Abstract
The effects of the combined use of cell-free supernatant (CFS) and grape seed extract (GSE) in inhibiting the growth of pre-existing and inoculated foodborne bacteria on mixed, ready-to-eat (RTE) baby leaf vegetables were examined. Lactobacillus brevis WK12 and Leuconostoc mesenteroides WK32 produced significantly more acetic and lactic acid than citric, malic, succinic, or fumaric acids after 24 h of fermentation. The inhibition zone sizes observed when Lactobacillus brevis WK12 and Leuconostoc mesenteroides WK32 CFSs and 0.1% GSE were used in combination were much greater than those observed when either of the CFSs alone was used on agar plates. The application of 5% CFS from Lactobacillus brevis WK12 or Leuconostoc mesenteroides WK32 in combination with 0.1% GSE reduced the total counts of aerobic mesophilic bacteria, coliforms, and yeasts/molds in RTE baby leaf vegetable samples by 2.01, 1.74, and 1.87 log CFU/g, respectively, compared with those determined in the control. Combined CFS and GSE treatment reduced Escherichia coli O157:H7 and Listeria monocytogenes counts by 1.72 and 2.89 log CFU/g, respectively. These results suggest that the combined CFS and GSE treatment may act as a natural disinfectant, enhancing the safety of RTE baby leaf vegetables.
Public Interest Statement
Chlorine is commonly used in the form of hypochlorous acid, and its use has already been prohibited in some European countries, due to the potential of toxic by product generation. Therefore, there is a need for a microbial decontamination method that is non-toxic, effective, and easily applicable. The results of this study provide clear evidence demonstrating that a natural sanitizer comprised of cell-free supernatant (CFS) and grape seed extract (GSE) can be useful for the reduction of native microbial flora and foodborne pathogens in mixed baby leaf vegetables. Particular importance are the results demonstrating that the greatest effects of Lactobacillus brevis WK12 or Leuconostoc mesenteroides WK32 CFSs on RTE baby leaf vegetables were achieved when CFSs were used in combination with GSE. Therefore, as an alternative to chlorine sanitation, combined washing with CFSs and GSE can improve the microbial safety of RTE baby leaves.
Competing Interests
The authors declare no competing interest.
1. Introduction
The consumption of fresh produce and ready-to-eat (RTE) vegetables has increased as they represent important natural sources of dietary fibre, phytochemicals, minerals, and vitamins (Zheng et al., Citation2013). However, consumers are also concerned about the safety of RTE leaf vegetables consumed raw in salads, burgers, and Bibimbap (a typical Korean dish) and increasingly demand foods free of toxins and harmful microorganisms (Shah et al., Citation2015). However, raw vegetables are expected to contain pathogenic bacteria, and minimal processing (washing, peeling, cutting, and packing) may increase the growth of spoilage microorganisms (Fröder et al., Citation2007; Santos, Martins, Pedroso, Sousa, & Ferreira, Citation2015). Levels of mesophilic and psychrotrophic microorganisms in the range of 103–108 CFU/g are commonly found in RTE vegetables (Siroli et al., Citation2015). In particular, data from previous studies have shown that the bacterial contaminants found in RTE vegetables include primarily Escherichia coli O157:H7 and Listeria monocytogenes strains (Alegre, Abadias, Anguera, Usall, & Viñas, Citation2010; Siroli et al., Citation2015). E. coli O157:H7 is one of the most prevalent enterohaemorrhagic strains of E. coli, causing approximately 30% of pathogenic infections in Korea, and it can cause serious diseases, such as haemolytic uremic syndrome (Jo et al., Citation2004). Listeria monocytogenes is a psychrotrophic pathogen that is able to grow under refrigeration, although at considerably lower growth rates (Dimitrijevic et al., Citation2006).
To improve the microbial safety of RTE vegetables, various chemical washing treatments, including sodium hypochlorite, ozone, sodium lactate, and electrolysed water have been used to reduce bacterial counts (Beuchat & Ryu, Citation1997; Chaidez, Lopez, Vidales, & Castro-Del Campo, Citation2007; Oh et al., Citation2014).
Chlorine is widely used in the fresh-cut industry due to its relatively low price, ease of application, and a wide spectrum of antimicrobial effects (Meireles, Giaouris, & Simões, Citation2016). However, the use of chlorine as a sanitizer in the RTE produce industry has been identified as a health concern, mainly due to the presence of trihalomethanes, haloacetic acids, and chlorophenols generated during chlorination (Bull et al., Citation2011). In fact, chlorine is commonly used in the form of hypochlorous acid, and its use has already been prohibited in some European countries, due to the potential of toxic by-product generation (Gil, Selma, López-Gálvez, & Allende, Citation2009). Ozone (O3) is produced as a gas that can be dissolved in washing water. Ozone is unstable, rapidly decomposes, and can become very toxic as it can affect the respiratory tract and cause irritation to the eyes and throat (Meireles et al., Citation2016). Therefore, a need for a microbial decontamination method that is non-toxic, effective, and easily applicable exists, and many studies have been conducted in order to investigate potential disinfectants as effective alternatives to chlorine or ozone.
