Abstract
Macadamia is well known for its high-quality kernel; however, its skin and husk, known as waste, have had limited uses as by-products. This study aimed to determine the characteristics of the macadamia nut and to examine the impact of different solvents on the recovery of phenolic compounds (TPC), flavonoids, proanthocyanidins and antioxidant properties of the macadamia skin. The results showed that the kernel, the commercial part, only accounts for about 20% of the nut weight; whereas, the skin or the husk, the waste, account for approximately 40%, revealing that macadamia skin is an abundant starting material for the utilisation of by-products. The type of solvents was found to significantly affect the recovery yields of bioactive compounds from the macadamia skin. The combination of organic solvents, methanol, ethanol, acetonitrile and acetone with water (50%, v/v) had the highest recovery yields for TPC, flavonoids and proanthocyanidins as well as the most potent antioxidant properties, followed by absolute methanol, then water. Absolute ethanol, acetonitrile and acetone had the lowest recovery yields. The current study recommended that 50% acetone should be used for the recovery of TPC and flavonoids and 50% ethanol should be used for the extraction of proanthocyanidins from the macadamia skin.
Public Interest Statement
Waste is a major problem of the food industry, thus many attempts have been made to utilise by-products from waste generated from the food industry. Large quantities of waste are generated annually from the macadamia industry; however, limited applications have been developed to utilise by-products from the waste. This study, for the first time, indicates the exact portion of waste from the macadamia and how the solvents used affect the recovery yields of bioactive compounds and antioxidant properties from the waste generated from the skin of the macadamia. These findings can be utilised for further preparation of the extract and isolation of bioactive compounds from the macadamia skin.
Competing interests
The authors declare no competing interests.
1. Introduction
Macadamia is an evergreen, native Australian tree originating from the rainforest regions of Australia’s East Coast. In Australia, there are at least five species of macadamia, but only two species produce edible fruits including Macadamia integrifolia (smooth shelled) and the Macadamia tetraphylla (rough shelled), of which the Macadamia intergrifolia is the species most commonly grown for its fruits (Munro & Garg, Citation2008). Australia is the world’s largest macadamia-producing country generating approximately 40,000 tonnes per year, with approximately 70% of the nut’s weight being skin and husk, which are inedible. These by-products are often treated as waste with little value (Wechsler et al., Citation2011). Although, these by-products have been partially utilised in garden mulching, animal feed fillers, furniture materials and carbon composites for post-combustion CO2 capture, most of these by-products have been disposed of into landfill, which is a cost-prohibitive action through their sheer volume (Bae & Su, Citation2013; Wechsler et al., Citation2011), and thus this abundant source is a great starting material for bioactive compounds (Walton & Wallace, Citation2010).
Bioactive compounds, such as polyphenols and their secondary metabolites flavonoids and proanthocyanidins, derive from plants and have been found to possess potent antioxidant properties and have been associated with various health benefits such as the prevention of cancer, cardiovascular diseases, diabetes and obesities (Vuong et al., Citation2014). Therefore, many attempts have been made to extract and isolate bioactive compounds from plant materials for utilisation in the food and pharmaceutical industries. As bioactive compounds range from very polar to very non-polar compounds, the extraction solvent plays an important role in extraction efficiency of bioactive compounds from the plant materials. However, extraction efficiency of bioactive compounds depends on the type of solvent used and each type of material has a suitable solvent for extraction of the targeted compounds (Pinelo, Rubilar, Jerez, Sineiro, & Núñez, Citation2005; Ye, Liang, Li, & Zhao, Citation2015). Therefore, it is necessary to determine the most suitable solvent for recovery of bioactive compounds from macadamia skin.
