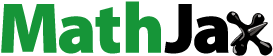
Abstract
This study investigated the impact of three adjacent land use systems [land under arable cropping (ARL), cattle grazing fallow (GFL) and Jatropha curcas L. Orchard (JCL)] on selected soil physical quality indicators in a Northern Nigeria Savanna Alfisol. GFL had significantly higher organic carbon content (26.2–32.1% higher) and bulk density (4.5–9.2% higher) than JCL and ARL respectively. Higher bulk density (ρb) in GFL aided by trampling induced compaction resulted in its high relative field capacity (RFC), permanent wilting point (PWP) and micro-pore spaces (PMIC). Continuous tillage in ARL created loose soil in the plough layer (<20 cm) which turn out to its low bulk density (ρb) and high plant-available water capacity (PAWC), total pore spaces (f) and macro-pore spaces (PMAC) over JCL and GFL. Total nitrogen content, dry stability of large macro-aggregate fractions [5–2 mm, (LMag)] and mean weight diameter (MWD) were higher in JCL than GFL and ARL to about 47.2–60.6, 12.5–68.8 and 8.57–44.76%, respectively. This showed some possibilities of JCL in improving the nitrogen content and stability of soil. Yet, long term studies on the impact of J. curcas L. on the soil quality are needed in order to evaluate if such possibilities are sustainable or not.
Public Interest Statement
Jatropha curcas L. (physic nut) is a large shrub belonging to the genus Euphorbiaceae, producing oil rich containing seeds. The oil from the seed is an environmentally benign renewable source of non-conventional energy and a promising substitute for diesel and other fuels. Furthermore, J. curcas L. was acclaimed to be a drought tolerant shrub with potential for reclaiming land ill-suited for enhanced crop production. To evaluate such claim, this study investigated some physical quality indicators of soil under J. curcas L. plantation in comparison with two adjacent land use systems. The study found an improvement in the structural stability and nitrogen content of soil under J. curcas L. plantation. This gives a limelight that the claim for land reclamation potential touted on J. curcas L. could be scientifically justified.
Competing Interests
The authors declare no competing interest.
1. Introduction
In a quest to respond to the demand of ever-increasing population, agricultural intensification has been the only feasible option. Across the globe, agricultural intensification with inappropriate land use has culminated in significant soil degradation. Despite the scarcity of recent and reliable data on the severity of soil degradation in Africa, Batjes (Citation2001) reports that degraded soils in Africa amount to about 494 million hectares. It has been also estimated that about 65% of agricultural land in sub-Saharan Africa (SSA) is degraded because of water and soil erosion, chemical and physical degradation (Scherr, Citation1999). The soils of the Northern Nigerian Savanna are predominantly Alfisols, Inceptisols and Ultisols, the latter especially characterized by low activity clays. Most of the soils have a small amount of organic matter content and high sand proportion in the surface layers which make them physically fragile and susceptible to degradation (Salako, Hauser, Babalola, & Tian, Citation2001). On top of this, intensive agriculture through continuous and at the same time inappropriate cropping systems and poor soil management as imposed by demographic pressures has also resulted in serious degradation of the fragile soils and an ensuing poor crop performance. The challenge now is to learn how to manage these soils for long-term productivity and improve environmental integrity.
The physical quality of an agricultural soil has a correlation with soil-strength and fluid transmission and storage characteristics in the crop root zone (Reynolds, Bowman, Drury, & Tan, Citation2002; Topp, Reynolds, Cook, Kirby, & Carter, Citation1997). An agricultural soil with good physical quality maintains good structure, holds crop upright, resists erosion and compaction, permits the correct proportion of water, allows unrestricted root growth, dissolves nutrients and air for both maximum crop performance and minimum environmental degradation (Reynolds et al., Citation2002; Topp et al., Citation1997). Soil physical quality affects chemical and biological processes in the soil, and therefore plays a crucial role in the indicators of soil quality (Dexter, Citation2004). In order to design sustainable management practices for agricultural production and soil conservation, the response of soil physical properties on common land use practices must be clearly understood.