Lactic acid bacteria (LAB) have shown great potential as biocontrol agents that control the growth of pathogenic organisms in food products, because they have a long history of safe use (Carr, Chill, & Maida, Citation2002). Cell-free supernatants (CFSs) of LAB isolated from fermented vegetables and dairy products contain antimicrobial organic acids, such as acetic, lactic, and citric acids (Rattanachaikunsopon & Phumkhachorn, Citation2010; Salleh, Lani, & Ismail, Citation2014).
Additionally, grape seed extract (GSE) is a polyphenol-rich by-product derived from the seeds of grape, Vitis vinifera. Antioxidant and antimicrobial activity of GSE against foodborne pathogens is well documented (Baydar, Özkan, & Sağdiç, Citation2004; Jayaprakasha, Selvi, & Sakariah, Citation2003). Furthermore, the use of GSE at a concentration of 0.01–1% along with other growth retardants, such as low pH and temperature, showed synergistic antimicrobial activity in RTE vegetables and fruits (Xu et al., Citation2007). However, to the best of our knowledge, no previous reports demonstrated the effects of the combination of GSE and CFSs produced by LAB as a natural washing sanitizer for RTE vegetables.
Therefore, this study was conducted to investigate the inhibitory effects of CFSs produced by LAB isolated from cabbage kimchi in combination with GSE against pre-existing microorganisms and foodborne pathogens on RTE baby leaf vegetables.
2. Material and methods
2.1. RTE baby leaf vegetables
Mixed RTE baby leaf vegetables were purchased from a local market in Gwangju, Korea. RTE baby leaves were obtained from red beet (Beta vulgaris L.), amaranth (Amaranthus caudatus), and red radish (Raphanus sativus L.).
2.2. LAB growth conditions and CFS preparation
Selected Lactobacillus brevis WK12 and Leuconostoc mesenteroides WK32 strains, isolated from well-fermented cabbage kimchi (Gwak et al., Citation2015), were obtained from the Microorganism and Gene Bank at the World Institute of Kimchi. de Man, Rogosa & Sharpe Broth (MRS, Difco Co., Detroit, MI, USA) was used for Lactobacillus brevis WK12 and Leuconostoc mesenteroides WK32 seed culture. Lactobacillus brevis WK12 fermentation food-grade medium consisted of 4% lactose (Sung Poong Co. Ltd., Seoul, Korea), 2% yeast extract (Angest, Yichang, China), 0.5% sodium acetate (Qingdao Twell Sansino Import & Export Co., Qingdao, China), and 0.2% dipotassium hydrogen phosphate (Youngjin Inc., Yesan, Korea). Leuconostoc mesenteroides WK32 fermentation food-grade medium consisted of 5% lactose, 1% yeast extract, 0.5% sodium acetate, and 0.2% dipotassium hydrogen phosphate. Seed-cultivated solutions were added at 1% (v/v) to a 5 L jar fermenter (Bio CnS Co., Daejeon, Korea) containing 3 L of fermentation medium. Lactobacillus brevis WK12 and Leuconostoc mesenteroides WK32 cultures were fermented under aerobic and anaerobic conditions at 30°C and 500 rpm, respectively. During the fermentation, pH values of these cultures were determined using a digital pH meter (Inpro3030, Mettler Toledo, Columbus, OH, USA), and cell mass in each culture was measured using a UV spectrophotometer (UV-1800; Shimadzu, Tokyo, Japan) at 600 nm. Optical density (OD) values were converted to log CFU/mL values using a standard calibration curve.
After fermentation, Lactobacillus brevis WK12 and Leuconostoc mesenteroides WK32 cultures were subjected to high-pressure saturated steam at 121°C for approximately 20 min. After sterilization, the cultures were centrifuged at 5,000 ×g (5810R centrifuge; Eppendorf, Hamburg, Germany) at 4°C for 15 min and then filtered through a 0.45 μm membrane filter to obtain CFSs.
