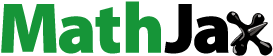
Abstract
Wheat flour was partially substituted with sorghum (SF), oat (OF), or corn (CF) flours at the 2.5, 5, 7.5, and 10% levels. Particle size (D), water-holding capacity, pasting properties with the Rapid Visco Analyser (RVA), dough volume (DV) and the physical properties of dough (Kieffer Extensibility Rig) were determined. Significant changes in the pasting properties of the substituted flours according to degree of substitution were observed. The RVA pasting parameters increased with the degree of substitution in all cases. The dough maximum force in tension (Rmax) and deformation work were also affected by degree of substitution and cereal type. The DV of wheat flour substituted with OF showed no significant differences from WF, but significant differences were observed when SF or CF were used. These modifications were related to changes in the composition of the dry matter resulting from differences in the properties of substitution cereal components (carbohydrates, proteins, ash and lipids). Hence low substitutions could considerably reduce the cost of raw materials and could nutritionally improve products with cereal blends. However, it is necessary to evaluate any changes in bread characteristics.
Public Interest Statement
The main idea is to show from the physical chemical and rheological point of view how important functional properties of wheat flour can be affected when it is replaced by low levels of sorghum, oats or corn flour. The above focused on the importance that these changes could have in an industrial process. On the other hand, the dependence of wheat flour is currently very large, which is why it is proposed to use different cereals to wheat that could be integrated into a commercial baking process by lowering the costs of production to be used in the substitution of wheat flour cereals of lower cost to wheat.
Competing Interests
The authors declare no competing interest.
1. Introduction
The development of technologies for processing grains that facilitate the production of a large amount of products with beneficial health properties has led to the increase of wheat flour products substituted by other flours. These can provide components such as fibre, proteins, natural oils, and the derivatives from transforming the bioactive components of these whole grains. Among flours employed in substituting wheat for improving nutritional and organoleptic characteristics, and obtaining even lower production costs, are those from sorghum, millet, corn, oat, rye, triticale, rice, amaranth, and barley (Chavan, Kadam, & Ramka Reddy, Citation1993). Another very important point to consider from an economic point of view is that the price of wheat grain constantly fluctuates on international markets. Therefore, it is very important to consider savings that can benefit flour producers or manufacturers of products based on wheat flours by replacing part of them with other flour options which, in addition to the nutritional benefits they offer, involve an end product with good organoleptic characteristics that consumers readily accept. This in turn lowers the costs of raw materials that to produce composite flours. In this study sorghum, oat, or corn flour which are currently cheaper than wheat grain (SAGARPA, Citation2014) were used. The introduction of other flour types involves changes in bread formation properties as a result of composite flours. It is important to consider the contribution of the carbohydrates, proteins, and lipids from the involved grains. These components have vital effects on dough’s rheological and physical properties. Water absorption capacity determines the quality of flour and its ability to form a viscoelastic dough, which are important in the food industry because they affect their functional properties and the quality of final products (bread, biscuits, pastas, etc.) (Berton, Scher, Villieras, & Hardy, Citation2002). Among carbohydrates, starch has a considerable effect on flour viscosity. However depending on the specific contribution of each flour, proteins and lipids also affect to this parameter. The rapid visco analyser (RVA) is a very effective tool for measuring the viscosity properties of cooked starch and flour, and for relating to flour functionality. Another property that results from these components, such as proteins, is wheat flour’s ability to form the viscoelastic network (gliadins and glutenins), which are responsible for gas retention during fermentation and for loaves rising during baking (Marchetti, Cardós, Campaña, & Ferrero, Citation2012). The aim of this study was to evaluate the impact of using low degrees of substitution of wheat flour with sorghum (SF), oat (OF), or corn (CF) flours on the physicochemical properties of composite flours.
