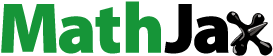
Abstract
The decomposer soil community maintains the arable soil ecosystem for the nutrient turnover. Here, we studied the dynamics of the soil fungal populations in a typical Phaeozem luvico in Argentina and related it to the management practices. Soil samples (at 0–10 cm depth) were collected from a field cultivated with wheat, at different sampling times: at post-harvest, before sowing, and at tillering. The relative abundance of individuals in the population on Nash Snyder and Oxgall agar media was assessed as colony forming units (CFU). The fungal population was classified by numerical taxonomy at the different sampling times. The highest values of CFU g−1 of soil were found at post-harvest under reduced tillage and differed significantly from those at conventional tillage. The genera Trichoderma, Fusarium, Aspergillus, Penicillium, and Fusarium oxysporum were present in the largest number of samples and discriminated the fungal community between times. This discrimination could be related to alterations in the availability of carbon sources during stubble degradation at post-harvest and before sowing and were of lesser importance at tillering.
Public Interest Statement
Soil quality and health has been defined as its ability to function as a living system, maintaining productivity and environmental quality, and to promote plant, animal, and human health. The soil management and other disturbances are related to changes in fungal diversity as part of soil microbial community. Observe modifications in soil mycoflora could precede any detectable changes in physical and chemical properties and thus provide an early signal of soil degradation. Fungal diversity seems to be a good indicator of ecosystem disturbance and functioning. Quantify the aerobic fungal population results in a sensitive indicator of the changes caused by stubble degradation in reduced and conventional tillages. Related to this, growers that know the predominance of the epidemic diseases in the area, may be given assistance applying the results obtained from this research by deploying the methodology about the appropriated tillage system to reduce the fungal diseases in natural way.
Competing Interests
The authors declare no competing interest.
1. Introduction
Agricultural intensification results in the modification of the quality of soil, thus affecting its productivity. Management practices impact significantly on the composition of the soil biota (Acosta Martínez, Mikha, & Vigil, Citation2007; Bastian, Bouziri, Nicolardot, & Ranjard, Citation2009; Gomez, Aulicino, Mónaco, Kripelz, & Cordo, Citation2015), and changes in the microbial community composition or activity could have immediate or long-term effects on the functioning of the ecosystem (Perry, Amaranthus, Borchers, Borchers, & Brainerd, Citation1989).
The decomposer soil community consists of a wide range of bacteria, fungi, protists, and invertebrates. Saprophytic fungi are essential for the maintenance of the arable soil ecosystem for the purpose of the nutrient turnover (Kalbitz, Solinger, Park, & Michalzik, Citation2000). In most cultivated well-aerated soils, 70% of the soil biomass carbon is represented by fungi. Several fungal groups in the soil act to decompose natural substrates. Cellulolytic fungi carry out crucial enzymatic activity in the degradation of plant residue substrate and can potentially suppress soil-borne diseases, as many soil-borne pathogens act with biocontrol mechanisms (antibiosis, hyperparasitism, nutrient competition, and induced systemic resistant (Cordo et al., Citation2007; Deacon et al., Citation2006).
Excessive tilling is currently producing a marked deterioration of the soil structure and a progressive acidification of the soil. Mbuthia et al. (Citation2015) observed a marked reduction of the composition of the soil organic matter and the diversity and size of the soil microbial communities in soils under conventional tillage (CT). Gupta, Roper, Kirkegaard, and Angus (Citation1994) established that stubble burning and excessive tillage cause important losses of soil organic matter in many parts of the world.
The nitrogen from crop residues is not directly available for plant growth. Nitrogen mineralization is a process that enables the growth of plants and microbes, through organically bound recycled nitrogen (Lupwayi et al., Citation2006; Van Den Bossche, De Bolle, De Neve, & Hofman, Citation2009).
Luque, Pioli, Bonel, and Alvarez (Citation2005) established that soil samples free of crop residues for recent mineralization taken at 0–5 cm depths have a much higher population of strictly aerobic cellulolytic fungi than those taken at 5–10 cm depths. This is because of the abundance of stubble on the surface and the influence of oxygen availability, which decreases with soil depth.
Here, we hypothesized that agricultural practices such as cropping, tillage, and fertilization affect the size, composition, and distribution of the soil fungal communities, and that the effects of these practices vary along time. Thus, the aim of the present study was to characterize the soil fungal populations adjacent to straw wheat residues on a Phaeozem luvico soil of Argentina and relate their dynamics to the tillage practices, the cropping conditions and the fertilization level.
2. Material and methods
2.1. Field experiment
The field experiment was carried out at the Agricultural Experimental Station Julio J. Hirschhorn, Facultad de Ciencias Agrarias y Forestales, Universidad Nacional de La Plata, Province of Buenos Aires (34°52′ S, 57°58′ W), Argentina. The soil was a Phaeozem luvico with a silt loam texture and slight internal drainage deficiency (Vargas Gil, Citation1990). The wheat cultivar Buck Pingo was sown on 20 July 2003 and 23 July 2004 with a Deutz seed drill, at a density of 300 plants per m2. The previous crops before this experiment were: soybean in 1993, corn in 1994, and wheat from 1995 to 2004. Wheat was managed under two tillage practices: reduced tillage (RT) and CT. CT consisted in plowing twice and harrowing twice to a depth of 0.15 m, 9 and 20 days before sowing, respectively, whereas RT consisted of one tillage pass with a chisel tine to a depth of 0.30 m, 30 days before sowing. Stubble was removed from the field plot, chopped, and organized in the litterbags (220 g for each litterbag) on the surface of the straw lying on the soil for RT and buried at 0.15 m depth for CT.