2.3. High-performance liquid chromatography (HPLC)-based analysis of organic acid contents in CFSs
Organic acid contents in CFSs were analysed using a Waters Alliance e2695 HPLC system (Waters Co., Milford, MA, USA) according to the methods of Koo, Kim, and Kang (Citation2015) and Özcelik, Kuley, and Özogul (Citation2016), with some modifications. CFSs were filtered through a 0.45 μm nylon syringe filter (Whatman filters, GE healthcare, Chicago, IL, USA). Separations were performed using an Aminex HPX-87H reversed-phase column (7.8 × 300 mm, 9 μm particle size; BioRad, Berkeley, CA, USA) at 25°C, and 10 μL of each sample was injected. The mobile phase consisted of distilled 0.008 N H2SO4 (100%, v/v) with a flow rate of 0.4 mL/min. The organic acid contents in the CFSs were determined by monitoring the absorbance at 210 nm using a UV detector, and quantified by comparisons with the retention time and peak area of a mixture of 6 standard organic acids (lactic, acetic, fumaric, succinic, malic, and citric acids). The organic acid content was expressed in g/L.
2.4. Determination of antimicrobial activities
Agar disk diffusion assay (Chen et al., Citation2016) was used to determine the antibacterial activity of CFSs in combination with GSE against 2 target foodborne pathogens. Tryptic soy agar (Difco Co.) was cooled to 45°C after autoclaving, inoculated with 0.2 mL overnight cultures, and placed in sterile petri dishes. After solidification in a laminar flow biosafety hood for 60 min, sterile paper disks (6 mm diameter; Toyo Ltd., Tokyo, Japan) were placed on the agar and the plates were kept at room temperature for 30 min to allow liquid absorption into the disk. Ten microliters of CFS alone or CFS in combination with GSE was pipetted onto the disks, and the plates were incubated at 37°C for 24 or 48 h. Following the incubation, antimicrobial activities were estimated by measuring the diameters (mm) of the zones of growth inhibition. The assays were performed in triplicate.
2.5. Foodborne pathogen strains and preparation of inocula
E. coli O157:H7 (NCTC 12079) and Listeria monocytogenes (ATCC 19111) strains, obtained from the American Type Culture Collection (ATCC) or from our own culture collection, were used for inoculation. E. coli O157:H7 and Listeria monocytogenes strains from frozen stock were streaked onto the tryptic soy agar (Difco Co.) and incubated at 37°C for 24 h. Single colonies of E. coli O157:H7 and Listeria monocytogenes were added to 50 mL tubes containing 30 mL of tryptic soy broth (Difco Co.), and incubated overnight at 37°C with shaking (150 rpm). From these overnight cultures, 1 mL each of E. coli O157:H7 and Listeria monocytogenes cultures were transferred to 500 mL of fresh medium and incubated for 24 h at 37°C with shaking (150 rpm). Harvested bacterial cell cultures were centrifuged at 4,000 ×g for 15 min, and the cultures were washed twice with sterile water containing 0.1% peptone. Inoculation solutions of E. coli O157:H7 and Listeria monocytogenes were prepared by diluting the bacteria in distilled water to a concentration of 7–8 log CFU/mL.
2.6. Inoculation of E. coli O157:H7 and Listeria monocytogenes on RTE baby leaves
Mixed RTE baby leaf vegetables were dipped in 70% ethyl alcohol and then exposed to UV-C irradiation for 20 min in a laminar flow hood equipped with UV-C lamps (40 W) to remove pre-existing microorganisms. The samples were dipped in a bacterial inoculum solution at a ratio of 1:10 (w/v) and agitated by stirring with a glove-covered hand for 15 min. The inoculated baby leaf samples were then air-dried in a laminar flow hood for 30 min to allow attachment of the pathogens to the surface.
2.7. RTE baby leaf vegetable washing
To prepare sanitizer solutions containing CFSs and GSE, Lactobacillus brevis WK12 and Leuconostoc mesenteroides WK32 CFSs were dissolved in sterile distilled water to a concentration of 5% and pH values of 4.08 and 4.50, respectively. GSE was dissolved in these solutions to a concentration of 0.1%. Mixed baby leaf samples were submerged in a bath containing distilled water, 100 ppm sodium hypochlorite, 5% CFS, or 5% CFS plus 0.1% GSE (1:10, baby leaf: washing solution, w/v), with gentle agitation for 5 min. Untreated baby leaf samples were used as controls.