2. Materials and methods
2.1. Materials
The commercial WF used was obtained from a local producer (Molí de Picó-Harinas Segura S.L. Valencia, Spain). This company provided the alveographic parameters: P = 94 ± 2 (maximum pressure (mm)), L = 128 ± 5 (extensibility (mm)), W = 392 ± 11 (strength (J-4)), and P/L of 0.73. OF and CF were obtained locally (La Carabasseta, Valencia, Spain). SF was purchased from a commercial bakery (Integral Food S.A. Barcelona, Spain). Sunflower oil (maximum acidity 0.2°; Koipesol Semillas, S.L., Spain), pressed yeast (Saccharomyces cerevisiae, Lesafre Ibérica, S.A., Spain), white sugar (≥ 99.8% of saccharose, Azucarera Ebro, S.L., Spain) and NaCl (refined marine salt ≥97% NaCl Salinera Española S.A., Spain) were purchased in a local market.
2.2. Preparation of composite flours
The composite flours were obtained by mixing wheat flours with SF, OF, or CF at ratios of 97.5/2.5, 95/5, 92.5/7.5, and 90/10, respectively.
2.3. Compositional analysis of flours
The percentages of moisture, protein, lipids, and ash of the wheat, oat, corn, and sorghum flours were determined in triplicate according to methods 44–40, 46–13, 30–20 and 08–01 (AACC, Citation1995), respectively. Carbohydrate content was calculated by differences. From the compositional data dry matter (DM) was calculated as total weight without moisture. In order to take into account the influence of proteins, carbohydrates (including both soluble as non-soluble fibres), and lipids from wheat and from the substitutes, their ratios (Pr, CHr and Lr, respectively), and per blend, were calculated as follows in Equation (1):(1)
(1)
where “component” refers to proteins, carbohydrates or lipids, and g is the wet basis.
2.4. Particle size of flours
Particle size (D) of flours was measured by laser scattering in a Mastersizer 2000 (Malvern, Instruments, UK) equipped with a Scirocco dry powder unit. The obtained results were the diameters of the equivalent spheres expressed as volume. The instrument provided the volume weighted mean D [4,3] expressed in micrometres (μm).
2.5. Water-holding capacity
The WHC test was conducted according to method 56–11 (AACC, Citation2000). Flour samples (5 g) were weighed in centrifuge tubes (50 mL) with screw caps. Then 25.0 mL of water were added and the mixture was shaken vigorously for 5 s to suspend flour. Samples were kept for 20 min on a vortex mixer with intermittent agitation for 5, 10, 15 and 20 min, followed by 15 min of centrifugation (Centrifuge Beckman Coulter Allegra X-30R, Brea, California, USA) at 1,000 ×g at room temperature. The supernatant was discarded and the obtained wet pellet was allowed to decant for 10 min and was weighed. WHC was calculated as described by Haynes, Bettge, and Slade (Citation2009).
2.6. Pasting properties using the RVA
The viscosity profile of the composite flours was obtained by method 76–21 (AACC, Citation2000). The RVA-S4 viscometer (Rapid Visco Analyser Super 4, Newport Scientific, Warriewood, Australia) was used for this study. The samples were analysed on the basis of 3.5 ± 0.01 g of sample adjusted to 14% moisture. The amount of water incorporated was 25 ± 0.01 g. The test profile had a starting temperature of 50°C and 960 RPM, and slowed to 160 RPM at 10 s. At minute 1 of the test, the temperature was maintained at 50°C, increased to 95°C for 4.42 min, and maintained until 7.42 min. At minute 11, the temperature dropped to 50°C and it was kept at 50°C for 2 more minutes until the test ended at 13 min. The obtained parameters were pasting temperature (PT), peak viscosity (PV), trough viscosity (TV), breakdown viscosity (BV), final viscosity (FV), and setback viscosity (SV). The viscosity values were expressed in centipoise (cP).
2.7. Dough preparation
The dough formulation was the following: 57.24% flour, 33.54% water, 1.97% refined sunflower oil, 2.23% commercial pressed yeast, 3.94% white sugar and 1.06% NaCl. Dough was prepared by mixing all the ingredients in a food mixer (Thermomix® TM31, Vorwerk, Germany) according to the following procedure: in the first phase, liquid components (water and oil), sugar and NaCl were mixed for 2 min at 37°C. The pressed yeast was added in the next phase and mixed at the same temperature for 30 s. Finally the flours, along with all the other ingredients, were added and mixed using a default bread dough mixing programme. The programme system focused on mixing ingredients with the mixer helices taking random turns in both directions (550 RPM) to obtain a homogeneous dough. This process was applied for 4.5 min at 37°C.