The study field consisted of a large soil extension (7,586.70 m2) that included a wheat-cropped area (CA) and a natural grassland area (NoCA), each organized in three replicates of two tillage treatments (RT and CT) and two doses of fertilization (0 and 160 kg of N ha−1, N0 and N160, respectively). Figure shows the spatial arrangement of the study field. The arrow indicates the places where the soil samples were taken from.
Figure 1. Schedule of the field experiment. Cropped conditions: CA: wheat-cropped area; NoCA: Natural grassland area. Cropping system: RT: Reduced tillage, CT: Conventional tillage. Doses of fertilization: N0: without fertilization; N160: 160 kg of Nitrogen ha−1.
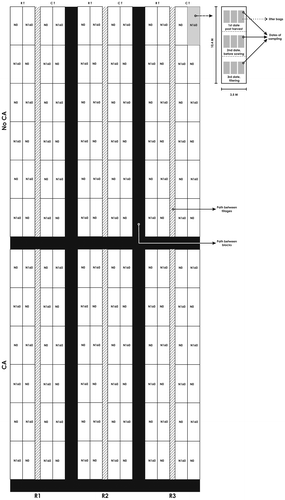
The field plots were 7 × 104 m2 and the experimental subplots were 3.50 × 10.40 m2. The tillage treatments were separated by wheat plots which were used as borders and each block (replications) was separated by a 3.5-m path of bare soil.
The soil of the field plots was sampled at three different stages of wheat development (sampling date): at post-harvest (December 2003–January 2004), before sowing (April–May 2004), and at tillering (second node enlarged; August–September 2004).
2.2. Meteorological data
Meteorological data (air and soil temperature, rainfall, and relative humidity) were measured by an automatic weather station placed 200 m from the experimental field. Data are shown in Table and were taken for the three experimental periods: post-harvest (May 2003–December 2003), before sowing (January 2004–May 2004), and at tillering (June 2004–October 2004).
Table 1. Meteorological data recorded at three experimental periods
2.3. Soil chemical properties
The soil chemical properties are shown in Table . The data were analyzed in the Laboratorio de Análisis de Suelos y Aguas from the Facultad de Ciencias Agrarias y Forestales, Universidad Nacional de La Plata, Buenos Aires Province, Argentina. The pH was determined with a pH meter (Orion research digital ionalyzer 501), in a suspension of soil and CaCl2 (1:2.5 w/w); the total organic carbon was determined by the Walkley and Black method and total nitrogen was determined by micro-Kjeldahl determination.
Table 2. Soil chemical properties recorded in the cultivated area (CA) and the natural grassland area (NoCA)
2.4. Soil fungal analysis
In spring 2003, an experiment with a double purpose was carried out: (1) to know the time taken by the wheat residues to be totally mineralized under different tillage practices and (2) to quantify the associated fungal population, in order to improve the technique of disease management. This last point was done to analyze the cellulolytic fungal population related to its decomposition. To this end, leaf and stem residues of wheat were placed in litterbags (20 × 20 cm) made of fiberglass nylon material (1.8 mm mesh). The litterbags were randomly placed under RT or CT in the CA and NoCA (Figure ). The litterbags were placed either horizontally on the surface of the straw lying on the soil in RT or buried at an acute angle in CT (maximum depth of 15 cm) (Gomez, Pioli, & Conti, Citation2007). Sampling of the soil adjacent to residues began in December 2003, 30 days after the placement of the mentioned litterbags.
On each sampling date, 10 soil cores (15 × 2 cm in diameter) for each treatment were taken from the site adjacent to each litterbag. They were mixed in a combining sample of 500 g of soil, and 30 g of each were taken to obtain a reduced number of replicates (three replicates on agar plates) before analysis. The distance between the soil sampling places for each sampling date was 3.5 m (Figure ). The purpose was that the soil cores provided a background fungal population for each plot. Following Silvestro et al. (Citation2013) and Luque et al. (Citation2005), the dilution plate technique was used and the 0–10 cm sampling depth was chosen because the surface horizons of a profile tend to have the highest levels of micro-organisms. The samples were air-dried at room temperature (22 C) and ground to pass a 2-mm sieve. Soil samples (5 g) were added to 50 mL of sterilized distilled water and shaken for 15 min. The original suspension (5 mL) was diluted in 45 mL of sterilized distilled water. This process was repeated three times to obtain 10-fold dilution series. Fungal colony forming units (CFU g−1 dry soil) were counted on two different culture media spread on plates: Nash–Snyder (N–S) medium, used because it is a selective medium for Fusarium spp. growth (Wolcan, Lori, & Perello, Citation1993), and Oxgall agar (Ox-A) medium, chosen because it produces a more general fungal growth (Dal Bello, Citation1982). Each soil suspension was spread in triplicate series. Plates were incubated at 24 C under light/dark cycles for 5–7 days before counting. After quantification, isolates of the original specimens were transferred to potato dextrose agar with chloramphenicol (300 mg L−1) slant tubes. The fungal isolates were identified to genus level and in some cases species level, using standard keys of morphological features (Domesch, Gams, & Anderson, Citation2007; Ellis, Citation1971, Citation1976; Leslie & Summerell, Citation2006; Nelson, Tousson, & Marasas, Citation1983). Only plates containing 10–100 CFU g−1 of soil were used for counting and the results were expressed as CFU g−1 of dry soil. The relative abundance of the most frequent fungal genera was determined by calculating the proportion of each genus in relation to the total number of isolates (Elmholt, Citation1996).
