests include: (1) Identification and characterization of novel bioactive polysaccharides in tree fruits and berries; (2) Defining pre-harvest and storage factors that influence fruit attributes (quality and bioactives content); (3) Development and processing of novel agri-products from lower value fruit waste and/or culls.
References
- Blakeney, A. B. , Harris, P. J. , Henry, R. J. , & Stone, B. A. (1983). A simple and rapid preparation of alditol acetates for monosaccharide analysis. Carbohydrate Research , 113 , 291–299. doi:10.1016/0008-6215(83)88244-5
- Boivin, D. , Lamy, S. , Lord-Dufour, S. , Jackson, J. , Beaulieu, E. , Côté, M. , … Béliveau, R. (2009). Antiproliferative and antioxidant activities of common vegetables: A comparative study. Food Chemistry , 112 , 374–380. doi:10.1016/j.foodchem.2008.05.084
- Bradford, M. M. (1976). A rapid and sensitive method for the quantitation of microgram quantities of protein utilizing the principle of protein-dye binding. Analytical Biochemistry , 72 , 248–254. doi:10.1016/0003-2697(76)90527-3
- Cantu-Jungles, T. M. , Maria-Ferreira, D. , da Silva, L. M. , Baggio, C. , Werner, M. F. , Iacomini, M. , … Cordeiro, L. M. C. (2014). Polysaccharides from prunes: Gastroprotective activity and structural elucidation of bioactive pectins. Food Chemistry , 146 , 492–499. doi:10.1016/j.foodchem.2013.09.093
- Carnachan, S. M. , Bootten, T. J. , Mishra, S. , Monro, J. A. , & Sims, I. M. (2012). Effects of simulated digestion in vitro on cell wall polysaccharides from kiwifruit (Actinidia spp.). Food Chemistry , 133 , 132–139. doi:10.1016/j.foodchem.2011.12.084
- Chen, H. , Qu, Z. , Fu, L. , Dong, P. , & Zhang, X. (2009). Physicochemical properties and antioxidant capacity of 3 polysaccharides from green tea, oolong tea, and black tea. Journal of Food Science , 74 , C469–C474. doi:10.1111/j.1750-3841.2009.01231.x
- Chen, H. , Zhang, M. , Qu, Z. , & Xie, B. (2008). Antioxidant activities of different fractions of polysaccharide conjugates from green tea (Camellia sinensis). Food Chemistry , 106 , 559–563. doi:10.1016/j.foodchem.2007.06.040
- Chen, H. X. , Zhang, M. , & Xie, B. J. (2004). Quantification of uronic acids in tea polysaccharide conjugates and their antioxidant properties. Journal of Agricultural and Food Chemistry , 52 , 3333–3336. doi:10.1021/jf0349679
- Cui, J. , Gu, X. , Wang, F. , Ouyang, J. , & Wang, J. (2015). Purification and structural characterization of an α-glucosidase inhibitory polysaccharide from apricot (Armeniaca sibirica L. Lam.) pulp. Carbohydrate Polymers , 121 , 309–314. doi:10.1016/j.carpol.2014.12065
- Dou, J. , Meng, Y. , Liu, L. , Li, J. , & Ren, D. (2015). Purification, characterization and antioxidant activities of polysaccharides from thinned-young apple. International Journal of Biological Macromolecules , 72 , 31–40. doi:10.1016/j.ijbiomac.2014.07.053
- DuBois, M. , Gilles, K. A. , Hamilton, J. K. , Rebers, P. A. , & Smith, F. (1956). Colorimetric method for determination of sugars and related substances. Analytical Chemistry , 28 , 350–356. doi:10.1021/ac60111a017
- Fan, H. , Mazza, G. , & Liao, X. (2010). Purification, composition and antioxidant activity of polysaccharides from wolfberry, cherry, kiwi, and cranberry fruits. Croatian Journal of Food Science and Technology , 2 , 9–17. Retrieved from http://hrcak.srce.hr/index.php?show=clanak&id_clanak_jezik=89739
- Finn, C. E. , & Mackey, T. (2006). Growth, yield, and fruit quality of 10 lingonberry (Vaccinium vitis-idaea) cultivars and selections in the Pacific Northwest, USA. Acta Horticulturae , 715 , 2006. doi:10.17660/ActaHortic.715.42
- Foley, S. L. , & Debnath, S. C. (2007). Influence of in vitro and ex vitro propagation on anthocyanin content and anti-oxidant activity of lingonberries. Journal of Horticultural Science & Biotechnology , 82 , 114–118.