2.8. Dough volume measurements
Seventy grams of dough were placed into a transparent cylindrical graduate glass container (Soleimani Pour-Damanab, Jafary, & Rafiee, Citation2011, 5.5 cm diameter, 15.5 cm high), which was placed inside the proofing chamber (KBF720 Binder, Tuttlingen, Germany) at controlled temperature and relative humidity (40°C and 90% RH). For this measurement, the initial dough height and that obtained after 1 h of fermentation were recorded. The calculation of volume (DV) was based on the formula for a cylinder volume.
2.9. Physical dough properties
Uniaxial extension was performed on dough with a TA.XT2 Texture Analyser (Stable Micro Systems, Godalming, Surrey, England) with the SMS/Kieffer dough and gluten extensibility rig. Dough was placed in a greased Teflon mould, compressed with the top Teflon plate, and was left to stand for 45 min at 30°C (Ktenioudaki, O’Shea, & Gallagher, Citation2013). Maximum force in tension (Rmax) and distance to rupture (Ext) were considered indications of dough resistance and extensibility, respectively. The area under the curve or deformation work (DFW) was also recorded. The test speed was 3.3 mm/s.
2.10. Statistical analysis
A one-way analysis of variance (ANOVA) was used to evaluate the effect of substitution level on the pasting properties measured by RVA (PT, PV, TV, BV, FV, and SV), WHC, rheological properties (Rmax, Ext, and DFW), DV, and D. For those cases where the effect was significant (p-value < 0.05), the means were compared by Fisher’s least significant difference (LSD) procedure. In order to obtain an overview of the data-set, all the parameters and compositional data (DM, Pr, CHr, and Lr) were also analysed by the multivariate statistical procedure, principal component analysis (PCA). Here the number of PCs was selected after taking into account the change of tendency in the scree plot. The accumulated percentage of explained variability was higher than 75%. Procedures were performed with PLS Toolbox 6.3 (Eigenvector Research Inc., Wenatchee, Washington, USA), a toolbox extension in the Matlab 7.6 computational environment (The Mathworks, Natick, Massachusetts, USA).
3. Results and discussion
3.1. Compositional analysis of flours
The moisture, protein, lipids, ash, and carbohydrate contents of the flour samples are shown in Table . Significant differences (p ≤ 0.05) among the flours were found. WF obtained the highest moisture value (13.09% ± 0.01), while OF gave the lowest one (10.34% ± 0.05). The opposite happened for lipids content (WF = 1% ± 0.02 and OF = 8% ± 0.19), and an inverse relationship between water content and lipids was observed. Similar lipid values for OF have been obtained by others authors (Hu, Zheng, Li, Xu, & Zhao, Citation2014). WF also had the highest protein content (12.7% ± 0.28), while the lowest value corresponded to CF (8.3% ± 0.24). The values for SF and OF were 10% ± 0.24 and 11% ± 0.25, respectively. The highest ash value was obtained by SF (1.74% ± 0.17). It is important to keep in mind this result as well as the highest quantity of lipids compared to the other flours. When both partially replace WF, the compositional characteristics of the obtained blend can strongly affect bread processing. The lowest carbohydrate content was observed in OF (69.74%); while WF (72.89%), SF (73.97%), and CF (76.63%) had higher values.
Table 1. Compositional analysis of the wheat, sorghum, oat, and corn flours
3.2. Particle size of flours
The particle size (Figure (A)) of flour, expressed as the mean of volume weighted (D [4,3] (μm)), was bigger for all the wheat flour substituted (WFS) than WF (99.42 μm ± 0.88). For all the composite flours (WFS), the highest values were obtained at the 10% degree of substitution (WSF:OF = 114.21 μm ± 1.56, and CF 111.87 μm ± 0.66), and even the increase in WFS with SF at 10% was slight (106.48 μm ± 0.79). Gomez, Rooney, Waniska, and Pflugfelder (Citation1987) observed a dough network interrupted by large particles, while smaller particles were responsible for most of the dough’s water absorption, viscosity, plasticity and softness. Reducing particle size can have substantial effects on the physicochemical changes, generated by an increased contact surface of particles, and this can affect food quality. The particle size of WF depends on the hardness and class, type of grinder and grinding time of wheat (Hareland, Citation1994). The trend was the bigger the particle size, the higher the degree of substitution, which became more evident when OF or CF was used.