2.8. Microbiological analysis
After washing, 20 g of baby leaf samples were placed in a sterile stomacher bag with a polyethylene filter layer containing 180 mL of peptone water (0.1% sterile peptone, w/v). Samples were then homogenised using a stomacher blender (MIX 2, AES Laboratoire, France) for 3 min and diluted with peptone water for microbial counting. Serial dilutions were performed in triplicate. For total aerobic mesophilic bacterial counts, samples were plated onto 3 M Petrifilm Aerobic Count Plates (Petrifilm AC; 3 M Co., St. Paul, MN, USA) and incubated at 37°C for 48 h. Coliforms were counted using 3 M Petrifilm E. coli/Coliform Count Plates incubated at 37°C for 24 h. Yeast/molds were plated on 3 M Petrifilm Yeast and Mold Count Plates (Petrifilm YM; 3 M Co.) and incubated at 25°C for 72 h. E. coli O157:H7 and Listeria monocytogenes counts were determined by plating diluted samples onto Sorbitol-MacConkey agar (Difco Co.) and Modified Oxford agar (Difco Co.) and incubating the plates at 37°C for 24 and 48 h, respectively. Each microbial count was determined as the mean of 3 measurement results and was expressed as log CFU/g.
2.9. Statistical analysis
Analysis of variance and Duncan’s multiple range tests were performed using the SPSS software package (Version 19, SPSS Inc., Chicago, IL, USA), and differences were considered significant at p < 0.05. Each microbial count represents the average of 3 determinations.
3. Results and discussion
3.1. Growth characteristics of Lactobacillus brevis WK12 and Leuconostoc mesenteroides WK32 during fermentation
The growth rates and pH changes in the cultures of Lactobacillus brevis WK12 and Leuconostoc mesenteroides WK32 during fermentation are shown in Figure . Under aerobic conditions, the cell mass of Lactobacillus brevis WK12 gradually increased in a time-dependent manner during 24 h of fermentation (Figure (a)). The maximum cell mass of 10.02 log CFU/mL was measured at 24 h. The pH of Lactobacillus brevis WK12 culture gradually decreased until it reached the minimum at 3.85. For Leuconostoc mesenteroides WK32, the cell mass rapidly increased to 9.99 log CFU/mL up during the first 9 h of fermentation and it remained stable under anaerobic conditions thereafter. The pH of the sample was shown to be 4.2 after 9 h of fermentation (Figure (b)). The pH markedly decreased with Leuconostoc mesenteroides WK32 cell mass increase.
3.2. Organic acid content analysis following the fermentation
The organic acids produced by Lactobacillus brevis WK12 and Leuconostoc mesenteroides WK32 after fermentation were analysed by HPLC (Figure ). Among 6 organic acids, the major components found in Lactobacillus brevis WK12 and Leuconostoc mesenteroides WK32 CFSs were lactic and acetic acids. In particular, higher acetic and lactic acid contents were detected in the Lactobacillus brevis WK12 CFS, with concentrations of 12 and 14 g/L, respectively, than in CFSs of Leuconostoc mesenteroides WK32. Gerez, Torino, Rollán, and Font de Valdez (Citation2009) reported that the production levels of lactic and acetic acids during Lactobacillus brevis fermentation were 1 and 3.8 g/L, respectively. A previous study showed that lactic (8.4 g/L) and acetic (6.6 g/L) acids were produced by Leuconostoc mesenteroides at higher levels than other organic acids, such as citric, succinic, and malic acid (Lee & Chang, Citation2016). The levels and types of organic acids produced during fermentation were shown to depend on LAB species, culture composition, and growth conditions (Özcelik et al., Citation2016).
Figure 2. Organic acid content (g/L) in CFSs produced by Lactobacillus brevis WK12 and Leuconostoc mesenteroides WK32 after fermentation.
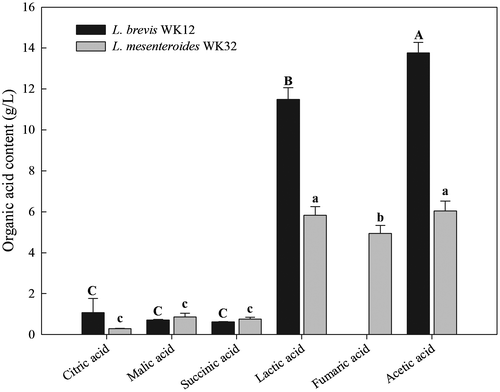
3.3. Determination of antimicrobial activity
The antimicrobial activities of CFSs alone or in combination with GSE, against E. coli O157:H7 or Listeria monocytogenes were examined using the agar diffusion test and the obtained results are presented in Table . Lactobacillus brevis WK12 CFSs exhibited inhibitory effects, producing zones of inhibition for E. coli O157:H7 and Listeria monocytogenes. Combination treatment with Lactobacillus brevis WK12 CFS and GSE showed better overall antimicrobial activities than GFS treatment alone against E. coli O157:H7 and Listeria monocytogenes. The antimicrobial effects were shown to increase with increased GSE concentrations in CFSs. Similarly, the inhibition zone sizes obtained when Leuconostoc mesenteroides WK32 CFSs and GSE were used were much greater than those observed when either CFS was used alone. Tejero-Sariñena, Barlow, Costabile, Gibson, and Rowland (Citation2012) reported a strong correlation between the organic acid concentration, organic acid species, pH, and microbial inactivity. Low pH makes organic acids liposoluble, allowing them to traverse the cell membrane and reach the cytoplasm of microorganisms (Djadouni & Kihal, Citation2012). Acetic acid was reported to have greater antimicrobial activity than lactic acid due to its higher pKa value (acetic acid, 4.75; lactic acid, 3.08) (Tejero-Sariñena et al., Citation2012). Neutralised CFSs (pH-adjusted using 0.1 N NaOH) did not lead to the generation of inhibition zones for E. coli O157:H7 and Listeria monocytogenes (data not shown). These results indicate that growth inhibition of foodborne pathogens may be attributed to the decreased pH values and antibacterial activities of organic acids in CFSs produced by LAB.