Jatropha curcas L. (hereafter referred to as Jatropha) is a multipurpose perennial shrub belonging to the family Euphorbiaceae (Jongschaap, Corre, Bindraban, & Brandenburg, Citation2007) which produces seeds rich in oil quality that is easily converted into biodiesel of international standard (Azam, Waris, & Nahar, Citation2005). Furthermore, Jatropha was acclaimed to be a hardy shrub that can grow in marginal land (or land ill-suited for enhanced production) with potentials for reclaiming degraded soil by checking erosion. In theory, Jatropha senescence and shed leaf form mulch around the plant base, thus increasing organic matter and thus promoting soil fertility and quality (Singh, Singh, Mishara, & Bhatia, Citation2010). The soil reclamation potential touted on Jatropha was built on scanty evidence, calling for systematic evaluation of the claim. Therefore, this research was performed to compare the influence of Jatropha cultivation and other conventional land use practices on the physical quality of Northern Nigeria Savanna Alfisol.
2. Materials and methods
2.1. Description of the study area
The research was conducted at the experimental farm of the Institute for Agricultural Research (IAR), Sabon Gari LGA of Kaduna State, Nigeria located at 11°11′19.3″N and 007°37′02.16″E, and 686 m above the sea level (Figure ). The area is situated in the Northern Guinea Savanna ecology with a mono-modal rainfall pattern and a long term mean annual rainfall of about 1,011 ± 161 mm concentrated entirely in five months (May/June–September/October) and mean daily temperature of 24°C (Oluwasemire & Alabi, Citation2004). The soil type is leached tropical ferruginous soil classified as Typic Haplustalf according to USDA soil taxonomy (Ogunwole, Babalola, Oyinlola, & Raji, Citation2001) which had developed on deeply weathered pre-Cambrian Basement Complex but overlaid by Aeolian drift of varying thickness.
2.2. Soil sampling and preparation
The study consists of three adjacent land use systems, namely: (i) >3 years land under arable cropping (Hereafter referred to as ARL) tilled by disc plough and was cropped with maize in 2008 and 2009, and cowpea in the 2010 rainy seasons respectively. (ii) >3 years cattle grazing fallow (Hereafter referred to as GFL) dominated by the following grass and shrub species; Cyperus rotundus L., Andropogon gayanus L., Loudetia annua, Piliostigma reticulatum, Daniellia oliveri and Guera senegalensis. (iii) >3 years Jatropha Orchard (Hereafter referred to as JCL). Loose and undisturbed core samples (5 cm height, 5 cm diameter) were collected at four depths 0–5, 5–10, 10–15 and 15–20 cm in May 2011. A stratified random sampling technique (Peterson & Calvin, Citation1986) that divides a large field into pseudo-replicates or subdivisions (in this case, five pseudo-replications) was adopted. Four random loose samples and one undisturbed core sample were collected from each replicate at each depth. Thereafter, the four randomly collected loose samples were bulked to give one composite sample per each depth and sub-division, making 60 loose composite and undisturbed core samples [Three (land use systems) *Four (soil depths) *Five (replications)]. In the laboratory, the composite samples were then divided into two halves and air-dried; one half was passed through 5 mm sieve and the other half passed through a 2 mm sieve by gently breaking apart the soil.
2.3. Laboratory analysis
The air-dried 2 mm sieved sample was used for total nitrogen (TN) determination using Kjeldahl digestion method (Bremner, Citation1996). Total organic carbon (OC) content was determined according to the Walkley–Black wet oxidation method (Nelson & Sommers, Citation1996). Particle size distribution was evaluated using the hydrometer method and sodium hexametaphosphate as a dispersant (Gee & Or, Citation2002). Soil structural index (SI) was estimated according to Reynolds et al. (Citation2007) as:(1)
(1)
Soil moisture retention characteristics of the undisturbed core samples were evaluated using pressure plate extractors (Klute, Citation1986). The moisture content (θ) was measured at 2, −5, −10, −33, −100, −500 and −1,500 kPa suction levels (h). Thermo-gravimetric core method (Blake & Hartge, Citation1986) was employed for bulk density (⍴b) determination.