3.3. Water-holding capacity
Water-holding capacity (WHC) refers to the ability of food or its components to hold water under certain conditions (Labuza & Busk, Citation1979). In flour, this property is used to determine its quality and ability to hold water, which is essential in the food industry since it determines dough’s functional properties. Figure shows the relationship between particle size and WHC. With this measurement, WFS with SF at 7.5 (69.53% ± 0.39) and 10% (69.42% ± 0.01) and OF at 2.5 (69.25% ± 0.49) and 5% (69.06% ± 0.21) of substitution showed no significant differences compared to WF (69.15% ± 0.6). The highest value was recorded when WF was substituted for SF at 2.5 (70.61% ± 0.14) and 5% (71.54% ± 0.12). This test offers a prediction of the functionality of flour, its ability to absorb water during the mixing process, and its ability to release that water during the baking process. WHC was affected by flour refinement as well as particle size. This means increased damaged starch, which affects flour quality. Berton et al. (Citation2002) concluded that WHC depends on the level of grinding, the measurement method used, and damaged starch content which is a major factor for increasing flour’s hydration capacity. The obtained results show an interval among flours which ranged from 65.77 to 71.54%. These results were similar to those obtained by Ram, Dawar, Singh, and Shoran (Citation2005), who worked on WHC of wheat flours and obtained values ranging from 53.4 to 70.6%. WHC lowered with increased particle size, except when WF was substituted for SF at the 2.5 (70.61% ± 0.14) and 5 (71.54% ± 0.12) percentages. WHC was also affected by degree of substitution, which became more evident when WF was substituted for CF at the 7.5 (65.95% ± 0.01) and 10 (65.77% ± 1.01) percentages.
3.4. Pasting properties using the RVA
The RVA is usually related to starch behaviour, although there are other important components involved in this test, such as proteins and lipids. These components interact with starch and affect the viscosity measurement in RVA (Batey, Citation2009). The viscosity profiles of WFS substituted for SF, OF or CF using RVA are illustrated in Figure . No significant difference (p ≤ 0.05) between the PT (Figure (A)) of WFS at the 2.5% substitution and WF (68.95°C ± 0.64) was found. Possibly the low degree of substitution did not alter the components in the system, nor the viscosity profiles in the components state. When substitution rose to 5%, significant increases in the PT in WFS with SF (87.28°C ± 0.04) and CF (85.95°C ± 0.57) were observed. Flours with OF underwent such an increase when the degree of substitution was 7.5%, and when the PT value equalled 84.73°C ± 0.04. The high fat content of oats could form a barrier against water to penetrate into starch granules to become fully gelatinised. At the 7.5 and 10% substitutions, some differences among CFs were observed, but were smaller. Modifications may be due to the change in the content of amylose, lipids, and proteins of WF (Sun, Han, Wang, & Xiong, Citation2014), and also to the particular contribution of these components for cereals in the WF substitution. Although there were slight differences in the PV values (Figure (B)), no significant differences were obtained among flours, except when WF was substituted for SF at the 2.5% substitution (2,164 cP ± 24.95). PV is associated with the starch swelling by the combination of starch granule rupturing and the disruption rate of granules. Diminished starch granule swelling is indicative of both low peaks of viscosity and reduced starch granule rupturing (Ktenioudaki et al., Citation2013). TV (Figure (C)) was lower in WFS with SF at 2.5% (1,308 cP ± 48.08), but was higher in WFS with OF at 10% (1,476 cP ± 36.77), where no significant differences in the latter for WF (1,421 cP ± 4.95) were observed. This parameter refers to minimum viscosity after PV. The obtained results indicated a significant direct relationship between PV and TV, where increasing PV also increased TV. The studies by Zaidul, Norulaini, Omar, Yamauchi, and Noda (Citation2007) observed that TV correlated directly with PV. BV is considered a measure of the degree of disintegration of granules or paste stability (Dengate, Citation1984; Newport Scientific, Citation1995). BV (Figure (D)) was lower in WFS with OF at both 2.5% (798 cP ± 11.31) and at 10% (790 cP ± 24.75), with significant differences found for WFS with SF or CF in four different proportions. However, these differences were not seen when compared with WF (823.5 cP ± 10.61). The BV values obtained from WFS with OF were the lowest; the