Table 1. Antimicrobial activity of CFSs in combination with GSE against Escherichia coli O157:H7 and Listeria monocytogenes, as determined by agar-diffusion testing
3.4. Antimicrobial effects of CFSs and GSE in the treatment of mixed RTE baby leaf vegetables
The effects of CFSs alone or in combination with GSE on the inactivation of natural microflora and foodborne pathogens found on mixed RTE baby leaf vegetables were examined (Table ). Prior to washing, the initial counts of total aerobic mesophilic bacteria, coliforms, and yeast/molds on baby leaf vegetables ranged from 5.93 to 8.57 log CFU/g. These results demonstrate the presence of high levels of natural microflora in commercial baby leaves. Azizkhani, Elizaquível, Sánchez, Selma, and Aznar (Citation2013) reported that baby leaf vegetables can be contaminated by various microorganisms, and high microbial counts in baby leaf vegetables can lead to the development of vegetable-related illnesses. Therefore, sanitization is required for the reduction of microorganism count and to ensure the microbial safety of baby leaf vegetables.
Table 2. Inactivation of the natural microflora and inoculated foodborne pathogens by washing with CFSs in combination with GSE (log CFU/g)
The initial counts of total aerobic mesophilic bacteria were determined to be 8.57 log CFU/g in the control (untreated) group, while the bacterial counts in samples treated with distilled water were 8.14 log CFU/g, indicating only a 0.43 log CFU/g reduction. Treatment with a 100 ppm NaOCl solution resulted in a decrease in the total aerobic mesophilic bacteria count to 7.21 log CFU/g, which is a reduction of 1.36 log CFU/g relative to the control. A single 0.1% GSE, 5% Lactobacillus brevis WK12 CFS, or Leuconostoc mesenteroides WK32 CFS treatment reduced the count of total aerobic mesophilic bacteria in the baby leaf vegetables by 1.60 log CFU/g. The application of CFSs in combination with 0.1% GSE significantly reduced total aerobic mesophilic bacteria counts, in comparison with those determined after the treatment with GSE or either CFS alone.
The presence of coliforms in leafy vegetables is undesirable because it indicates poor hygiene conditions due to contamination or inadequate washing (Choi, Park, Choi, Kim, & Chun, Citation2015). The control was shown to contain 7.00 log CFU/g coliforms, and washing the leafy samples with distilled water led to a reduction in coliform levels to 6.32 log CFU/g, corresponding to the reduction of 0.68 log CFU/g in coliform count (Table ). Similar to the coliform count reductions observed following NaOCl treatment, the coliform counts observed after washing with GSE or either CFS decreased to 5.87–5.97 log CFU/g. Combination treatment with Lactobacillus brevis WK12 or Leuconostoc mesenteroides WK32 CFS and GSE resulted in coliform levels of 5.26 and 5.36 log CFU/g, representing the reduction of 1.74 and 1.64 log CFU/g, respectively, in comparison with that determined in the control sample.
Yeast/mold counts determined in this study showed patterns similar to those of the total aerobic mesophilic bacteria (Table ). Washing with solution that contained 100 ppm NaOCl reduced the counts of yeast/molds in the leaf samples by approximately 1.36 log CFU/g. The hygienic capacity of 100 ppm NaOCl treatment against yeast/molds did not differ significantly from that of 0.1% GSE. In contrast, CFEs from Lactobacillus brevis WK12 and Leuconostoc mesenteroides WK32 in combination with GSE were more effective in reducing the yeast/mold levels, resulting in 1.69 and 1.87 log CFU/g reductions, respectively, relative to the control.