- Gan, L. , Zhang, S. H. , Yang, X. L. , & Xu, H. B. (2004). Immunomodulation and antitumor activity by a polysaccharide–protein complex from Lycium barbarum . International Immunopharmacology , 4 , 563–569. Retrieved from http://www.sciencedirect.com/science/article/pii/S1567576904000517 10.1016/j.intimp.2004.01.023
- Halliwell, B. , Zhao, K. , & Whiteman, M. (2000). The gastrointestinal tract: A major site of antioxidant action?. Free Radical Research , 33 , 819–830. doi:10.1080/10715760000301341
- Hanhineva, K. , Törrönen, R. , Bondia-Pons, I. , Pekkinen, J. , Kolehmainen, M. , Mykkänen, H. , & Poutanen, K. (2010). Impact of dietary polyphenols on carbohydrate metabolism. International Journal of Molecular Sciences , 11 , 1365–1402. doi:10.3390/ijms11041365
- Harrison, j. e. , Oomah, b. d. , Diarra, m. s. , & Ibarra-Alvarado, c. (2013). Bioactivities of pilot-scale extracted cranberry juice and pomace. Journal of Food Processing and Preservation , 37 , 356–365.10.1111/jfpp.2013.37.issue-4
- He, J. Z. , Ru, Q. M. , Dong, D. D. , & Sun, P. L. (2012). Chemical characteristics and antioxidant properties of crude water soluble polysaccharides from four common edible mushrooms. Molecules , 17 , 4373–4387. doi:10.3390/molecules17044373
- He, N. , Shi, X. , Zhao, Y. , Tian, L. , Wang, D. , & Yang, X. (2013). Inhibitory effects and molecular mechanisms of selenium-containing tea polysaccharides on human breast cancer MCF-7 cells. Journal of Agricultural and Food Chemistry, 61 , 579–588. doi: 10.1021/jf3036929
- Heidenreich, C. , (2010). The lowdown on lingonberries. New York Berry News , 9 . Retrieved June 19, 2010, from www.fruit.cornell.edu/berry/production/pdfs/Lingonberries.pdf
- Herken, E. N. , & Guzel, S. (2010). Total antioxidant capacity and total phenol contents of selected commercial fruit juices in Turkey. International Journal of Food Properties , 13 , 1373–1379. doi:10.1080/10942912.2010.499039
- Ibrahim, A. Y. , Mahmoud, M. G. , & Asker, M. M. S. (2014). Anti-inflammatory and antioxidant activities of polysaccharide from Adansonia digitata: An in vitro study. International Journal of Pharmaceutical Sciences Review & Research, 25 , 174–182. Retrieved from www.globalresearchonline.net
- Isaak, C. K. , Petkau, J. C. , & O, K. , Debnath, S. C. , & Siow Y. L. (2015). Manitoba Lingonberry (Vaccinium vitis-idaea) Bioactivities in ischemia-reperfusion injury. Journal of Agricultural and Food Chemistry , 63 , 5660–5669. doi:10.1021/acs.jafc.5b00797
- Kalt, W. , & McDonald, J. E. (1996). Chemical composition of lowbush blueberry cultivars. Journal of American Society Horticultural Science , 121 , 142–146. Retrieved from journal.ashspublications.org/content/121/1/142.full.pdf
- Lee, J. , & Finn, C. E. (2012). Lingonberry (Vaccinium vitis-idaea L.) grown in the Pacific Northwest of North America: Anthocyanin and free amino acid composition. Journal of Functional Foods , 4 , 213–218. doi:10.1016/j.jff.2011.10.007
- Li, J. , Wang, Y , Zhang, D. , Hu, X. , Zhang, Z. , & Xiang, C. (2010). Characterization and bioactivity of water-soluble polysaccharidesfrom the fruit of pumpkin. Journal of Food Agriculture and Environment , 8 , 237–241. Retrieved from http://world-food.net/characterization-and-bioactivity-of-water-soluble-polysaccharides-from-the-fruit-of-pumpkin/