highest TV values were obtained when this cereal was used in the substitution, and a relationship with PV was observed. Accordingly, similar behaviour between SF and CF was observed when both were used in the substitution of Ragaee and Aal (Citation2006) found that high BV values were associated with high PV values, which is related to the degree of starch swelling during heating. For FV (Figure (E)), the degree of the substitution effect was more noticeable when WF was substituted for SF. Significant increases in WFS with SF were observed, the substitution percentage increased, the above was more evident for SF at 10% (3,029.5 cP ± 47.38). The same trend was observed when WF was substituted for OF and CF. Changes in FV resulted from the gel formation during cooling; this happens because of a re-association between the starch molecules, primarily amylose, extracted previously from starch granules during the mixing process. However, the temperature in this stage plays a very important role. At low temperatures, starch chains intersect each other to form a gel that helps increase viscosity. Finally, SV (Figure (F)) considerably increased in WFS with SF, behaved similarly to that observed in FV, and this increase was greater the higher the substitution percentage. SV is obtained by the difference between values FV and TV. Therefore, it presents a relevant relationship with FV. A higher ash value was recorded for SF. This is indicative of low grade refining, and consequently less damage to its components, including the amylose of good cross-linking properties to increase the viscosity in this stage together with FV. Zaidul, Karim, Manan, Norulaini, and Omar (Citation2003) reported that SV reflects the starch retrogradation tendency, which inhibits minimum viscosity increasing upon cooling. Zaidul et al. (Citation2007) also found that substituting wheat flour with potato starch obtained significant changes in PV and SV, caused by the protein and lipids from WF.
3.5. Measurement of dough volume
Dough fermentation of WF is one of the most important processes in the bread-making industry that may be affected by protein content and its quality, and also by the formation of a matrix gluten-starch as a cell membrane capable of stabilising gas expanding cells (Bloksma, Citation1990; Hoseney, Citation1992). The values obtained within 1 h of fermentation (Figure (B)) showed no significant differences when WF was substituted for OF at all the degrees of substitution, and was even similar to the WF values (136.32 cm3 ± 4.62). This is possible because the balance of the protein fraction in oats, albumins (10–20%), globulins (12–55%), prolamins (12–14%) and glutelins (23–54%) (Haard, Citation1999), which generate the holding capacity of gas produced during fermentation, is similar to that in dough produced employing WF. WFS with the SF and CF dough volumes (DV) displayed a slight tendency to lower when substitution increased, and was more evident for the 7.5 and 10% degrees of substitution.
3.6. Physical dough properties
The Rmax of dough obtained from the mixtures of WF with SF, OF and CF at any percentage (2.5, 5, 7.5 and 10%) (Table ) obtained significantly lower values than WF (0.69 N ± 0.01). Indeed the WFS values with SF at 7.5% and 10% (0.59 N ± 0.06 and 0.61 N ± 0.06, respectively) and OF at 2.5% (0.6 N ± 0.01) of substitution came closest to WF. For the three substituents, CF obtained statistically lower values, regardless of the degree of substitution. Regarding Ext (Table ), which expresses distance to rupture, no significant differences for WFS with SF at 5% (78.1 mm ± 8.49) and 7.5% (77.7 mm ± 5.95), WFS with OF at 2.5% (72.1 mm ± 2.33) and 5% (78.7 mm ± 1.73), and WFS with CF at 2.5% (73.42 mm ± 12.9) were seen compared to WF (78.15 mm ± 3.76). The lowest values went to this parameter, and for Rmax when WF was substituted for CF at 5% (63.8 mm ± 5.29). However, the trend found was a lowering % of substitution. This behaviour has been reported by Rieder, Holtekjølen, Sahlstrøm, and Moldestad (Citation2012), who worked on the dough rheological quality of WF substituted for barley and oat flour. These authors observed how Rmax and Ext lowered by wheat gluten dilution, and how fibre content increased, which can break the gluten network during mixing. The DFW values (Table ) all significantly differed from WF (34.23 N-mm ± 0.7). The degree of substitution and cereal type strongly affected this result. The lowest values were recorded for WFS with CF at its four percentages (11.82–14.16 N-mm), and no significant difference was recorded among them, which also occurred with the Rmax values.