For inoculated RTE baby leaf vegetables, the initial counts of E. coli O157:H7 and Listeria monocytogenes inoculated on the samples were 7.36 and 7.97 log CFU/g, respectively. Washing with 100 ppm NaOCl resulted in E. coli O157:H7 and L Listeria monocytogenes counts of 6.44 and 6.20 log CFU/g, respectively, which represented the reduction of 0.92 and 1.77 log CFU/g, respectively. The combination of Lactobacillus brevis WK12 CFS and GSE reduced the levels of E. coli O157:H7 and Listeria monocytogenes by 5.64 and 5.08 log CFU/g, respectively. Our results indicate that washing with a binary solution of 5% CFS containing 0.1% GSE was more effective than treatments with either 100 ppm NaOCl or 5% CFS alone. Koo et al. (Citation2015) reported that Leuconostoc isolates inhibited both the growth of pathogenic bacteria by producing antimicrobial agents (including organic acids) and the growth of meat spoilage bacteria, and that E. coli O157:H7 growth in ground beef was inhibited by Leuconostoc CFS. Pujato, del L Quiberoni, Candioti, Reinheimer, and Guglielmotti (Citation2014) showed that Leuconostoc citreum CFS significantly reduced Listeria monocytogenes growth in milk. Although the antimicrobial activity of LAB has been previously reported, the present investigation is the first to evaluate the antimicrobial efficacy of combined CFS and GSE treatment. The pH of 5% CFSs, 0.1% GSE, and 5% CFSs in combination with 0.1% GSE ranged from 3.96 to 4.50. We demonstrated that the effectiveness of single or binary sanitizer solutions against microorganisms found in RTE baby leaf vegetable samples is pH-dependent. This is probably due to the fact that the molecular structure, size, and pKa value of acids are important for the effects of these solutions as well. Furthermore, the use of chemical sanitizers with fresh produce is strictly regulated. For example, a maximum of 4 ppm residual chlorine in wastewater discharge is allowed by the U.S. National Organic Program Standard (Zhang, Critzer, Davidson, & Zhong, Citation2014), which can limit the effectiveness of sanitization. In contrast, organic acids are generally recognised as safe, and acetic, citric, and lactic acids are the most common acids used in the food industry for sanitization purposes (Meireles et al., Citation2016). No visible changes of the leaf vegetables were observed after washing them with CFSs alone or in combination with GSE (data not shown). Therefore, our findings provide evidence for the improved antimicrobial activity of Lactobacillus brevis WK12 and Leuconostoc mesenteroides WK32 CFSs in combination with GSE as natural sanitizers of baby leaf vegetables.
4. Conclusions
In summary, the results of this study provide clear evidence demonstrating that a natural sanitizer comprised of CFS and GSE can be useful for the reduction of native microbial flora and foodborne pathogens in mixed baby leaf vegetables. In particular, the greatest inactivation effects by L. brevis WK12 or L. mesenteroides WK32 CFSs on RTE baby leaf vegetables were achieved when used in combination with GSE. Therefore, as an alternative to chlorine sanitation, combined washing with CFSs and GSE can improve the microbial safety of RTE baby leaves. Additionally, combining this treatment with others, such as irradiation, modified atmosphere packaging, and low storage temperature, should be further investigated.
Funding
This work was supported by the WIKIM Institutional Research Program [grant number KE1603–2] through the World Institute of Kimchi funded by the Ministry of Science, ICT, and Future Planning, Republic of Korea.
Additional information
Notes on contributors
Keon Jin Lee
The authors have worked on the WIKIM Institutional Research Project (KE1603-2) through the World Institute of Kimchi funded by the Ministry of Science, ICT and Future Planning, Republic of Korea. The present paper is a part of the project work. In particular, Dr Ho Hyun Chun, the corresponding author, received his PhD from Chungnam National University in 2013. He joined World Institute of Kimchi, Gwanju, Republic of Korea in 2014. He is currently appointed as a senior researcher from Advanced Process Technology and Fermentation Research Group at the Institute. While working with a range of food and agricultural produce preservation-related projects, he has learned that non-thermal technologies such as natural sanitizer, UV-C, and electron beam irradiation can be used to improve food preservation and microbiological safety.