Relative field capacity (RFC) was calculated as:(2)
(2)
Permanent wilting point (PWP):(3)
(3)
Plant-available water capacity (PAWC):(4)
(4)
Total pore spaces of the soil (f) was estimated as unity minus the fractions of bulk density (⍴b) and particle density (⍴p, assumed to be 2.65 gcm−3)(5)
(5)
Macro pore spaces (PMAC) is defined as:(6)
(6)
Micro pore spaces (PMIC) was therefore calculated as porosity (f) minus macro pore spaces (PMAC)(7)
(7)
The stability of soil aggregates to forces of wind was estimated using dry sieving method (Kemper & Rosenau, Citation1986). A 200 g of air-dried 5 mm sieved sample was weighed and transferred into a nest of sieves consisting of diameters 2, 0.25 and 0.053 mm. The nest of sieves was placed on a mechanical shaker and shaken for 2 min, after which the weight of soil retained on each sieve was taken. Aggregate size distribution was calculated as the fraction of soil retained in the sieve i.e.(8)
(8)
Four aggregate fractions were obtained: 5–2 mm [referred to as large macro-aggregates (Lmag)], 2–0.25 mm [macro-aggregates (Mag)], 0.25–0.053 mm [micro-aggregates (Miag)] and < 0.053 mm [silt + clay (SC)]. The mean weight diameter (MWD) as an index of soil structural stability to wind erosion (Unger, Citation1997) was then calculated as follows:(9)
(9)
where xi = the mean diameter of the class (mm), wi = is the proportion of each size class with respect to the total sample.
2.4. Statistical analysis
The data were subjected to analysis of variance (F-test) using the SAS 9.0 statistical software. Means were compared using a least significant difference (LSD) where analysis of variance (F-test) was significant at p ≤ 0.05. The relationships between the parameters were assessed using bivariate correlation analysis.
3. Results
The results for particle size distribution, OC, SI, TN and bulk density (ρb) are provided in Table . The three land use systems (ARL, GFL and JCL) did not demonstrate significant variation in clay, silt and sand contents, where all the three land use systems have a loam textural class. Similar textural class (loam) was likewise identified in all the sampled soil depths with clay content significantly increasing with depth. Soil OC content was statistically (p ≤ 0.05) higher in GFL (8.44 g kg−1), followed by JCL (6.69 g kg−1) and ARL (6.39 g kg−1) that were statistically comparable. This showed that GFL store OC of about 26.2 and 32.1% more than in JCL and ARL, respectively. Statistically analogous values of the SI were also obtained in all the three land use systems. Similar TN content was found in GFL (0.36 g kg−1) and ARL (0.33 g kg−1) land use systems, which being significantly (p ≤ 0.05) lower than the content under JCL (0.53 g kg−1). When averaged across the three land use systems, TN content in JCL was 47.2 and 60.6% higher than the content in GFL and ARL, respectively. Soil bulk density (ρb) was statistically (p ≤ 0.05) higher in GFL (1.63 Mg m−3), followed by JCL (1.56 Mg m−3) and lowest in ARL (1.48 Mg m−3).
Table 1. Influence of land use systems and soil depth on particle size distribution, OC, SI, TN and bulk density (ρb)
The results for soil moisture retention characteristics [RFC, PWP and PWAC] and pore size distribution [total pore spaces (f), macro-pore spaces (PMAC) and micro-pore spaces (PMIC)] are shown in Table . RFC was significantly (p ≤ 0.05) higher in GFL (81.84%, m3 m−3) compared to JCL (79.44%) and ARL (51.74%) that were statistically at par. Interaction between land use systems and soil depth in RFC was also expressive. The highest RFC (87.44%) was seen in 0–5 cm under GFL and lowest (50.20%) in 10–15 cm under ARL (Figure ). Similarly, PWP was in the following decreasing order among the three land use systems: GFL (26.55%) > JCL (21.62%) > ARL (14.03%). PAWC was higher and statistically comparable in ARL (10.53%) and JCL (8.93%) opposed to the lowest capacity value observed in GFL (6.75%). Total pore spaces (f) were significantly higher in ARL (44.09%), followed by JCL (41.08%), with the lowest value realized in GFL (38.53%). Comparable percentage volume of PMAC was gained in GFL (7.92%) and JCL (7.99%), these being statistically lower than in ARL (23.44%). Conversely, GFL and JCL had sharply higher percentage volume of micro-pore spaces (PMIC) in relation to the smallest percentage volume observed in ARL.