Table 2. Dry matter, ratios of proteins, carbohydrates, lipids and physical properties of WF and each WFS
3.7. Overview study of the data-set
In order to obtain an overview of the data-set, all the parameters evaluated and compositional data (DM, Pr, CHr, and Lr) were analysed employing the multivariate statistical procedure, PCA. The Pr, CHr and Lr ratios (Table ) were used to consider the interactions among components. Figure shows the scores and loadings of each PCA result. The analysis resulted in three PCs, which explained 84.11% of total variability. PC1 (Figure (A)), with 36.21% of total variability, was related to the substitution degree of all the WFS, PC2 (Figure (B)) and PC3 (Figure (C)), with a variability of 21.19 and 20.41%, respectively, in relation to flour type. The loadings of PC1 (Figure (A)) showed that all the RVA variables presented positive contributions, principally PT, followed by PV and FV. Likewise, the compositional variables, such as the component ratios (CHr, Pr and Lr), DM and particle size (D), gave positive contributions, where CHr, Pr and D obtained the maximum. The variables related to physical properties, DV and WHC, gave negative contributions. According to these results, the changes in D, and also in Pr and CHr, with an increasing degree of substitution, could be related directly to the changes in PT, and inversely with DV, WHC and the physical properties. As previously discussed, particle size had a significant effect on WF functionality (Protonotariou, Drakos, Evageliou, Ritzoulis, & Mandala, Citation2014). Similarly, the degree of substitution of WF brought about a reduction in DV, WHC and the physical properties, possibly due to the lower content of the protein fractions involved directly in gluten formation. In fact, the weight of the loadings of Pr and CHr were considerably high. This indicates the strong influence of the compositional variables of substitution cereals on PT, DV, the physical properties and WHC. PC2 (Figure (B)) clusters WF and WFS with OF in the positive zone, while SF or CF are clustered in the negative zone. WFS with OF presented a crescent tendency as the degree of substitution increased. The variables with more contributions to PC2 were TV, DV, Lr and DM, while BV was much more negative. The clearest results were the influence on DV, where no differences between OF and WF were seen (Figure (B)). This effect was given mainly by its differences form DM compared to the other substitution cereals, especially amount of lipids and polymeric fibre components, such as β-glucans. In their aqueous phase, these components can increase dough viscosity which, in turn, can stabilise gas cells, which may be the reason for a higher bread volume (Rieder et al., Citation2012). These components can also easily form complexes with the short amylose molecules present in oat starch. So the highly branched amylopectin could be more active during gel structure formation (Berski, Krystyjan, Buksa, Zięć, & Gambuś, Citation2014). Generally speaking, the variables with high loadings on PC2 (Figure (B)) could be understudied as they were more influenced by the changes caused by WFS with OF in the model, for which a marked separation across its axis was seen. For PC3 (Figure (C)), the scores of WF and WFS with SF shifted towards the positive zone of the axis, following an increase in the degree of substitution, except when 2.5% was used, which appeared in the negative zone, but close to WF. WFS with OF was placed around the 0 zone of axis, and not even the degree of substitution had any influence. WFS with CF was placed completely in the negative zone. The variables with the highest loading weights were DFW, Rmax, FV and WHC, and they were all in the positive zone. Thus the physical properties of WFS with SF increased individually, particularly DFW, Rmax, WHC and FV, together with the degree of substitution. This phenomenon can be attributed to the increase in Rmax, possibly related to sorghum protein (kafirins), which is able to generate stiff dough, caused by the cross-linking of kafirin monomers that are capable of forming disulphide bonds since they contain more cysteine residues (Oom, Pettersson, Taylor, & Stading, Citation2008). In summary, the main affected variables were those that were related to pasting properties because most showed high loadings for the three PCs. In other words, their weighting on the total variability produced by both degree of substitution and cereal type was high. DV, WHC, and the physical properties ranked second. Finally, the observed variations in substituted wheat flour behaviour should be interpreted as a result of the features of the cereal used as a substitute. These characteristics are susceptible to application with the aim of modifying WF behaviour, improving processes, or changing product features to reduce costs and enhance the nutritional profile.