References
- Alegre, I., Abadias, M., Anguera, M., Usall, J., & Viñas, I. (2010). Fate of Escherichia coli O157:H7, Salmonella and Listeria innocua on minimally-processed peaches under different storage conditions. Food Microbiology, 27, 862–868.10.1016/j.fm.2010.05.008
- Azizkhani, M., Elizaquível, P., Sánchez, G., Selma, M. V., & Aznar, R. (2013). Comparative efficacy of Zataria multiflora Boiss., Origanum compactum and Eugenia caryophyllus essential oils against E. coli O157:H7, feline calicivirus and endogenous microbiota in commercial baby-leaf salads. International Journal of Food Microbiology, 166, 249–255.10.1016/j.ijfoodmicro.2013.07.020
- Baydar, N. G., Özkan, G., & Sağdiç, O. (2004). Total phenolic contents and antibacterial activities of grape (Vitis vinifera L.) extracts. Food Control, 15, 335–339.10.1016/S0956-7135(03)00083-5
- Beuchat, L. R., & Ryu, J. H. (1997). Produce handling and processing practices. Emerging Infectious Diseases, 3, 458–465.
- Bull, R. J., Reckhow, D. A., Li, X., Humpage, A. R., Joll, C., & Hrudey, S. E. (2011). Potential carcinogenic hazards of non-regulated disinfection by-products: Haloquinones, halo-cyclopentene and cyclohexene derivatives, N-halamines, halonitriles, and heterocyclic amines. Toxicology, 286, 1–19.10.1016/j.tox.2011.05.004
- Carr, F. J., Chill, D., & Maida, N. (2002). The lactic acid bacteria: A literature survey. Critical Reviews in Microbiology, 28, 281–370.10.1080/1040-840291046759
- Chaidez, C., Lopez, J., Vidales, J., & Castro-Del Campo, N. C. (2007). Efficacy of chlorinated and ozonated water in reducing Salmonella typhimurium attached to tomato surfaces. International Journal of Environmental Health Research, 17, 311–318.10.1080/09603120701417063
- Chen, X., Zhang, X., Meng, R., Zhao, Z., Liu, Z., Zhao, X., Shi, C., & Guo, N. (2016). Efficacy of a combination of nisin and p-Anisaldehyde against Listeria monocytogenes. Food Control, 66, 100–106.10.1016/j.foodcont.2016.01.025
- Choi, D. S., Park, S. H., Choi, S. R., Kim, J. S., & Chun, H. H. (2015). The combined effects of ultraviolet-C irradiation and modified atmosphere packaging for inactivating Salmonella enterica serovar Typhimurium and extending the shelf life of cherry tomatoes during cold storage. Food Packaging Shelf Life, 3, 19–30.10.1016/j.fpsl.2014.10.005
- Dimitrijevic, M., Anderson, R. C., Callaway, T. R., Jung, Y. S., Harvey, R. B., Ricke, S. C., & Nisbet, D. J. (2006). Inhibitory effect of select nitrocompounds on growth and survivability of listeria monocytogenes in vitro. Journal of Food Protection, 69, 1061–1065.10.4315/0362-028X-69.5.1061
- Djadouni, F., & Kihal, M. (2012). Antimicrobial activity of lactic acid bacteria and the spectrum of their biopeptides against spoiling germs in foods. Brazilian Archives of Biology and Technology, 55, 435–444.10.1590/S1516-89132012000300015
- Fröder, H., Martins, C. G., de Souza, K. L., Landgraf, M., Franco, B. D., & Destro, M. T. (2007). Minimally processed vegetable salads: microbial quality evaluation. Journal of Food Protection, 70, 1277–1280.10.4315/0362-028X-70.5.1277
- Gerez, C. L., Torino, M. I., Rollán, G., & Font de Valdez, G. F. (2009). Prevention of bread mould spoilage by using lactic acid bacteria with antifungal properties. Food Control, 20, 144–148.10.1016/j.foodcont.2008.03.005
- Gil, M. I., Selma, M. V., López-Gálvez, F., & Allende, A. (2009). Fresh-cut product sanitation and wash water disinfection: Problems and solutions. International Journal of Food Microbiology, 134, 37–45.10.1016/j.ijfoodmicro.2009.05.021
- Gwak, H. J., Lee, J. H., Kim, T. W., Choi, H. J., Jang, J. Y., Lee, S. I., & Park, H. W. (2015). Protective effect of soy powder and microencapsulation on freeze-dried Lactobacillus brevis WK12 and Lactococcus lactis WK11 during storage. Food Science and Biotechnology, 24, 2155–2160.10.1007/s10068-015-0287-5
- Jayaprakasha, G. K., Selvi, T., & Sakariah, K. K. (2003). Antibacterial and antioxidant activities of grape (Vitis vinifera) seed extracts. Food Research International, 36, 117–122.10.1016/S0963-9969(02)00116-3