Water and other organic solvents such as methanol, ethanol, acetonitrile, acetone, hexane and diethyl ether have usually been applied in the extraction of bioactive compounds from plants. According to the US Food and Drug Administration (FDA, Citation2012), Class 3 solvents such as ethanol, acetone, ethyl acetate and ethyl ether should be used as they are less toxic and of lower risk to human health. Whereas, Class 2 solvents such as acetonitrile, chloroform, hexane and methanol should be limited in pharmaceutical products because of their inherent toxicity (FDA, Citation2012). In the current study, we aimed to analyse the impact of different solvents including water, Class 2 solvents (methanol and acetonitrile), Class 3 solvents (ethanol and acetone) and the combination between these organic solvents with water at a ratio of 50:50 (v/v) on the recovery of total phenolic compounds, flavonoids, proanthocyanidins and antioxidant capacity from the macadamia skin. Results from this study will provide a better understanding on the impact of various common extraction solvents on extraction efficiency of phenolic compounds, and indicate the most suitable solvent for effective recovery of phenolic compounds and antioxidant capacity from the skin of the macadamia.
2. Materials and methods
2.1. Materials
Macadamia (M. tetraphylla) nuts (Figure ) were collected in July 2014 in the Central Coast region, New South Wales, Australia (latitude of 33.4°S, longitude of 151.4°E). Once harvested, the skin of the nuts was removed and then immediately frozen in liquid nitrogen and freeze dried (FD3 freeze dyer, Thomas Australia Pty. Ltd., Seven Hills, NSW, Australia) to minimise oxidation and degradation of bioactive compounds. The dried skin was then ground using a commercial blender (John Morris Scientific, Chatswood, NSW, Australia). The dried skin was sieved using a steel mesh sieve (1.4 mm EFL 2000; Endecotts Ltd., London, England). The dried, ground skin was kept in a sealed and labelled container at 5°C for further analysis.
2.2. Methods for characterisation of macadamia (M. tetraphylla) nuts
2.2.1. Moisture content
Moisture content of the fresh nut, skin, husk and kernel was determined by drying the fresh nuts in a vacuum oven set at 90°C for 24 hours, and the moisture was calculated based on weight difference and expressed as percentage and standard deviation.
2.2.2. Weight of fresh nut, skin, husk or kernel
The weight of the nut, or its skin, husk or kernel was measured from the 20 randomly selected nuts using a four decimal analytical balance. The nut weight was expressed as mean value (g) and standard deviation.
2.2.3. Fruit and seed diameters
The diameters of fresh nuts and seeds were measured from the 20 randomly selected nuts using the four-decimal thickness gauge. The values were expressed as mean (mm) and standard deviation.
2.3. Extraction process
Nine extraction solvents were used for comparison in the current study, including: water, methanol, ethanol, acetonitrile, acetone, methanol 50%, ethanol 50%, acetonitrile 50% and acetone 50%. Ultrasound-assisted extraction was applied to extract the ground, dried sample at a solvent-to-sample ratio of 100:1 mL/g of dried sample. The extraction chamber was completely immersed into an ultrasonic bath (Soniclean, 220 V, 50 Hz and 250 W, Soniclean Pty Ltd., Thebarton, Australia) with pre-set conditions for temperature of 35°C, time of 30 min and power of 150 W. Agitation was conducted for three seconds once every five minutes using a Vortex. When the ultrasonic extraction was completed, the extracts were immediately cooled on ice to room temperature, filtered using filter paper and diluted 20 times for quantitative analysis and antioxidant determination.
2.4. Methods for determination of chemical properties
2.4.1. Total phenolic content (TPC)
TPC was determined as described by Vuong et al. (Citation2013). To 1 mL of diluted sample 5 mL of 10% (v/v) Folin–Ciocalteu reagent was added, followed by the addition of 4 mL of 7.5% (w/v) Na2CO3, then mixed well on a vortex vibrator for 2 minutes and incubated in the dark at RT for 1 h before the absorbance was measured at 760 nm using a UV spectrophotometer (Varian Australia Pty. Ltd., Victoria, Australia). Gallic acid was used as the standard for a calibration curve and the results were expressed as mg of gallic acid equivalents per g of sample (mg GAE/g).