2.5. Statistical analysis
The number of CFU g−1 of soil was analyzed for each culture medium. First, a mixed model (McIntosh, Citation1983) was applied to the factorial analysis of the treatments across time, where sampling time and treatments were the random and fixed factors, respectively. Treatments were a combination of tillage system, fertilization level, and cropping condition. When the treatments × sampling time interactions were significant, the multiplicative effects were explored using a factorial analysis. Tukey’s test (p < 0.05) was used to compare treatments (Sockal & Rohlf, Citation1981).
For each sampling time and culture medium used to isolate the fungal genera and species, a three-way ANOVA was performed to assess differences between cropping conditions, tillage systems, and fertilization level followed by Tukey’s test (p < 0.05) to compare treatments (Sockal & Rohlf, Citation1981). To facilitate comparison between tillage systems and sampling dates, the proportional or percentage abundance of each genus or species was calculated. This simple method, called Wittaker plots (Whittaker, Citation1965), means that the abundance of all genera and species together is designed as 1.0 or 100% and that the relative abundance of each genus and species is given as a proportion or percentage of the total.
2.6. Multivariate analysis
CFU was used to classify samples by numerical taxonomy. A basic matrix with n rows (treatment: combination of fertilization, tillage, and cropped areas) and t-columns (fungal species) was built for each sampling time and culture medium, separately. Simple correspondence analysis was performed to investigate the data structure. Chi-square distance showed the proximity for both fungal species and treatments. A Biplot showed the grouping among each set of variables and the relationship between them (Sneath & Sokal, Citation1973). Numerical taxonomy was performed using NTSYS-pc 2.0 (Rohlf, Citation1998).
3. Results
No differences were found between laboratory techniques when an ANOVA was separately applied for each sampling time (ANOVA not shown). The combined mixed model for CFU g−1 of soil (Table ) revealed significant differences between sampling times and sampling times × treatments interaction, at 0.05 and 0.01 for N–S and Ox-A media, respectively. The decomposition of the interaction sum of square proved that only the sampling times × tillage practice interaction was significant at 0.05 and 0.01 for N–S and Ox-A, respectively (ANOVA not shown). The sum of square decomposition for each significant source of variation demonstrated that only the sampling times × tillage practice interaction explained 47.69 and 59.54% of the model, while the sampling time effect explained only 19.04 and 18.94%, for N–S and Ox-A, respectively. Tukey’s test analysis of tillage practices across the three sampling dates, for N–S and Ox-A, is shown in Figures and , respectively. The highest values of CFU g−1 of soil were found for the samples collected at post-harvest from soils under RT and differed significantly from those at CT throughout the experiment for Ox-A. However, for N–S, the values of fungal CFU g−1 were not significantly higher before sowing and at tillering than at post-harvest under CT (Figure ). These results demonstrate a contrasting tendency, for both culture media (Figures and ). The CFU values of samples coming from RT decreased along the sampling times, whereas those from CT increased slightly.
Table 3. Mixed model to analyze the CFU g−1 of soil for two culture media: Nash–Snyder (N–S) and Oxgall agar (Ox-A). F values for treatments, sampling time, and treatments × sampling time interactions. Treatments are a combination of: Tillage System (RT and CT) ×, Nitrogen Fertilization (N0 and N160) × Cropping Condition (CA and NoCA)
Figure 2(a). Mean values of CFU for the “treatment × sampling time” interaction (TR × ST) for the Nash Snyder (N-S) medium. Treatments were: tillage system with reduced tillage (RT) and conventional tillage (CT); Tukey’s test at 5% probability for the T × ST interaction values for the N–S medium. Columns with the same letter do not differ significantly (p ≤ 0.05).
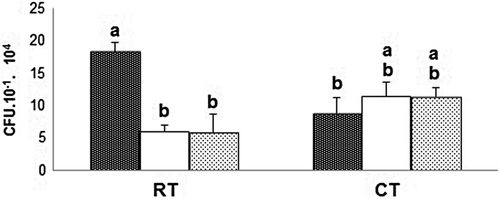
Figure 2(b). Mean values of CFU for the treatment × sampling time interaction (TR × ST) for the Oxgall agar (Ox-A) medium. Treatments were: tillage system with reduced tillage (RT) and conventional tillage (CT); Tukey’s test at 5% probability for the T × ST interaction values for the Ox-A medium. Columns with the same letter do not differ significantly (p ≤ 0.05).