- Li, X. M. , Li, X. L. , & Zhou, A. G. (2007). Evaluation of antioxidant activity of the polysaccharides extracted from Lycium barbarum fruits in vitro . European Polymer Journal , 43 , 488–497. doi:10.1016/j.eurpolymj.2006.10.025
- Lu, S. P. , & Zhao, P. T. (2010). Chemical characterization of Lycium barbarum polysaccharides and their reducing myocardial injury in ischemia/reperfusion of rat heart. International Journal of Biological Macromolecules , 47 , 681–684. doi:10.1016/j.ijbiomac.2010.08.016
- Luo, Q. , Cai, Y. , Yan, J. , Sun, M. , & Corke, H. (2004). Hypoglycemic and hypolipidemic effects and antioxidant activity of fruit extracts from Lycium barbarum . Life Sciences , 76 , 137–149. Retrieved from http://www.sciencedirect.com/science/journal/00243205/76/2 10.1016/j.lfs.2004.04.056
- Mateos-Aparicio, I. , Mateos-Peinado, C. , Jiménez-Escrig, A. , & Rupérez, P. (2010). Multifunctional antioxidant activity of polysaccharide fractions from the soybean byproduct okara. Carbohydrate Polymers , 82 , 245–250. doi:10.1016/j.carbpol.2010.04.020
- Moorthy, N. S. , Ramos, M. J. , & Fernandes, P. A. (2012). Studies on α-glucosidase inhibitors development: Magic molecules for the treatment of carbohydrate mediated diseases. Mini Reviews in Medicinal Chemistry , 12 , 713–720. doi:10.2174/138955712801264837
- Ni, X-Z. , Wang, B-Q. , Zhi, Y. , Wie, N-N. , Zhang, X. , Li, S-S. , … Zhao, J-M. (2010). Total fractionation and analysis of polysaccharides from leaves of Panax ginseng C. A. Meyer. Chemical Research in Chinese Universities , 26 , 230–234. Retrieved from http://www.cjcu.jlu.edu.cn/hxyj/EN/article/downloadArticleFile.do?attachType=PDF&id=12787
- Palanuvej, C. , Hokputsa, S. , Tunsaringkarn, T. , & Ruangrungsi, N. (2009). In vitro glucose entrapment and alpha-glucosidase inhibition of mucilaginous substances from selected Thai medicinal plants. Scientia Pharmaceutica , 77 , 837–849. doi:10.3797/scipharm.0907-17
- Pawlaczyk, I. , Czerchawski, L. , Kuliczkowski, W. , Karolko, B. , Pilecki, W. , Witkiewicz, W. , & Gancarz, R. (2011). Anticoagulant and anti-platelet activity of polyphenolic-polysaccharide preparation isolated from the medicinal plant Erigeron canadensis L. Thrombosis Research , 127 , 328–340. doi:10.1016/j.thromres.2010.11.031
- Penhallegon, R. (2006). Lingonberry production guide for the Pacific Northwest (Oregon State University extension bulletin number PNW 583-E). Corvallis: Oregon State University. Retrieved from https://catalog.extension.oregonstate.edu/pnw583
- Reque, P. M. , Steffens, R. S. , Martins Da Silva, A. , Jablonski, A. , Flores, S. H. , Rios, A. O. , & Vogt de Jong, E. (2014). Characterization of blueberry fruits (Vaccinium spp.) and derived products. Food Science and Technology , 34 , 773–779. doi:10.1590/1678-457X.6470
- Ross, K. A. (2014). Concepts important in understanding the health benefits of phenolics in fruits and vegetables: Extractable and non-extractable phenolics and the influence of cell wall polysaccharides. Research in Health and Nutrition (RHN) , 2 , 29–43. Retrieved from http://www.seipub.org/rhn/paperInfo.aspx?ID=13297
- Ross, K. A. , & Mazza, G. (2012). Conjugated polysaccharides as potential health promoting compounds in berries and cherries. In C. Tuberoso (Ed.), Berries: Properties, consumption and health benefits (pp. 55–81). New York, NY: Nova Science.