2.4.2. Total flavonoids
The total flavonoid content was measured as described by Zhishen, Mengcheng, and Jianming (Citation1999). To 0.5 mL of diluted sample 2 mL of H2O and 0.15 mL of 5% (w/v) NaNO2 were added and left at RT for 6 minutes. Then, 0.15 mL of 10% (w/v) AlCl3 was added and left at RT for 6 minutes. It was followed by the addition of 2 mL of 4% (w/v) NaOH and 0.7 mL of H2O with the final solution being mixed well and left at RT for a further 15 minutes before the absorbance was measured at 510 nm using a UV spectrophotometer. Rutin was used as the standard for a calibration curve and the results were expressed as mg of rutin equivalents per g of sample (mg RUE/g).
2.4.3. Proanthocyanidins
The content of proanthocyanidins was determined as described by Li et al. (Citation2006). To 0.5 mL of diluted sample 3 mL of 4% (w/v) of vanillin was added. The mixture was then added to 1.5 mL concentrated HCl and left at RT for 15 minutes before the measurement of absorbance at 500 nm using a UV spectrophotometer. Catechin was used as the standard for a calibration curve and the results were expressed as mg of catechin equivalents per g of sample (mg CE/g).
2.5. Methods for determination of antioxidant properties
2.5.1. ABTS radical scavenging capacity
ABTS radical scavenging activity was determined according the methods described by Thaipong, Boonprakob, Crosby, Cisneros-Zevallos, and Hawkins Byrne (Citation2006) with few modifications. A stock solution was prepared by adding 10 mL of 7.4 mM ABTS solution to 10 mL of 2.6 mM K2S2O8 and left at RT in the dark for 15 hours and then stored at −20°C until required. The working solution was freshly prepared by diluting 1 mL of stock solution with 60 mL of methanol to obtain an absorbance value of 1.1 ± 0.02 at 734 nm. To 0.15 mL of sample 2.85 mL of the working solution was added and left in the dark at RT for 2 hours before its absorbance was read at 734 nm using a UV–VIS spectrophotometer (Cary 50 Bio, Varian Australia Pty. Ltd., Australia). Trolox was used as a standard and the results were expressed as μM trolox equivalents per g of dried sample (μM TE/g).
2.5.2. DPPH radical scavenging activity
The radical scavenging activity was measured based on the method described by Thaipong et al. (Citation2006), with some modifications. A stock solution was prepared by dissolving 24 mg of DPPH in 100 mL of methanol and then stored at −20°C until required. The working solution was then prepared fresh by mixing 10 mL of stock solution with 45 mL of methanol to obtain an absorbance of 1.1 ± 0.02 at 515 nm. To 0.2 mL of sample 3.8 mL of working solution was added and then left under darkness at RT for 3 hours before measuring the absorbance at 515 nm using the UV spectrophotometer. Trolox was used as the standard for a calibration curve and the results were expressed as μM of trolox equivalents per g of sample (μM TE/g).
2.5.3. Cupric-reducing antioxidant capacity (CUPRAC)
CUPRAC was determined as described by Apak, Güçlü, Özyürek, and Karademir (Citation2004) with some modifications. To 1 mL of CuCl2 1 mL of neocuproine and 1 mL of NH4Ac were added and then followed by the addition of 1.1 mL of sample. After mixing well, the mixture was incubated at RT for 1.5 hours before measuring the absorbance at 450 nm using the UV spectrophotometer. Trolox was used as the standard for a calibration curve and the results were expressed as μM of trolox equivalents per g of sample (μM TE/g).
2.5.4. Ferric-reducing antioxidant power (FRAP)
FRAP was measured as described by Thaipong et al. (Citation2006) with some modifications. A working FRAP solution was prepared by mixing 300 mM Acetate buffer, 10 mM TPTZ in 40 mM HCl and 20 mM FeCl3 in the ratio of 10:1:1 and warmed at 37°C in a water bath (Ratek Instruments Pty. Ltd., Victoria, Australia) before using. To 0.15 mL of sample 2.85 mL of the working FRAP solution was added and incubated at RT in the dark for 30 minutes before its absorbance was read at 593 nm. Trolox was used as the standard and the results were expressed as μM trolox equivalents per g of dried sample (μM TE/g).