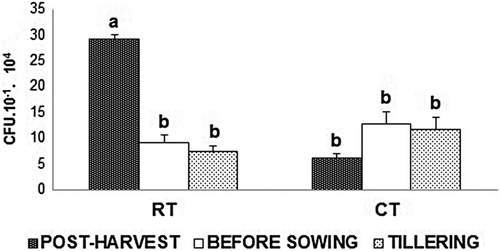
The results of the factorial analysis for each sampling time using CFU g−1 of soil showed that, in both culture media, the tillage system was highly significant at post-harvest. In addition, before sowing and at tillering, significant differences at 5% were found when the CFU values were measured in N–S (Table ). The CFU g−1 of soil counted on Ox-A showed a significant tillage practice × fertilization level interaction only at post-harvest. Tukey’s test demonstrated that the samples found under RT and N160 had values of CFU significantly higher than those under CT and N160 (p < 0.01) but not significantly different from those at RT and N0. In contrast, soil samples from CT and N160 had the lowest number of propagules (Figure ).
Table 4. Factorial analysis of variance using CFU g−1 of soil for both culture media at each sampling time
Figure 3. Mean values of CFU for the Ox-A medium at post-harvest. Tukey’s test to compare the tillage system × fertilization interaction. RT-N0: reduced tillage × 0 kg nitrogen ha−1; RT-N160: reduced tillage × 160 kg nitrogen ha−1; CT-N0: conventional tillage × 0 kg nitrogen ha−1; CT-N160: conventional tillage × 160 kg nitrogen ha−1.
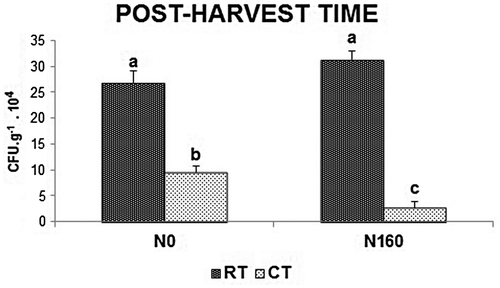
Table shows the fungal relative abundance of each genus and species on soils sampled for each tillage system and sampling time.
Table 5. Relative abundance of fungal genera and species from the soil collected adjacent to litterbag places
The multivariate analysis, for post-harvest, separated RT from CT samples, when both culture media (Figures (a) and (a)) were assayed. On the other hand, the second coordinate axis separated fertilized (N160) from unfertilized (N0) samples and the CA from the NoCA. Our results showed that in both culture media, Trichoderma spp., Fusarium oxysporum, Aspergillus spp., and Penicillium spp. had the highest CFU because they were present in the largest number of samples.
Figure 4. Correspondence analysis for the Nash–Snyder (N–S) medium. Association between treatment and fungal species at each sampling time. Treatments are: tillage system with reduced tillage (RT) and conventional tillage (CT); cropping condition with wheat-cropped area (CA) or without wheat-cropped area (No-CA); nitrogen fertilization with 0 kg nitrogen ha−1 (N0) or 160 kg nitrogen ha−1. Variability percentage for dimensions 1 and 2.
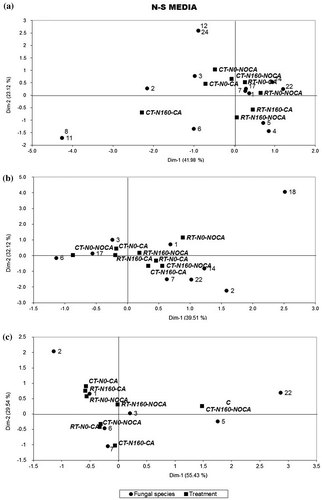
Figure 5. Correspondence analysis for the Oxgall Agar (Ox-A) medium. Association between treatment and fungal species at each sampling time. Treatments are: tillage system with reduced tillage (RT) and conventional tillage (CT); cropping condition with wheat-cropped area (CA) or without wheat-cropped area (No-CA); nitrogen fertilization with 0 kg nitrogen ha−1 (N0) or fertilized with 160 kg nitrogen ha−1. Variability percentage for dimensions 1 and 2.
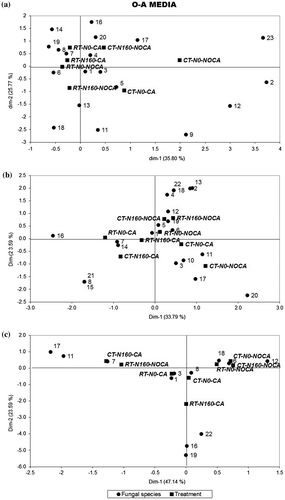
Reduced matrixes were built when the N–S medium was used. The first axis of correspondence analysis separated the CA treatment (on the left axis) from the NoCA treatments (on the right axis). At post-harvest, 14 fungal species were counted, but only 9 and 7 were detected before sowing and at tillering, respectively (Figure (a)–(c)). The Ox-A medium allowed identifying 19 fungal species at post-harvest, 21 before sowing and 12 at tillering (Figure (a)–(c), respectively).