Table 2. Influence of land use systems and soil depth on soil moisture retention characteristics [RFC, PWP and PWAC] and pore size distribution [total pore-spaces (f), PMAC and micro-pore spaces (PMIC)]
The three land use systems had significant (p ≤ 0.05) effect on the dry aggregate size distribution and MWD (Table ). Large-macro aggregates [LMag (5–2 mm)] and MWD were highest in JCL, followed by GFL and lowest in ARL. JCL and GFL had the highest distribution of macro-aggregates [Mag (2–0.25 mm)] when linked to the smallest value obtained in ARL. If averaged across the land uses, JCL has higher Lmag, Mag and MWD of 12.5–68.8, 4.4–27.3 and 8.57–44.76% when compared to GFL and ARL, respectively. The micro-aggregates [Miag (0.25–0.05 mm)] were in the following decreasing order among the land use systems: ARL (0.46) > GFL (0.31) > JCL (0.25). This means that ARL has higher distribution of Miag by about 48.39 and 84.0% in comparison to GFL and JCL, respectively. The land use systems had no significant effect on the silt + clay [SC (<0.05 mm)] fractions. Large-macro aggregates (LMag) and MWD showed a sharply decreasing pattern with depth as opposed to the distribution of Mag. Additionally, the interaction between land use systems and soil depths was also worth noting for the fraction of LMag, Miag and MWD. Highest and lowest LMag and MWD were found in 15–20 cm under JCL and 5–10 cm under ARL respectively (Figures and ). Contrariwise, highest and lowest Miag fractions were seen in 5–10 cm under ARL and 15–20 cm under JCL respectively (Figure ).
Table 3. Influence of land use systems and soil depth on dry aggregate fraction [large macro-aggregates (LMag), macro-aggregates (Mag), micro-aggregates (Miag), silt + clay (SC) and MWD
4. Discussion
The loamy texture of the fields indicates a moderately coarse nature of the soils. The increase of clay content with depth shows downward eluviation of clay from overlaying eluvial A horizon to the underlying illuvial B horizon, this fits well with characteristics of an Alfisol in USDA soil taxonomy and was similarly reported by Maniyunda and Gwari (Citation2014) in a tropical Alfisol of northern Nigeria. The higher OC content at GFL of 26.2–32.1% over JCL and ARL might be tied to the deposition of plant litter material and root turnover with limited soil disturbance by tillage operations as well as cattle droppings during grazing cycle. Moges and Holden (Citation2008) reported high OC in pasture fallows, hence, better carbon sequestration potential than continuous arable cultivation. The SI as a measure of structural degradation of soil did not significantly differ between the three land use systems, where all the values fell below the optimal range of 7%. Low values of SI observed in this study indicate structurally degraded condition of the fields (Reynolds et al., Citation2007). One of the probable reasons for low SI was sub-optimal level of OC observed in all the three land use systems. Although nitrogen content (TN) in all the land use systems was classified as low according to Esu (Citation1991), JCL had significantly higher nitrogen content (47.2–60.6% higher) than GFL and ARL. Jatropha is deciduous xerophytes that shed its leaves at a particular period of the year producing a litter material that can be as much as 2.27 tons ha−1 in a year (Abugre, Oti-Boateng, & Yeboah, Citation2011). The litter material was found to have a relatively low C: N (Abugre et al., Citation2011) which dictates the high decomposition rate accompanied by high residual nitrogen and low residual OC content in the soil. Higher bulk density (ρb) in GFL could be ascribed to traffic induced compaction resulting from cattle trampling during grazing. The low ρb in ARL might not be surprising due to the fact that the study depth was limited to the upper 20 cm; tillage operation creates loose soil in the plough horizon and subsequent compaction of layer beneath the plough layer reported in literature at the depth of 25–50 cm, called plough sole (Anusontpornperm, Thanachit, Suddhiprakarn, & Kheoruenromne, Citation2014; Marcinek, Komisarek, & Kazmierowski, Citation1995; Miatkowski, Citation1998).