2.6. Statistical analyses
The one-way ANOVA and the LSD post hoc test were conducted using the SPSS statistical software version 20. Differences between the mean levels of the components in the different experiments were taken to be statistically significant at p < 0.05.
3. Results and discussion
3.1. Characteristics of macadamia (M. tetraphylla) nut
The characteristics of macadamia nuts are shown in Table . The moisture content of the nuts accounts for about 50% of the total fresh weight, of which moisture content of the skin is 20%, followed by the kernel with 37% and the husk with the lowest moisture content at about 27%. It is interesting to note that the kernel, the edible and commercial part, only accounts for approximately 20% of the total weight of the fresh nut. The rest of the nut (80%) is considered waste, indicating a large quantity of waste being generated annually, and of which more than 40% of the total nut weigh is the skin waste. According to the Australian Macadamia Society, there are approximately 40 thousand tons of macadamia produced annually in Australia and 140 thousand tons produced throughout the world (Australian-Macadamia-Society, Citation2015). This means that there are about 80 thousand tons of macadamia skin produced annually in Australia and 280 thousand tons produced worldwide. These results reveal that macadamia skin is an abundant material, which undoubtedly is a great potential starting material for recovery of bioactive compounds for further utilisation.
Table 1. Macadamia (M. tetraphylla) nut characteristics
3.2. Influence of extraction solvents on bioactive compounds
The effect of different solvents on the recovery of TPC is shown in Figure . In general, extraction solvents had a significant impact on the recovery of TPC, and the combined organic solvents and water had higher recovery yield in comparison with the absolute solvent used in this study. Among the absolute solvents, methanol had the highest recovery with approximately 127 mg GAE/g of the dried skin. Methanol was closely followed by water and ethanol with about 56 and 20% of TPC being recovered in comparison with methanol. Both absolute acetone and acetonitrile had the lowest recovery yields of TPC with less than 4% in comparison with methanol.
Figure 2. Impact of solvents on recovery of TPC from macadamia skin
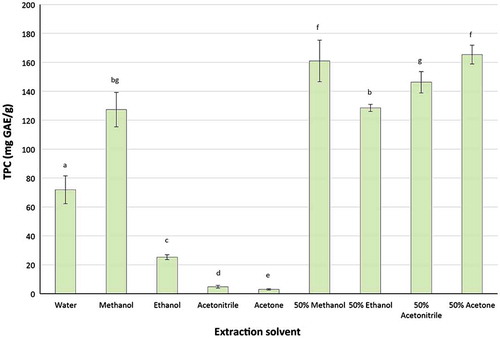
However, in combination with water (50%, v/v), the recovery yield of TPC was significantly improved for every organic solvent used in the current study. Fifty per cent acetone and 50% methanol provided the highest recovery yield with approximately 130% higher than that of absolute methanol. It was followed by 50% acetonitrile and 50% ethanol.
These results are similar to the findings in the previous studies, which reported that extraction solvents significantly affected the recovery yields of TPC from plant materials (Bhebhe, Füller, Chipurura, & Muchuweti, Citation2015; Dhanani, Shah, Gajbhiye, & Kumar, Citation2013; Do et al., Citation2014; Ye et al., Citation2015). However, the recovery of TPC for individual solvents was different. This study found that 50% acetone and 50% methanol had the highest extraction yields, followed by 50% acetonitrile, 50% ethanol and methanol, and acetone had the lowest extraction yield of TPC. Whereas, Singh et al. (Citation2014) found that ethanol had the highest extraction yield of TPC from pomegranate, and Do et al. (Citation2014) reported that absolute acetone and ethanol gave higher extraction yields than 50% acetone or 50% ethanol. These differences can be explained by the different dielectric constants (ε) (H2O, ε = 80; MeOH, ε = 33; acetonitrile, ε = 37.5; acetone, ε = 21; EtOH, ε = 24.55) and different polarities (H2O = 1; MeOH = 0.762; acetonitrile = 0.460; acetone = 0.355; EtOH = 0.654) of the solvents used, which can result in different extraction yields of the phenolic compounds. These findings further confirm that the extraction solvent plays an important role in extractability of phenolic compounds from the materials and each material has a more suitable solvent for extraction of TPC.