4. Conclusions
Cereal type and degree of substitution had a significant effect on flour properties. Even though the degree of substitution was low, this factor was related to the increase in PT, the lower WHC and DV values, which also had strong effects on the physical properties (all related to particle size, carbohydrate and protein ratios). Changes in components’ individual contribution to the flours involved in substitution affected the behaviour of blends. OF, which contributed with the highest level of lipids, generated less loss of DV, PT and BV, but greater loss of TV. SF had a considerable influence on the increase of PT, SV, WHC, FV and the physical properties. CF compared to SF had an inverse effect as it lowered the physical properties, WHC, and FV. The information obtained herein can be useful for taking into account the adjustment of processing parameters when a low degree of substitution is intended to be used to develop new products or to reduce the costs of raw materials.
Additional information
Funding
Notes on contributors
Francisco Vásquez
The work mainly focuses on basic research and technological development in the area of cereals. Basic research projects cover aspects related to the quality, handling, storage and processing of the main grains. In terms of technological development we work on ingredients functionality and the development of new products, as well as the implementation of new processes for the transformation of cereals. The use of sub-products of the food industry can be a good alternative to incorporate them into industrial processes with the aim of reducing costs in the finished product and in many cases improvements from the nutritional point of view. On the other hand, various analytical and technical assistance services are provided to the industrial sectors related to grain processing.
References
- AACC. (1995). Approved methods of the American Association of Cereal Chemists (9th ed.). St. Paul, MN: Author.
- AACC. (2000). Approved methods of American Association of Cereal Chemists. Methods 56–11, 76–21 (10th ed.). St. Paul, MN: Author.
- Batey, I. L. (2009). Interpretation of RVA curves. In G. B. Crosbie & A. S. Ross (Eds.), The RVA handbook (pp. 19–30). AACC International.
- Berski, W., Krystyjan, M., Buksa, K., Zięć, G., & Gambuś, H. (2014). Chemical, physical and rheological properties of oat flour affected by the isolation of beta-glucan preparation. Journal of Cereal Science, 60, 533–539.10.1016/j.jcs.2014.09.001
- Berton, B., Scher, J., Villieras, F., & Hardy, J. (2002). Measurement of hydration capacity of wheat flour: Influence of composition and physical characteristics. Powder Technology, 128, 326–331.10.1016/S0032-5910(02)00168-7
- Bloksma, A. H. (1990). Dough structure, rheology, and baking quality. Cereal Foods World, 35, 237–244.
- Chavan, J. K., Kadam, S. S., & Ramka Reddy, N. (1993). Nutritional enrichment of bakery products by supplementation with nonwheat flours. Critical Reviews in Food Science Nutrition, 33, 189–226.10.1080/10408399309527620
- Dengate, H. N. (1984). Swelling, pasting, and gelling of wheat starch. In Y. Pomeranz (Ed.), Advances in cereal science and technology (pp. 49–82). St. Paul, MN: American Association of Cereal Chemists.
- Gomez, M. H., Rooney, L. W., Waniska, R. D., & Pflugfelder, R. L. (1987). Dry corn masa flours for tortilla and snack food production. Cereal Foods World, 32, 372.
- Haard, N. F. (1999). Cereals: Rationale for fermentation. In N. F. Haard (Ed.), Fermented cereals: A global perspective (Vol. 1, pp. 1–27). Rome: Agriculture Organization of the United Nations.
- Hareland, G. V. (1994). Evaluation of flour particle size distribution by laser diffraction, sieve analysis and near-infrared reflectance spectroscopy. Journal of Cereal Science, 20, 183–190.10.1006/jcrs.1994.1058
- Haynes, L. C., Bettge, A. D., & Slade, L. (2009). Soft wheat and flour products methods review: Solvent retention capacity equation correction. AACC International Report, 54, 174–175.