4. Discussion
Reduced tillage, use of cover crops, and appropriate use of fertilizers are some of the conservation agricultural practices being promoted for the mitigation of soil erosion and improvement in soil and water quality and crop productivity (Hobbs, Sayre, & Gupta, Citation2008).
Nitrogen is one of the nutrients that are recycled through crop residues. Most of the nitrogen in crop residues is in organic form and not directly available for plant growth. The mineralization process allows that the organically bound nitrogen would be available for crop or microbial growth (Lupwayi et al., Citation2006).
Since 2004, in the Argentine fields, the number of tillage operations used for summer fallow and seedbed preparation has been diminished to avoid the soil degradation action (Asociación Argentina de Productores en Siembra Directa Citation1996, 1999). Holland and Coleman (Citation1987) observed that the organic matter amended in the soil induces most of the biological changes in the soil communities because of the availability of carbon sources. Bossio, Scow, Gunapala, and Graham (Citation1998) established that organic fertilization modified the microbial population structure. Bastian, Alabouvette, and Saiz-Jimenez Citation(2009) suggested that to create a healthy soil and contribute to improving crop production, a diverse and balanced population of biologically active microbial species should be maintained throughout management practices.
In this work, the soil viable fungi were counted using the dilution plate count technique, as previously done by Gomez et al. (Citation2007), Luque et al. (Citation2005), and Nesci, Barros, Castillo, and Etcheverry (Citation2006) for Argentina and by Harris, Schomberg, Banks, and Giddens (Citation1994) for the USA. We recognize that this method is imperfect because it detected only part of the total community and was subject to quantitative error. Some species would be inhibited by other fungi that produce large numbers of spores and more quickly, without being detected by the dilution method. Oxgall agar, which has a fungistatic substance, added to the basal potato dextrose agar used in this study, reduced the growth of the fast-growing species, originating the largest number of species quantified on this nutritive medium. Thus, we used this technique to analyze the dynamics of the fungal cellulolytic population in the soil. The soil dilution technique allowed us to obtain a continuous number of CFU of different genera in a short period of time. Considering that the detectable portion of the mycoflora differs over time and environments, this method was useful in this research to indicate changes in the community dynamics and composition (Beare, Coleman, Pohlad, & Wright, Citation1993; Gomez et al., Citation2007; Harris et al., Citation1994; Nesci et al., Citation2006; Pfender & Wootke, Citation1988).
In agreement with Beare et al. (Citation1993), Holland and Coleman (Citation1987) and Van Den Bossche et al. (Citation2009) about the number of propagules and the high doses of fertilization, our results indicate that for post-harvest time and in CT the low number of CFU g−1 of soil could be related to more rapid nitrogen mineralization and nitrification. We can suggest that under CT-N160 condition, the mineralized nitrogen increased by the degradation of soil residues that added to the incorporated by fertilization. Thus, the activity of the microbial biomass accelerated drastically by the bacterial predominance, decreasing the fungal CFU g−1 of our soils (Van Den Bossche et al., Citation2009).
According to Bastian et al. Citation(2009), Gomez et al. (Citation2007) and Mbuthia et al. (Citation2015), under no-till, the soil fungi localized adjacent to plant residues and responsible for nitrogen mineralization are considerably diverse. The results of this work showed that under RT the number of fungal species increased slightly during the decomposition process, compared with the increase in the number of fungal species in CT and for the same sampling time. Nevertheless, at tillering, the number of species was equal, independently of the tillage system. Alternaria alternata, Epicoccum nigrum, and Cladosporium Cladosporioides, which are regular colonizers of the phyllosphere of senescing plant tissue (Elmholt & Smedegaard-Petersen, Citation1988), were among the fungi found colonizing initial wheat residues. These primary saprophytes were grouped with other common cellulolytic and sugar-degrading fungi, including Fusarium oxysporum, Mucor racemosus f. racemosus, and Phoma sp. These species and their sexual stages (Ascomycota in general) represent the main saprophytic decomposers classically identified in different soil niches (Bastian et al., Citation2009).
Related to the significant predominance of the genera Trichoderma spp., Fusarium oxysporum, Aspergillus spp., and Penicillium spp. (Table ) in the largest amount of soils of this study, Beare et al. (Citation1993) reported similar fungal species in their work and Gomez et al. (Citation2007) established that because these species are capable of using different substrates in the soil they predominated over other fungal populations that used only a few specific substrates. The reason why other fungal genera were in a low proportion or absent in the sampling sites was that saprophytic fungi were present in a high relative density. On the other hand, in CT-N0-NoCA, Cephalosporium sp. and Alternaria triticina were relatively more abundant because these genera can survive in surface residues and slightly degraded organic matter (Gomez et al., Citation2007).