Flavonoids are the biggest secondary metabolites of phenolic compounds in plants with more than 4,000 individual identified compounds (D’Archivio et al., Citation2007). Flavonoids have been linked with various health benefits (D’Archivio et al., Citation2007; Kumar & Pandey, Citation2013), thus, it is necessary to determine the impact of solvents on extraction yields of flavonoids for their maximum extraction. The results (Figure ) showed that solvents also significantly affected the extraction yields of flavonoids. A combination of methanol, acetonitrile and acetone with water (50%) had the highest extraction yields, followed by absolute methanol and 50% ethanol. Water had a lower extraction yield than absolute methanol or its combination with ethanol, methanol, acetonitrile and acetone; however, it had higher extraction yield than that of absolute ethanol, acetonitrile or acetone. Addai, Abdullah, and Mutalib (Citation2013) also found that 50% acetone, 50% ethanol and 50% methanol had higher extraction yields of flavonoids than those of absolute acetone, ethanol and methanol as well as water from two papaya cultivars (Hongkong and Eksotika). In addition, Bettaieb Rebey et al. (Citation2012) also reported that 80% methanol, 80% ethanol and 80% acetone extracted more flavonoids than water or absolute methanol, ethanol or acetone. The current study found that water had better extraction yields in comparison with absolute ethanol, acetonitrile and acetone; however, previous studies found that water had lower extraction yields in comparison with those of absolute ethanol, acetonitrile and acetone (Do et al., Citation2014). The difference can be explained by the different polarities of the solvents, which selectively extracted targetable flavonoid compounds from the materials.
Figure 3. Effect of solvents on recovery of flavonoids from macadamia skin
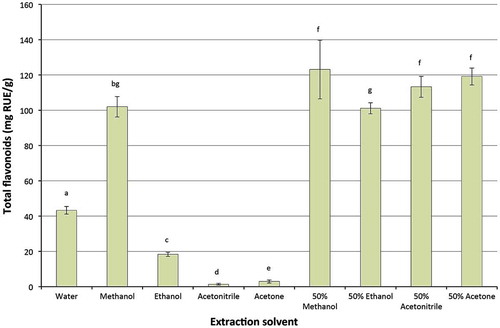
Proanthocyanidins, usually known as condensed tannins, are also abundant and structurally complex subclasses among the flavonoids. They have been found in many fruits and are the most common group of flavonoids in our diet and have been associated with health benefits (Shoji, Citation2014; Vuong et al., Citation2014). The impact of different solvents on extraction yields of proanthocyanidins was therefore investigated and the results are shown in Figure . Methanol and a combination of the tested organic solvents had significantly higher extraction yields than those of water. Absolute ethanol, acetonitrile and acetone had lower extraction yields than that of water. Generally, the findings for proanthocyanidins are similar to those of flavonoids, except the results for 50% ethanol and 50% acetone. Fifty per cent acetone had higher extraction yields of flavonoids than that of 50% of ethanol, whereas 50% of ethanol had higher extraction yields of proanthocyanidins than that of 50% acetone. Variation in extraction yields of proanthocyanidins can be explained by the differences in polarities of the solvents used in the current study.