The higher RFC and PWP in GFL compared to the other land use systems were equally the result of its high ρb. Hillel (Citation2004) reported that intermediate pores are likely to be greater in compacted soil with increased ρb because most of the originally larger inter-aggregate pores (macropores) have been squeezed into intermediary size (micropores). Micropores (PMIC) were positively correlated with RFC and PWP (Table ) with a coefficient of 0.72** and 0.58** respectively. This supported that RFC and PWP are largely characteristics of PMIC. Therefore, the high RFC and PWP in GFL were the consequence of its high ρb and the corresponding high PMIC. Conversely, ρb was negatively correlated (Table ) with total pore spaces (f) and macroporosity (PMAC) with coefficient of −1.00** and −0.50** respectively; this explained that the significantly higher PAWC, f and PMAC in ARL over the other land use systems must be associated with its low bulk density. The values of PWAC in all the three land use systems were less than the optimal range of ≥15.0% (Reynolds, Drury, Tan, Fox, & Yang, Citation2009), therefore, considered limited for ideal root growth and function. GFL and JCL have their PMAC within the optimal range (5–10%), while ARL had RFC above the upper critical limit (10%) as described by Reynolds et al. (Citation2009); this suggests that all the fields still have capability to quickly drain excess water and facilitate good aeration despite being structurally degraded.
Table 4. Correlation matrix among the parameters
The result presented above showed a substantial fraction of large macro-aggregates (LMag), macro-aggregates (Mag) and MWD in JCL over GFL and ARL to 12.5–68.8, 4.4–27.03 and 8.57–44.76% respectively. In contrary, fraction of micro-aggregates (Miag) was highest in ARL by 48.39–84.0% than in GFL and JCL respectively. This explains the relevant effect of tillage in breaking and destroying large aggregate fractions of the soil. The high MWD indicates the possibility of soil under Jatropha to better withstand forces of wind erosion than soils under GFL and ARL. Ogunwole, Chaudhary, Daudu, Chikara, and Patolia (Citation2008) reported 11 and 2% increase in MWD and macro-aggregates stability in soil under Jatropha in comparison to the native vegetation. This might be associated with the symmetrical nature of the Jatropha plant rooting system, where the lateral roots positively influence the aggregate stability of the soil through the additional cohesion of soil particles (Reubens et al., Citation2011); as well as through root exudates and microbial excretions into the soil, which act as cementing agents of the soil particles (Haynes & Francis, Citation1993). The insignificant difference between the land use systems in the stability of silt + clay fractions, shows land use only affect the dry aggregates stability of the soil at only macro and micro levels.
5. Conclusions
(1) | Despite the benefit of turnover increase in soil organic matter through cattle droppings during grazing cycle as perceived by peasant farmers in Northern Nigeria and elsewhere; it’s might result to soil compaction of the surface layer more devastating than conventional arable cultivation as showed by this study. | ||||
(2) | Use of disc plough in conventional tillage for arable crop production could result in low bulk density in the upper 20 cm, while the compacted layer might be found beneath this layer. Therefore, subsequent researches investigating the effect of tillage on soil compaction are advised to go beyond the upper 20 cm soil layer. | ||||
(3) | Jatropha (J. curcas L.) showed some possibilities of enriching nitrogen and stability of Nigerian Savanna Alfisol soil to wind erosion better than CGL and arable cultivation. But, still a long term study on the influence of Jatropha (J. curcas L.) on soil quality is needed in order to understand if such possibilities are sustainable. |
Additional information
Funding
Notes on contributors
B.M. Shehu
B.M. Shehu was a graduate of Bayero University Kano, Nigeria and now an assistant lecturer in the Department of Soil Science, Bayero University Kano, Nigeria. His areas of research interest are soil physics, soil and water conservation, soil fertility management and plant nutrition.
J.O. Ogunwole
J.O. Ogunwole was a graduate of Ahmadu Bello University Zaria, Kaduna State, Nigeria and now a professor in the Department of Crop Production and Protection, Federal University, Dutsin-Ma, Katsina State, Nigeria. His areas of research interest are soil physics, soil ecology, soil organic carbon sequestration, soil and water conservation, and plant-soil-water relations.