For both cultural media, the highest number of fungal species was associated with post-harvest time. Our results for post-harvest add to the conclusion of Beare et al. (Citation1993), who also found that fungal densities are strongly affected by tillage practices, with fewer CFU in buried residues from CT than in those from RT. Also, we demonstrated that for both culture media, the CFU g−1 of soil from RT decreased along the sampling times. However, the CFU g−1 of soil from CT increased slightly. This indicates that the highest variability is produced by the tillage system at post-harvest. Along the time, these differences were not so clear and special associations were found between particular species and treatment.
The results of the correspondence analysis on Oxgall agar and at three sampling times are in agreement with that found by Gomez et al. (Citation2007), who clearly distinguished the separation of no-till sites from the other sites over the relative abundance of fungal genera for three years in Entre Ríos Province, Argentina. The treatments and micro-organisms that were far away from the graphical center (0,0) had the lowest CFU g−1. These micro-organisms are considered rare species because they are present in only one or a few treatments and may show preference for some special condition. In this work, the multivariate analysis grouped the soil treatments coming from NoCA on the first component, associated with a greater diversity of fungal species in Oxgall agar at the three sampling times. This result is in agreement with that found by Acosta Martínez et al. (Citation2007), who demonstrated that the highest diversity observed in soil samples from the NoCA was due to the positive impact of surface cover, vegetation and lack of tillage of pasture on the soil, which led to higher soluble organic carbon and total nitrogen than those of the cropping system and thus to a higher soil microbial population.
The results of this work are also in accordance with those of Bastian et al. Citation(2009), who found a significant discrimination of the fungal community structure between the first and the last sampling time. The changes in the fungal community structure could be related to alterations in the availability of carbon sources occurring during degradation at post-harvest and before sowing and were of lesser importance at tillering. According to Bastian et al. Citation(2009) there is a strong selective pressure of fresh organic matter on the overall soil diversity leading to proliferation of the fungal population in the first and second sampling times.
Other factors of change in the structure of the fungal community could be expected when the period of time between sampling dates is taken into account. In this sense, Broder and Wagner established that although many of the temporal changes in fungal composition may be attributed to the succession during residue decay, some are probably due to seasonal changes in temperature and humidity, which influence the growth of some fungal species. Under the experimental conditions of this research, the changes in temperature (soil temperature) and humidity (soil relative humidity) were less pronounced in CT than in RT and the fungal communities maintained their biological equilibrium, increasing their CFU g−1 of soil both before sowing and at tillering. Our results add to the conclusions of Luque et al. (Citation2005), who affirmed that the fungal counts were higher in the top layer of the soil profile due to the abundance of stubble in the surface with important oxygen availability. In this sense, Nicolardot, Bouziri, Bastian, and Ranjard (Citation2007) and Bastian et al. Citation(2009) verified that reduced and minimum tillage provide enough crop residues and organic carbon as substrate for multiplication of soil micro-organisms.
According to Pianka (Citation1970), these fungal communities are classified as r- and K-strategists. Our interpretation is that the dynamics of the community structure observed seems to be related to changes in the availability of carbon resources occurring during degradation. Our study confirmed the ability of the fungal taxonomic group present at post-harvest to colonize residues and consume labile organic carbon in the first step of the degradation process. We also deduced that this group could then strongly be involved in the mineralization of wheat-derived carbon and CO2 release. In this first step of mineralization, fast-growing micro-organisms, commonly classified as r-strategists, are the consequence of non-limited substrate. In contrast, the fungal species present at tillering were stimulated by residue decomposition at later stages. According to Cayuela et al. (Citation2009), the supply of carbon in the form of complex and insoluble compounds induces parallel growth of K-strategists, which grow slowly and are expected to allocate more energy to the production of extracellular enzymes. Our results suggest that, in the context of a unified r- and K-selection continuum, some fungal species (such as Glyocephalis sp., Chaetomium cochlioides, Aspergillus spp., Nigrospora spp., and Penicillium spp.) can be considered as K-strategists which are adapted to survive with a slower growth rate when resources are limited or consist of more complex recalcitrant organic matter. Thus, our hypothesis is that the initial response to rapid mineralization of the more labile plant fraction was followed by enzyme induction before mineralization of more resistant fractions.
5. Conclusion
• | This study confirmed that the tillage system was the most discriminating factor to describe the fungal community of the soil associated with the degradation of wheat straw residues. | ||||
• | The dynamics of fungal populations over time had a pattern of differential behavior mainly in relation to tillage. The results demonstrate that the fungal CFU values of soil samples from RT decreased along the sampling times and that those from CT increased slightly. This dynamic was also influenced by nitrogen fertilization exclusively in soils with CT. | ||||
• | These differential patterns would be determined by a differential reduction of the organic matter through each sampling time, resulting in interspecific competition for the same resource and in a change in the succession of species in quantity and quality. | ||||
• | Our results suggest that it is important to know the influence of tillage practices on the size and composition of residue-associated fungal communities to develop residue management strategies. These strategies could regulate their decomposition rates and control the proliferation of phytopathogenic fungi present on the residues. |
Acknowledgments
We thank E. Balonga for the graphic design assistance and Ing. Agronomo Martin Pardi for technical assistance in the field experiment.