Figure 4. Effect of solvents on recovery of proanthocyanidins from macadamia skin
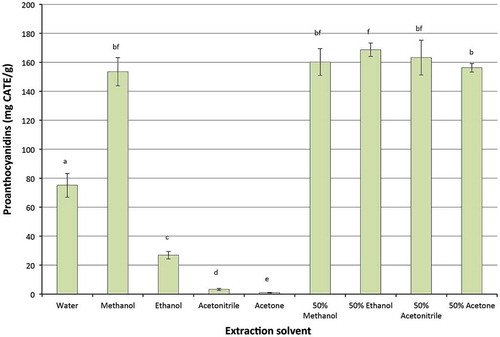
In summary, solvents play an important role in extraction yields of phenolic compounds, flavonoids and proanthocyanidins from the macadamia skin. Overall, 50% combination of organic solvent with water gave the highest recovery yield of phenolic compounds and their second metabolites, followed by absolute methanol, water and absolute ethanol, acetonitrile and acetone had the lowest recovery yields. This study recommends that 50% of methanol and 50% of acetone should be used for extraction of total phenolic compounds and flavonoids, and 50% of ethanol should be used for extraction of proanthocyanidins. According to US Food Drug and Administration (FDA, Citation2012), acetone and ethanol belong to Class 3 chemical group, which is known as a human health hazard at levels normally accepted in pharmaceuticals, whereas methanol is classified as chemical group 2, which should be limited to be used in pharmaceutical products because of their inherent toxicity. Therefore, 50% acetone is suggested to be used for extraction of TPC and flavonoids and 50% ethanol is recommended for extraction of proanthocyanidins.
3.3. Influence of extraction solvents on antioxidant properties
As antioxidant properties of an extract can vary depending on antioxidant assays used for determination, four antioxidant assays were used in the current study to investigate the impact of extraction solvents on antioxidant properties of the extracts from macadamia skin. The results (Figure ) showed that extraction solvents significantly affected antioxidant properties of the extracts. Overall, absolute methanol and combination (50%) of the tested organic solvents with water gave the highest antioxidant properties, followed by water, which gave significantly higher antioxidant properties than absolute ethanol, acetonitrile or acetone. These findings were supported by previous studies on Limnophila aromatic (Do et al., Citation2014), Withnaia somnifera (L.) (Dhanani et al., Citation2013), and black tea and selected herbal infusions (Bhebhe et al., Citation2015), which reported that extracts prepared by the combination of organic solvents (methanol, ethanol and acetone) with water had higher antioxidant properties than water or absolute organic solvents.
Figure 5. Effect of solvents on recovery of antioxidant properties from macadamia skin using various antioxidant assays such as ABTS (A), DPPH (B), FRAP (C) and CUPRAC (D)
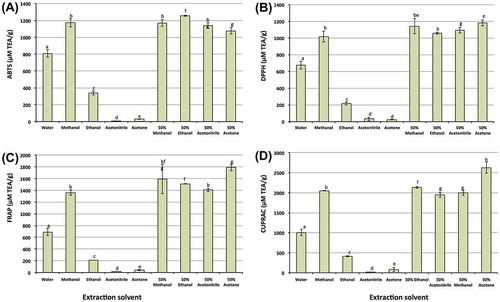
The variation of antioxidant properties can be explained by the phenolic compounds, which were dissolved differently due to different polarities of the solvents used. As shown in Table , phenolic compounds and their secondary metabolites all had a close correlation with antioxidant properties of the extracts (R 2 > 0.9). These findings revealed that phenolic compounds and their second metabolites are major antioxidants in macadamia skin. Extracts prepared from the macadamia skin using a combination between organic solvents and water possessed more potent antioxidant properties than aqueous or absolute organic solvent extracts, further suggesting that 50% ethanol or 50% acetone should be used for the recovery of phenolic compounds and antioxidant capacity from macadamia skin.
Table 2. Correlation between bioactive compounds and antioxidant properties
4. Conclusion
The results of this study revealed that macadamia skin is an abundant waste generated by the macadamia industry. Approximately 40% of the macadamia nut weight is the skin waste, indicating that macadamia skin is a great starting source for the recovery of by-products. The results also showed that the type of solvents had a significant impact on the recovery yields of phenolic compounds, flavonoids, proanthocyanidins and antioxidant properties of the macadamia skin. A combination of organic solvents with water gave significantly higher recovery yields than those of water or absolute organic solvents. Fifty per cent acetone is recommended for the extraction of phenolic compounds and flavonoids and 50% ethanol is suggested for extraction of proanthocyanidins from macadamia skin.