- Hoseney, R. C. (1992). Physical chemistry of bread dough. In H. G. Schwartzberg & R. W. Hartel (Eds.), Physical chemistry of foods (pp. 443–457). New York, NY: Marcel Dekker.
- Hu, X. Z., Zheng, J. M., Li, X. P., Xu, C., & Zhao, Q. (2014). Chemical composition and sensory characteristics of oat flakes: A comparative study of naked oat flakes from China and hulled oat flakes from western countries. Journal of Cereal Science, 60, 297–301.10.1016/j.jcs.2014.05.015
- Ktenioudaki, A., O’Shea, N., & Gallagher, E. (2013). Rheological properties of wheat dough supplemented with functional by-products of food processing: Brewer’s spent grain and apple pomace. Journal of Food Engineering, 116, 362–368.10.1016/j.jfoodeng.2012.12.005
- Labuza, T. P., & Busk, C. G. (1979). An analysis of the water binding in gels. Journal of Food Science, 44, 1379–1385.10.1111/jfds.1979.44.issue-5
- Marchetti, L., Cardós, M., Campaña, L., & Ferrero, C. (2012). Effect of glutens of different quality on dough characteristics and breadmaking performance. LWT-Food Science and Technology, 46, 224–231.10.1016/j.lwt.2011.10.002
- Newport Scientific. (1995). Interpretation. In Newport Scientific (Ed.), Operation manual for the series 3 Rapid Visco Analyser (pp. 25–28). Sydney: Author.
- Oom, A., Pettersson, A., Taylor, J. R. N., & Stading, M. (2008). Rheological properties of kafirin and zein prolamins. Journal of Cereal Science, 47, 109–116.10.1016/j.jcs.2007.02.005
- Protonotariou, S., Drakos, A., Evageliou, V., Ritzoulis, C., & Mandala, I. (2014). Sieving fractionation and jet mill micronization affect the functional properties of wheat flour. Journal of Food Engineering, 134, 24–29.10.1016/j.jfoodeng.2014.02.008
- Ragaee, S., & Aal, E. M. A. (2006). Pasting properties of starch and protein in selected cereals and quality of their food products. Food Chemistry, 95, 9–18.10.1016/j.foodchem.2004.12.012
- Ram, S., Dawar, V., Singh, R. P., & Shoran, J. (2005). Application of solvent retention capacity tests for the prediction of mixing properties of wheat flour. Journal of Cereal Science, 42, 261–266.10.1016/j.jcs.2005.04.005
- Rieder, A., Holtekjølen, A. K., Sahlstrøm, S., & Moldestad, A. (2012). Effect of barley and oat flour types and sourdoughs on dough rheology and bread quality of composite wheat bread. Journal of Cereal Science, 55, 44–52.10.1016/j.jcs.2011.10.003
- SAGARPA. (2014). Agencia de Servicios a la Comercialización y Desarrollo de Mercados Agropecuarios. México: ASERCA.
- Soleimani Pour-Damanab, A. R., Jafary, A., & Rafiee, Sh. (2011). Monitoring the dynamic density of dough during fermentation using digital imaging method. Journal of Food Engineering, 107, 8–13.10.1016/j.jfoodeng.2011.06.010
- Sun, Q., Han, Z., Wang, L., & Xiong, L. (2014). Physicochemical differences between sorghum starch and sorghum flour modified by heat-moisture treatment. Food Chemistry, 145, 756–764.10.1016/j.foodchem.2013.08.129
- Zaidul, I. S. M., Karim, A. A., Manan, D. M. A., Norulaini, N. A. N., & Omar, A. K. M. (2003). Gelatinization properties of sago and wheat flour mixes. ASEAN Food Journal, 12, 585–598.
- Zaidul, I. S. M., Norulaini, N. A. N., Omar, A. K. M., Yamauchi, H., & Noda, T. (2007). RVA analysis of mixtures of wheat flour and potato, sweet potato, yam, and cassava starches. Carbohydrate Polymers, 69, 784–791.10.1016/j.carbpol.2007.02.021