Additional information
Funding
Notes on contributors
Cristina A. Cordo
The Facultad de Ciencias Agrarias y Forestales de La Plata, Buenos Aires, Argentina, with the CIDEFI Centre, has been recognized for its outstanding contribution in several aspects related to Plant Pathology, Biocontrol, and Soil Microbiology. The Biologist Romina Gómez is a specialist in fungal soil population related with different tillage practices and cropping conditions. The co-authors are grouped to contribute with some aspects of the research related with the microbial soil community. Example of this is Mónica Aulicino who has been responsible to make the Statistical analysis of the collected data, or Cristina Cordo and Cecilia Mónaco who are specialists in Micology and taxonomy. On the other hand, the special contribution of Natalia Kripelz made possible the cultural studies and maintenance of the fungal collection. Cristina A Cordo is a member of the research group.
References
- Acosta Martínez, V., Mikha, M., & Vigil, M. (2007). Microbial communities and enzyme activities in soils under alternative crop rotations compared to wheat-fallow for the Central Great Plains. Applied Soil Ecology, 37, 41–52.10.1016/j.apsoil.2007.03.009
- Asociación Argentina de Productores en Siembra Directa. (1996). Gacetilla informativa (p. 12). Rosario: Author.
- Asociación Argentina de Productores en Siembra Directa. (1999). Gacetilla informativa (p. 3). Rosario: Author.
- Bastian, F., Alabouvette, C., & Saiz-Jimenez, C. (2009). Bacteria and free-living amoeba in the Lascaux cave. Research in Microbiology, 160, 38–40.10.1016/j.resmic.2008.10.001
- Bastian, F., Bouziri, L., Nicolardot, B., Ranjard, L. (2009). Impact of wheat straw decomposition on successional patterns of soil microbial community structure. Soil Biology and Biochemistry, 41, 262–275.10.1016/j.soilbio.2008.10.024
- Beare, M., Coleman, D. C., Pohlad, B. R., & Wright, D. (1993). Residue placement and fungicide effects on fungal communities in conventional and no-tillage soils. Soil Science Society of America Journal, 57, 392–399.10.2136/sssaj1993.03615995005700020018x
- Bossio, D., Scow, K., Gunapala, N., & Graham, K. (1998). Determinants of soil microbial communities: Effects of agricultural management, season, and soil type on phospholipid fatty acid profiles. Microbial Ecology, 36, 1–12.10.1007/s002489900087
- Cayuela, L., Golicher, D. J., Newton, A. C., Kolb, M., de Alburquerque, F. S., & Arets, E. (2009). Species distribution modeling in the tropics: Problems, potentialities, and the role of biological data for effective species conservation. Tropical. Conservation Science, 2, 319–352.
- Cordo, C., Monaco, C., Segarra, C., Simon, M., Mansilla, A., Perelló, A., … Conde, R. (2007). Trichoderma spp. as elicitors of wheat plant defense responses against Septoria tritici. Biocontrol Science and Technology, 17, 687–698.10.1080/09583150701527094
- Dal Bello, G. (1982). Selección de dos medios de cultivo adaptados al aislamiento de hongos del suelo. In 2° Congreso Latinoamericano de Fitopatología (pp. 42–52). Buenos Aires.
- Deacon, L., Pryce-Miller, J., Frankland, B., Baingbridge, B., Moore, P., & Robinson, C. (2006). Diversity and function of decomposer fungi from a glassland soil. Soil Biology and Biochemistry, 38, 7–20.
- Domesch, K., Gams, W., & Anderson, T. (2007). Compendium of soil fungi (2nd ed.). Eching: IHW Verlag.
- Ellis, M. B. (1971). Dematiaceous hyphomycetes (p. 608). Surrey: Commonwealth Mycological Institute.
- Ellis, M. B. (1976). More dematiaceous hyphomycetes (p. 507). Surrey: Commonwealth Mycological Institute.
- Elmholt, S. (1996). Microbial activity, fungal abundance, and distribution of Penicillium and Fusarium as bioindicators of a temporal development of organically cultivated soils. Biological Agriculture & Horticulture, 13, 123–140.10.1080/01448765.1996.9754772
- Elmholt, S., & Smedegaard-Petersen, V. (1988). Side-effects of field applications of ‘propiconazol’ and ‘captafol’ on the composition of non-target soil fungi in spring barley. Journal of Phytopathology, 123, 79–88.10.1111/j.1439-0434.1988.tb01039.x
- Gomez, E., Pioli, R., & Conti, M. (2007). Fungal abundance and distribution as influenced by clearing and land use in a vertic soil in Argentina. Biology and Fertility of Soils, 43, 373–377.
- Gomez, R. P., Aulicino, M. B., Mónaco, C. I., Kripelz, N. I., & Cordo, C. A. (2015). Impact of different cropping conditions and tillage practices on the soil fungal abundance of a Phaeozem luvico. Spanish Journal of Agricultural Research, 13, e1102–1113.10.5424/sjar/2015132-6556
- Gupta, V., Roper, M., Kirkegaard, J., & Angus, J. (1994). Changes in microbial biomass and organic matter levels during the first year of modified tillage and stubble management practices on a red earth. Australian Journal of Soil Research, 32, 1339–1354.10.1071/SR9941339
- Harris, P., Schomberg, H., Banks, P., & Giddens, J. (1994). Burning, tillage and herbicide effects on the soil microflora in wheat-soybean double crop system. Soil Biological and Biochemistry, 27, 153–156.