Additional information
Funding
Notes on contributors
Adriana Dailey
Adriana Dailey graduated BSc in Food Science and Human Nutrition from the University of Newcastle, Australia in 2014. She is presently an honours student at the University of Newcastle. She is actively working on the project: “Recovery of phenolic compounds and lignin from macadamia waste for utilisation as the food ingredients”.
Quan V. Vuong
Quan V. Vuong is a lecturer in Food Science and Human Nutrition at the University of Newcastle Australia. He has experience and is interested in identification, extraction and purification of bioactive compounds from various natural sources and utilisation of waste from food processing to add value to the food industry.
References
- Addai, Z. R. , Abdullah, A. , & Mutalib, S. A. (2013). Effect of extraction solvents on the phenolic content and antioxidant properties of two papaya cultivars. Journal of Medicinal Plants Research , 7 , 3354–3359. doi:10.5897/JMPR2013.5116
- Apak, R. , Güçlü, K. , Özyürek, M. , & Karademir, S. E. (2004). Novel total antioxidant capacity index for dietary polyphenols and vitamins C and E, using their cupric ion reducing capability in the presence of neocuproine: CUPRAC method. Journal of Agricultural and Food Chemistry , 52 , 7970–7981. doi:10.1021/jf048741x
- Australian-Macadamia-Society . (2015). Global production . Retrieved September 30, 2015, from http://www.australian-macadamias.org/industry/item/601-issue-five
- Bae, J.-S. , & Su, S. (2013). Macadamia nut shell-derived carbon composites for post combustion CO2 capture. International Journal of Greenhouse Gas Control , 19 , 174–182. doi:10.1016/j.ijggc.2013.08.013
- Bettaieb Rebey, I. , Bourgou, S. , Ben Slimen Debez, I. , Jabri Karoui, I. , Hamrouni Sellami, I. , Msaada, K. , … Marzouk, B. (2012). Effects of extraction solvents and provenances on phenolic contents and antioxidant activities of cumin (Cuminum cyminum L.) seeds. Food and Bioprocess Technology , 5 , 2827–2836. doi:10.1007/s11947-011-0625-4
- Bhebhe, M. , Füller, T. N. , Chipurura, B. , & Muchuweti, M. (2015). Effect of solvent type on total phenolic content and free radical scavenging activity of black tea and herbal infusions. Food Analytical Methods . doi:10.1007/s12161-015-0270-z
- D’Archivio, M. , Filesi, C. , Di Benedetto, R. , Gargiulo, R. , Giovannini, C. , & Masella, R. (2007). Polyphenols, dietary sources and bioavailability. Annali dell’Istituto Superiore di Sanita , 43 , 348–361.
- Dhanani, T. , Shah, S. , Gajbhiye, N. A. , & Kumar, S. (2013). Effect of extraction methods on yield, phytochemical constituents and antioxidant activity of Withania somnifera . Arabian Journal of Chemistry . doi:10.1016/j.arabjc.2013.02.015
- Do, Q. D. , Angkawijaya, A. E. , Tran-Nguyen, P. L. , Huynh, L. H. , Soetaredjo, F. E. , Ismadji, S. , & Ju, Y. H. (2014). Effect of extraction solvent on total phenol content, total flavonoid content, and antioxidant activity of Limnophila aromatica . Journal of Food and Drug Analysis , 22 , 296–302. doi:10.1016/j.jfda.2013.11.001
- FDA . (2012). Guidance for industry . Retrieved September 28, 2015, from http://www.fda.gov/downloads/drugs/guidancecomplianceregulatoryinformation/guidances/ucm073395.pdf
- Kumar, S. , & Pandey, A. K. (2013). Chemistry and biological activities of flavonoids: An overview. The Scientific World Journal , 2013 , 16. doi:10.1155/2013/162750
- Li, Y. , Guo, C. , Yang, J. , Wei, J. , Xu, J. , & Cheng, S. (2006). Evaluation of antioxidant properties of pomegranate peel extract in comparison with pomegranate pulp extract. Food Chemistry , 96 , 254–260. doi:10.1016/j.foodchem.2005.02.033
- Munro, I. A. , & Garg, M. L. (2008). Nutrient composition and health beneficial effects of macadamia nuts. In C. Alasalvar & F. Shahidi (Eds.), Tree nuts: Composition, phytochemicals, and health effects (pp. 249–558). Boca Raton, FL: CRC Press.