J.M. Jibrin
J.M. Jibrin was a graduate of Ahmadu Bello University Zaria, Kaduna State, Nigeria and now a Professor in the Department of Soil Science, Bayero University Kano, Nigeria. His areas of research interest are soil fertility management, plant nutrition, and crop modeling.
References
- Abugre, S., Oti-Boateng, C., & Yeboah, M. F. (2011). Litter fall and decomposition trend of Aropha curcas L. leaves mulches under two environment conditions. Agriculture and Biology Journal of North America, 2, 462–470.
- Anusontpornperm, S., Thanachit, S., Suddhiprakarn, A., & Kheoruenromne, I. (2014, July 06–10). Is rippering the effective solution to alleviating plough pan problem? Zurich: Proceedings International Conference of Agricultural Engineering. Retrieved from www.eurageng.eu
- Azam, M. M., Waris, A., & Nahar, N. M. (2005). Prospects and potential of fatty acid methyl esters of some non-traditional seed oils for use as biodiesel in India. Biomass and Bioenergy, 29, 293–202.
- Batjes, N. H. (2001). Options for increasing carbon sequestration in West African soils: An exploratory study with special focus on Senegal. Land Degradation and Development, 12, 131–142.10.1002/(ISSN)1099-145X
- Blake, G. R., & Hartge, K. H. (1986). Bulk density, methods of soil analysis. In A. Klute (Ed.), Part 1. Physical and mineral method (pp. 363–382). Madison: American Society of Agronomy (ASA) and Soil Science Society of America (SSSA).
- Bremner, J. M. (1996). Nitrogen-total. In D. L. Sparks (Ed.), Methods of soils analysis: Chemical methods (pp. 1085–1121). Madison, WI: American Society of Agronomy (ASA) and Soil Science Society of America (SSSA).
- Dexter, A. R. (2004). Soil physical quality. Geoderma, 120, 201–214.10.1016/j.geoderma.2003.09.004
- Esu, I. E. (1991). Detailed soil survey of NIHORT Farm at Bunkure Kano State, Nigeria. Zaria: Institute for Agricultural Research Samaru.
- Gee, G. W., & Or, D. (2002). Particle-size analysis. In J. H. Dane & G. C. Topp (Eds.) Methods of soil analysis. Part 4. Physical methods (pp. 255–293). Madison, WI: Soil Science Society of America Book Series 5.
- Haynes, R. J., & Francis, G. S. (1993). Changes in microbial biomass C, soil carbohydrate composition and aggregate stability induced by growth of selected crop and forage species under field conditions. Journal of Soil Science, 44, 665–675.10.1111/ejs.1993.44.issue-4
- Hillel, D. (2004). Introduction to environmental soil physics (p. 115). San Diego, CA: Elsevier Science.
- Jongschaap, R. E. E., Corre, W. J., Bindraban, P. S., & Brandenburg, W. A. (2007). Claims and facts on Jatropha curcas L.: Global Jatropha curcas evaluation, breeding and propagation Programme. Wageningen: Plant Research International, B.V.
- Kemper, W. D., & Rosenau, R. C. (1986). Aggregate stability and size distributions. In A. Klute (Ed.), Methods of soil analysis, Part 1: Agronomy monograph (2nd ed., pp. 425–442). Madison: ASA and SSSA.
- Klute, A. (1986). Water retention: Laboratory method. In A. Klute (Ed.), Methods of soil analysis, Part 1: Physical and mineralogical properties, monograph 9 (pp. 687–734). Madison, WI: ASA.
- Maniyunda, L. M., & Gwari, M. G. (2014). Soils development on a toposequence on loessial deposit in Northern Guinea Savanna, Nigeria. ARPN Journal of Agricultural & Biological Science, 9, 110–116.
- Marcinek, J., Komisarek, J., & Kazmierowski, C. (1995). Degradacja fizyczna gleb płowychi czarnych ziem intensywnie uzytkowanych rolniczo w Wielkopolsce [Physical degradation of soils utilized intensely for farming in Wielkopolska]. Zeszyty Problemowe Postepow Nauk Rolniczych, 418, 141–147 (in Polish).