- Hobbs, P. R., Sayre, K., & Gupta, R. (2008). The role of conservation agriculture in sustainable agriculture. Philosophical Transactions of the Royal Society B: Biological Sciences, 363, 543–555. doi:10.1098/rstb.2007.2169
- Holland, E., & Coleman, D. (1987). Litter placement effects on microbial and organic matter dynamics in an agroecosystem. Ecology, 68, 425–433.10.2307/1939274
- Kalbitz, K., Solinger, S., Park, J., & Michalzik, B. (2000). Controls on the dynamics of dissolved organic matter in soils: A review. Soil Science, 165, 277–304.10.1097/00010694-200004000-00001
- Leslie, J., & Summerell, B. (2006). The Fusarium laboratory manual (1st ed., p. 388). Oxford: Blackwell.10.1002/9780470278376
- Lupwayi, N. Z., Clayton, G. W., O′Donovan, J. T., Harker, K. N., Turkington, T. K., & Soon, Y. K. (2006). Nitrogen release during decomposition of crop residues under conventional and zero tillage. Canadian Journal of Soil Science, 86, 11–19.10.4141/S05-015
- Luque, A., Pioli, R., Bonel, B., & Alvarez, D. (2005). Cellulolytic fungi populations in stubble and soil as affected by agricultural management practices. Biological Agriculture & Horticulture, 23, 121–142.10.1080/01448765.2005.9755316
- Mbuthia, L. W., Acosta-Martínez, V., DeBruyn, J., & Schaeffer, Sean, Tyler, D., Odoi, E., … Eash, N. (2015). Long term tillage, cover crop, and fertilization effects on microbial community structure, activity: Implications for soil quality. Soil Biology and Biochemistry, 89, 24–34.10.1016/j.soilbio.2015.06.016
- McIntosh, M. S. (1983). Analysis of combined experiments1. Agronomy Journal, 75, 153–155.10.2134/agronj1983.00021962007500010041x
- Nelson, P., Tousson, T., & Marasas, W. (1983). Fusarium species: An illustrated manual for identification (p. 193). University Park, PA: Penn State University Press.
- Nesci, A., Barros, G., Castillo, C., & Etcheverry, M. (2006). Soil fungal population in preharvest maize ecosystem in different tillage practices in Argentina. Soil & Tillage Research, 91, 143–149.
- Nicolardot, B., Bouziri, L., Bastian, F., & Ranjard, L. (2007). A microcosm experiment to evaluate the influence of location and quality of plant residues on residue decomposition and genetic structure of soil microbial communities. Soil Biology and Biochemistry, 39, 1631–1644.10.1016/j.soilbio.2007.01.012
- Perry, D. A., Amaranthus, M., Borchers, J., Borchers, S., & Brainerd, R. (1989). Bootstrapping in ecosystems. BioScience, 39, 230–237.10.2307/1311159
- Pfender, W., & Wootke, S. (1988). Microbial communities of Pyrenophora-infested wheat straw as examined by multivariate analysis. Microbial Ecology, 15, 95–113.10.1007/BF02012954
- Pianka, E. (1970). On r- and K-selection. The American Naturalist, 104, 592–597.10.1086/282697
- Rohlf, M. (1998). NTSYS–pc. Numerical taxonomy and multivariate analysis system [Version 2.0]. Stony Brook, NY: Department of Ecology and Evolution, State University of New York.
- Silvestro, L., Stenglein, S., Forjan, H., Dinolfo, M., Arambarri, A., Manso, L., & Moreno, M. (2013). Occurrence and distribution of soil Fusarium species under wheat crop in zero tillage. Spanish Journal of Agricultural Research, 11, 72–79.10.5424/sjar/2013111-3081
- Sneath, P. H. A., & Sokal, R. R. (1973). Numerical taxonomy: The principles and practice of numerical classification (573 p.). San Francisco, CA: W.H. Freeman.
- Sockal, R., & Rohlf, F. (1981). Biometry: The principles and practices of statistics in biological research (2nd ed.). San Francisco, CA: W.H. Freeman.
- Van Den Bossche, A., De Bolle, S., De Neve, S., & Hofman, G. (2009). Effect of tillage intensity on N mineralization of different crop residues in a temperate climate. Soil & Tillage Research, 103, 316–324.
- Vargas Gil, J. (1990). Atlas de suelos de la República Argentina [Soils Atlas of República Argentina]. Buenos Aires: INTA.
- Whittaker, R. (1965). Dominance and diversity in land plant communities: Numerical relations of species express the importance of competition in community function and evolution. Science, 147, 250–260.10.1126/science.147.3655.250
- Wolcan, S., Lori, G., & Perello, A. (1993). Poblaciones de Fusarium en suelos de la provincia de Buenos Aires (Republica Argentina) [Population of Fusarium sp. in soils of Buenos Aires Province (Argentina)]. Fitopatologia Brasileira, 18, 399–403.