- Pinelo, M. , Rubilar, M. , Jerez, M. , Sineiro, J. , & Núñez, M. J. (2005). Effect of solvent, temperature, and solvent-to-solid ratio on the total phenolic content and antiradical activity of extracts from different components of grape pomace. Journal of Agricultural and Food Chemistry , 53 , 2111–2117. doi:10.1021/jf0488110
- Shoji, T. (2014). Chapter 26—Chemical properties, bioavailability, and metabolomics of fruit proanthocyanidins. In R. R. Watson , V. R. Preedy , & S. Zibadi (Eds.), Polyphenols in human health and disease (pp. 339–351). San Diego, CA: Academic Press.10.1016/B978-0-12-398456-2.00026-8
- Singh, M. , Jha, A. , Kumar, A. , Hettiarachchy, N. , Rai, A. K. , & Sharma, D. (2014). Influence of the solvents on the extraction of major phenolic compounds (punicalagin, ellagic acid and gallic acid) and their antioxidant activities in pomegranate aril. Journal of Food Science and Technology , 1–8. doi:10.1007/s13197-014-1267-0
- Thaipong, K. , Boonprakob, U. , Crosby, K. , Cisneros-Zevallos, L. , & Hawkins Byrne, D. (2006). Comparison of ABTS, DPPH, FRAP, and ORAC assays for estimating antioxidant activity from guava fruit extracts. Journal of Food Composition and Analysis , 19 , 669–675. doi:10.1016/j.jfca.2006.01.003
- Vuong, Q. V. , Hirun, S. , Phillips, P. A. , Chuen, T. L. K. , Bowyer, M. C. , Goldsmith, C. D. , & Scarlett, C. J. (2014). Fruit-derived phenolic compounds and pancreatic cancer: Perspectives from Australian native fruits. Journal of Ethnopharmacology , 152 , 227–242. doi:10.1016/j.jep.2013.12.023
- Vuong, Q. V. , Hirun, S. , Roach, P. D. , Bowyer, M. C. , Phillips, P. A. , & Scarlett, C. J. (2013). Effect of extraction conditions on total phenolic compounds and antioxidant activities of Carica papaya leaf aqueous extracts. Journal of Herbal Medicine , 3 , 104–111. doi:10.1016/j.hermed.2013.04.004
- Walton, D. A. , & Wallace, H. M. (2010). Dropping macadamia nuts-in-shell reduces kernel roasting quality. Journal of the Science of Food and Agriculture , 90 , 2163–2167. doi:10.1002/jsfa.4063
- Wechsler, A. , Ramírez, M. , Crosky, A. , Zaharia, M. , Jones, H. , Ballerini, A. , & Sahajwalla, V. (2011). Physical properties of furniture panels from macadamia shells . Paper presented at the ICCM International Conferences on Composite Materials, Jeju Island.
- Ye, F. , Liang, Q. , Li, H. , & Zhao, G. (2015). Solvent effects on phenolic content, composition, and antioxidant activity of extracts from florets of sunflower (Helianthus annuus L.). Industrial Crops and Products , 76 , 574–581. doi:10.1016/j.indcrop.2015.07.063
- Zhishen, J. , Mengcheng, T. , & Jianming, W. (1999). The determination of flavonoid contents in mulberry and their scavenging effects on superoxide radicals. Food Chemistry , 64 , 555–559. doi:10.1016/s0308-8146(98)00102-2