- Miatkowski, Z. (1998). Zageszczenie podglebia jako element procesu fizycznej degradacji gleb [Compaction of subsoil as an element of the process of physical degradation of soil]. Zeszyty Problemowe Postepow Nauk Rolniczych, 460, 431–443 (in Polish).
- Moges, A., & Holden, N. M. (2008). Soil fertility in relation to slope position and agricultural land use: A Case study of umbulo catchment in Southern Ethiopia. Environmental Management, 42, 753–763.10.1007/s00267-008-9157-8
- Nelson, D. W., & Sommers, L. E. (1996). Total carbon, organic carbon and organic matter. In J. M. Bigham (Ed.), Methods of soil analysis: Part 3 chemical and microbiological properties (pp. 961–1010). Madison, WI: ASA, CSSA, SAAJ.
- Ogunwole, J. O., Babalola, O. A., Oyinlola, E. Y., & Raji, B. A. (2001). A pedological characterization of soils in the Samaru area of Nigeria. Samaru Journal of Agricultural Research, 17, 71–77.
- Ogunwole, J. O., Chaudhary, D. R., Daudu, C. K., Chikara, J., & Patolia, J. S. (2008). Contribution of Jatropha curcas to soil quality improvement in a degraded Indian Entisol. Acta Agriculture Section B - Soil and Plant Science, 58, 245–251.
- Oluwasemire, K. O., & Alabi, S. O. (2004). Ecological impact of changing rainfall pattern, soil processes and environmental pollution in the Nigerian Sudan and Northern Guinea Savanna agro-ecological zones. Nigerian Journal of Soil Resources, 5, 23–31.
- Peterson, R. G., & Calvin, L. D. (1986). Sampling. Methods of soil analysis 1, Physical and mineralogical methods (Soil Science Society of America). Agronomy, 9, 33–51.
- Reubens, B., Achten, W. M. J., Maes, W. H., Danjon, F., Aerts, R., Poesen, J., & Muys, B. (2011). More than biofuel? Jatropha curcas root system symmetry and potential for soil erosion control. Journal of Arid Environments, 75, 201–205.10.1016/j.jaridenv.2010.09.011
- Reynolds, W. D., Bowman, B. T., Drury, C. F., & Tan, C. S. (2002). Indicators of good soil physical quality: Density and storage parameters. Geoderma, 110, 131–146.10.1016/S0016-7061(02)00228-8
- Reynolds, W. D., Drury, C. F., Yang, X. M., Fox, C. A., Tan, C. S., & Zhang, T. Q. (2007). Land management effects on the near-surface physical quality of a clay loam soil. Soil and Tillage Research, 96, 316–330.10.1016/j.still.2007.07.003
- Reynolds, W. D., Drury, C. F., Tan, C. S., Fox, C. A., & Yang, X. M. (2009). Use of indicators and pore volume-function characteristics to quantify soil physical quality. Geoderma, 152, 252–263.10.1016/j.geoderma.2009.06.009
- Salako, F. K., Hauser, S., Babalola, O., & Tian, G. (2001). Improvement of the physical fertility of a degraded Alfisol with planted and planted natural fallow under humid tropical conditions. Soil use and manage, 17, 41–47.
- Scherr, S. J. (1999, February). Soil degradation: A threat to developing–country food security by 2020?(Discussion Paper 27). IFPRI Food, Agriculture and the Environment, Washington, DC, 20006-1002 USA.
- Singh, P., Singh, S., Mishara, S. P., & Bhatia, S. K. (2010). Molecular characterization of genetic diversity in Jatropha curcas L. Genes Genomes and Genomics, 4, (Special issue 1), 1–8.
- Topp, G. C., Reynolds, W. D., Cook, F. J., Kirby, J. M., & Carter, M. R. (1997). Physical attributes of soil quality. In E. G. Gregorich & M. R. Carter (Eds.), Soil quality for crop production and ecosystem health (pp. 21–58). New York, NY: Elsevier.10.1016/S0166-2481(97)80029-3
- Unger, P. W. (1997). Management-induced aggregation and organic carbon concentrations in the surface layer of a Torrertic Paleustoll. Soil and Tillage Research, 42, 185–208.10.1016/S0167-1987(97)00003-2