- Ross, K. A. , Fukumoto, L. , & Godfrey, D. (2015). Influence of variety, storage, and simulated gastrointestinal digestion on chemical composition and bioactivity of polysaccharides from sweet cherry and apple tree fruits. Cogent Food and Agriculture , 1 , 1062597. doi:10.1080/23311932.2015.1062597
- Ross, K. A. , Godfrey, D. V. , & Fukumoto, L. R. (2015). Purification and characterization of polysaccharides from sweet cherries, raspberries, and ginseng: Chemical composition and bioactivity. Research in Health and Nutrition (RHN) , 3 , 1–13. Retrieved from www.seipub.org/rhn
- Ross, K. A. , Siow, Y. L. , Brown, D. C. W. , Isaak, C. K. , Fukumoto, L. , & Godfrey, D. (2015). Characterization of water extractable crude polysaccharides from cherry, raspberry, and ginseng berry fruits: Chemical composition and bioactivity. International Journal of Food Properties , 18 , 670–689. doi:10.1080/10942912.2013.837066
- Saura-Calixto, F. (2011). Dietary fiber as a carrier of dietary antioxidants: An essential physiological function. Journal of Agricultural and Food Chemistry , 59 , 43–49. doi:10.1021/jf1036596
- Saura-Calixto, F. (2012). Concept and health-related properties of nonextractable polyphenols: The missing dietary polyphenols. Journal of Agricultural and Food Chemistry , 60 , 11195–11200. doi:10.1021/jf303758j
- Scott, R. W. (1979). Colorimetric determination of hexuronic acids in plant materials. Analytical Chemistry , 51 , 936–941. doi:10.1021/ac50043a036
- Sene, C. F. B. , McCann, M. C. , Wilson, R. H. , & Grinter, R. (1994). Fourier-transform Raman and Fourier-transform infrared spectroscopy (An investigation of five higher plant cell walls and their components. Plant Physiology , 106 , 1623–1631. Retrieved from www.plantphysiol.org
- Shimizu, M. (2010). Interaction between food substances and the intestinal epithelium. Bioscience, Biotechnology, and Biochemistry , 74 , 232–241. doi:10.1271/bbb.90730
- Singleton, V. L. , & Rossi, J. A. (1965). Colorimetry of total phenolics with phosphomolybdic–phosphotungstic acid reagents. American Journal of Enology and Viticulture , 16 , 144–158. Retrieved from http://ajevonline.org/content/16/3/144.abstract
- Siu, K.-C. , Chen, X. , & Wu, J.-Y. (2014). Constituents actually responsible for the antioxidant activities of crude polysaccharides isolated from mushrooms. Journal of Functional Foods , 11 , 548–556. doi:10.1016/j.jff.2014.08.012
- Sun, Y.-X. , Liu, J.-C. , & Kennedy, J. F. (2010). Purification, composition analysis and antioxidant activity of different polysaccharide conjugates (APPs) from the fruiting bodies of Auricularia polytricha . Carbohydrate Polymers , 82 , 299–304.10.1016/j.carbpol.2010.04.056
- Tamaki, Y. , & Mazza, G. (2010). Measurement of structural carbohydrates, lignins, and micro-components of straw and shives: Effects of extractives, particle size and crop species. Industrial Crops and Products , 31 , 534–541. doi:10.1016/j.indcrop.2010.02.004
- Tamaki, Y. , & Mazza, G. (2011). Rapid determination of carbohydrates, ash, and extractives contents of straw using attenuated total reflectance Fourier transform mid-infrared spectroscopy. Journal of Agricultural and Food Chemistry , 59 , 6346–6352. doi:10.1021/jf200078 h