- Jo, M. Y., Kim, J. H., Lim, J. H., Kang, M. Y., Koh, H. B., Park, Y. H., Yoon, D. Y., Chae, J. S., Eo, S. K., & Lee, J. H. (2004). Prevalence and characteristics of Escherichia coli O157 from major food animals in Korea. International Journal of Food Microbiology, 95, 41–49.10.1016/j.ijfoodmicro.2004.01.016
- Koo, O. K., Kim, S. M., & Kang, S. H. (2015). Antimicrobial potential of Leuconostoc species against E. coli O157:H7 in ground meat. Journal of the Korean Society for Applied Biological Chemistry, 58, 831–838.10.1007/s13765-015-0112-0
- Lee, S. H., & Chang, H. C. (2016). Isolation of antifungal activity of Leuconostoc mesenteroides TA from kimchi and characterization of its antifungal compounds. Food Science and Biotechnology, 25, 213–219.10.1007/s10068-016-0032-8
- Meireles, A., Giaouris, E., & Simões, M. (2016). Alternative disinfection methods to chlorine for use in the fresh-cut industry. Food Research International, 82, 71–85.10.1016/j.foodres.2016.01.021
- Oh, M. H., Park, B. Y., Jo, H. J., Lee, S. M., Lee, H. Y., Choi, K. H., & Yoon, Y. H. (2014). Use of antimicrobial food additives as potential dipping solutions to control pseudomonas spp. Contamination in the frankfurters and ham. Korean Journal for Food Science of Animal Resources, 34, 591–596.10.5851/kosfa.2014.34.5.591
- Özcelik, S., Kuley, E., & Özogul, F. (2016). Formation of lactic, acetic, succinic, propionic, formic and butyric acid by lactic acid bacteria. Food Science and Technology Research, 73, 536–542.
- Pujato, S. A., del L Quiberoni, A., Candioti, M. C., Reinheimer, J. A., & Guglielmotti, D. M. (2014). Leuconostoc citreum MB1 as biocontrol agent of Listeria monocytogenes in milk. Journal of Dairy Research, 81, 137–145.10.1017/S002202991300068X
- Rattanachaikunsopon, P., & Phumkhachorn, P. (2010). Lactic acid bacteria: Their antimicrobial compounds and their uses in food production. Annals of Biological Research, 1, 218–228.
- Salleh, F., Lani, M. N., & Ismail, N. (2014). Antimicrobial activity of cell free supernatant of lactic acid bacteria isolated from fermented durian flesh against multiple antibiotic resistance’s Salmonella associated with food poisoning cases in Malaysia. IOSR Journal of Pharmacy and Biological Sciences, 9, 60–65.10.9790/3008
- Santos, M. I. S., Martins, S. R., Pedroso, L., Sousa, I., & Ferreira, M. A. S. S. (2015). Potential bio-activity of whey fermented extract as sanitizer of organic grown lettuce. Food Control, 50, 477–481.10.1016/j.foodcont.2014.09.032
- Shah, M. S., Eppinger, M., Ahmed, S., Shah, A. A., Hameed, A., & Hasan, F. (2015). Multidrug-resistant diarrheagenic E. coli pathotypes are associated with ready-to-eat salad and vegetables in Pakistan. Journal of the Korean Society for Applied Biological Chemistry, 58, 267–273.10.1007/s13765-015-0019-9
- Siroli, L., Patrignani, F., Serrazanetti, D. I., Tabanelli, G., Montanari, C., & Gardini, F. (2015). Lactic acid bacteria and natural antimicrobials to improve the safety and shelf-life of minimally processed sliced apples and lamb's lettuce. Food Microbiology, 47, 74–84.10.1016/j.fm.2014.11.008
- Tejero-Sariñena, S., Barlow, J., Costabile, A., Gibson, G. R., & Rowland, I. (2012). In vitro evaluation of the antimicrobial activity of a range of probiotics against pathogens: Evidence for the effects of organic acids. Anaerobe, 18, 530–538.10.1016/j.anaerobe.2012.08.004
- Xu, W., Qu, W., Huang, K., Guo, F., Yang, J., Zhao, H., & Luo, Y. (2007). Antibacterial effect of grapefruit seed extract on food-borne pathogens and its application in the preservation of minimally processed vegetables. Postharvest Biology and Technology, 45, 126–133.10.1016/j.postharvbio.2006.11.019
- Zhang, L., Critzer, F., Davidson, P. M., & Zhong, Q. (2014). Formulating essential oil microemulsions as washing solutions for organic fresh produce production. Food Chemistry, 165, 113–118.10.1016/j.foodchem.2014.05.115
- Zheng, X., Wang, W., Piao, H., Xu, W., Shi, H., & Zhao, C. (2013). The genus Gnaphalium L. (Compositae): Phytochemical and pharmacological characteristics. Molecules, 18, 8298–8318.10.3390/molecules18078298