- Thetsrimuang, C. , Khammuang, S. , & Sarnthima, R. (2011). Antioxidant activity of crude polysaccharides from edible fresh and dry mushroom fruiting bodies of Lentinus sp. strain RJ-2. International Journal of Pharmacology , 7 , 58–65. doi:10.3923/ijp.2011.58.65
- Torronen, R. , Kolehmainen, M. , Sarkkinen, E. , Mykkanen, H. , & Niskanen, L. (2012). Postprandial glucose, insulin, and free fatty acid responses to sucrose consumed with blackcurrants and lingonberries in healthy women. American Journal of Clinical Nutrition , 96 , 527–533. doi:10.3945/ajcn.112.042184
- Tsiapali, E. , Whaley, S. , Kalbfleisch, J. , Ensley, H. E. , Browder, I. W. , & Williams, D. L. (2001). Glucans exhibit weak antioxidant activity, but stimulate macrophage free radical activity. Free Radical Biology & Medicine , 30 , 393–402. doi:10.1016/S0891-5849(00)00485-8
- Wang, B. (2011). Chemical characterization and ameliorating effect of polysaccharide from Chinese jujube on intestine oxidative injury by ischemia and reperfusion. International Journal of Biological Macromolecules , 48 , 386–391. doi:10.1016/j.ijbiomac.2010.12.005
- Wang, S. Y. , Feng, R. , Bowman, L. , Penhallegon, R. , Ding, M. , & Lu, Y. (2005). Antioxidant activity in lingonberries (Vaccinium vitis-idaea L.) and its inhibitory effect on activator protein-1, nuclear factor-κb, and mitogen-activated protein kinases activation. Journal of Agricultural and Food Chemistry , 53 , 3156–3166. doi:10.1021/jf048379m
- Wang, Y. , Yang, Z. , & Wei, X. (2010). Sugar compositions, α-glucosidase inhibitory and amylase inhibitory activities of polysaccharides from leaves and flowers of Camellia sinensis obtained by different extraction methods. International Journal of Biological Macromolecules , 47 , 534–539. doi:10.1016/j.ijbiomac.2010.07.007
- Wu, C. , Shen, J. , He, P. , Chen, Y. , Li, L. , Zhang, L. , … Deng, Y. (2012). The α-glucosidase inhibiting isoflavones isolated from Belamcanda chinensis leaf extract. Natural Products , 6 , 110–120. Retrieved from www.acgpubs.org/RNP
- Yang, L. , & Zhang, L.-M. (2009). Chemical structural and chain conformational characterization of some bioactive polysaccharides isolated from natural sources. Carbohydrate Polymers , 76 , 349–361. doi:10.1016/j.carbpol.2008.12.015
- Yang, X. , Zhao, Y. , Wang, Q. , Wang, H. , & Mei, Q. (2005). Analysis of the monosaccharide components in angelica polysaccharides by high performance liquid chromatography. Analytical Sciences , 21 , 1177–1180. doi:10.2116/analsci.21.1177
- Yi, Y. , Liao, S. T. , Zhang, M. W. , Shi, J. , Zhang, R. F. , Deng, Y. Y. , & Wei, Z. C. (2011). Physicochemical characteristics and immunomodulatory activities of three polysaccharide-protein complexes of longan pulp. Molecules , 16 , 6148–6164.10.3390/molecules16076148
- Zhang, L. , Li, J. , Hogan, S. , Chung, H. , Welbaum, G. E. , & Zhou, K. (2010). Inhibitory effect of raspberries on starch digestive enzyme and their antioxidant properties and phenolic composition. Food Chemistry , 119 , 592–599. doi:10.1016/j.foodchem.2009.06.063