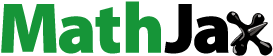
Abstract
Composting fruit and vegetable waste from grocery stores on farms is a challenge due to the large quantity of liquid that leaches out. This leachate needs to be characterized for its effects on plant growth and soil. It was acidic with high conductivity; the plant mineral nutrient contents derived from the leachate (in decreasing order) were K, N mainly as ammonium-N, Ca, Mg, Na, and P; the trace elements were quite low. The germination and rootlet growth of cress and corn increased with the dilution of the compost leachate. In greenhouse assays, the compost leachate exhibited phytotoxicity to corn when used at high application rates. The corn leaf area, shoot and root biomass, plant concentrations and uptake of N, P and K were similar among the 20 mM N-fertilized control and the 30–40 m3/ha leachate treatments. In a field study on sandy loam, corn yields and plant uptake of P and K were similar among the mineral fertilized control and split-application leachate treatments applied to meet the P or K requirements of corn. After harvest, the soil pH, conductivity, and total N, P, and K contents were not affected by the leachate treatments. These results demonstrate the opportunity of recycling plant nutrients from compost leachate of fruit and vegetable waste as fertilizer to meet crop nutrient requirements without phytotoxicity and trace elements threats.
PUBLIC INTEREST STATEMENT
This manuscript deals with the use of leachates produced during composting of fruit and vegetable waste collected from grocery stores. These leachates are rich in nutrients and bring water to plants. The plants were produced on soil farms, and their leftovers should be returned to soil. In order to foster sustainability, plant productivity and safeguard the soil resource, waste used in agriculture should improve soil properties. The waste needs to be characterized to avoid toxicity to plants and possible contamination of the soil. Furthermore, providing crops with adequate nourishment required careful quantification to adjust fertilization according to crop requirements. These leachates must be used to conserve clean water. Since fruit and vegetables contain carbon and plant nutrients and consist of more than 80% water, composting fruit and vegetable waste on farms would provide compost, as stabilized carbon to improve soil physical and biological properties, and compost leachate to fertilize and water plants, thereby closing the cycle of carbon, plant nutrients, and water.
Competing interests
The authors declare that the research was conducted without any commercial or financial relationship that could be construed as a potential conflict of interest.
1. Introduction
Managing food waste is an issue of worldwide concern. Moreover, clean and green technologies need to be optimized to prevent the dumping, landfilling, or incineration of food waste. In grocery stores, food for sale is safe for consumption and in compliance with the microbial and chemical residue surveillance program. However, in Canada, 65% of their food is lost as waste (Gooch & Felfel, Citation2014). Therefore, few grocery stores train employees to remove packing material and place trimming and rotting fruit and vegetable in a suitable container for farm composting, in which microorganisms decompose organic matter, releasing CO2, H2O, and heat and produce compost. Traditionally, for farm composting, a nitrogen (N) source is mixed with a carbon (C) source, such as manure or slurry with straw or other C sources. In this Eastern Canada study, fruit and vegetable waste (d.w.) containing 1.7 g N/kg, 0.3 g P/kg and 2.4 kg K/kg was used instead of manure or slurry, thus decreasing the hygiene risk (Sall, Antoun, Chalifour, & Beauchamp, Citation2016). The C source adjusted the C: N ratio, moisture, and porosity in the composting recipe (Sall et al., Citation2016). Some liquid, however, percolated through the compost pile and ran off. This complex wastewater leached out with minerals; suspended solids; particulates and soluble organic matter; and various oxidized, and other chemical by-products of the composting process. In the past, this leachate was lost to the soil and its environmental impact left unnoticed. While Quebec regulations now require it to be collected and adequately treated, there is only limited information about the situation caused by the cool, humid conditions under Eastern Canada conditions. For example, composting 6000 kg of fruit and vegetable waste generated about 400 L of leachate per day (Beaudette, Citation2014). To treat this leachate on farms, Beaudette (Citation2014) suggested using anaerobic and aerobic bioreactors and constructing ponds. This, however, would require new equipment, land, knowledge, and investment. In contrast, farmers wanted a low-cost approach to feed their crops and to close the nutrient loop by reusing the leachate during the summer months. For that, farmers were ready to use a common slurry spreader and follow the fertilization plan prepared by an agronomist to avoid soil P build up.
Few studies have reported compost leachate being used as a plant mineral fertilizer, while others noted phytotoxicity (Caceres, Magri, & Marfà, Citation2015; Das & Kirkland, Citation2008; Gutiérrez-Miceli, García-Gómez, Oliva-Llaven, Montes-Molina, & Dendooven, Citation2017; Jarecki, Chong, & Voroney, Citation2012; Romero, Ramos, Costa, & Márquez, Citation2013; Savage & Tyrrel, Citation2005; Zhou, Wang, & Zhang, Citation2010). Indeed, the recycling of compost leachate needs more research if the cycle of plant nutrients is to be closed without phytotoxic effects. Therefore, the physicochemical composition and effects of the leachate produced by fruit and vegetable waste compost were investigated. First, the plant mineral nutrients, including the trace elements, were determined. Then, under controlled environmental conditions, the phytotoxicity of leachate for cress and corn germination, and corn grown in pots were studied. Finally, the effects of leachate on corn yield and soil accumulation of plant nutrients were investigated in small field plots.
2. Materials and methods
2.1. Leachate physicochemical analyses
The sorted fruit and vegetable waste mixed with straw or wood waste was composted on a horticultural farm close to Quebec City in Eastern Canada (46°42ʹ09.8ʹ’N; 71°37ʹ25.2ʹ’W). This farm composted less than 150 m3/year and was equipped with forced aeration composting cells protected by a roof and concrete walls to limit the percolation of rain and melting snow through the material (Sall et al., Citation2016). Some liquid by-products were drained during the composting process and collected in a 14 m3 concrete tank. For two years, the leachates were sampled in June, July, and August with a 250 mL auger at different depths to fill a 2 L polyethylene bottle. The samples were transported to the laboratory and kept at 4°C, analyzed, and subsamples frozen. Leachate samples were analyzed for pH and electrical conductivity with a pH and conductivity meters, and ammonium-N (NH4+-N), nitrate-N (NO3–-N), orthophosphate (PO4−3-P), and sulfate (SO4−2-S) with a high-performance liquid chromatograph equipped with a conductivity detector and an IC-Pak anion column (Waters 431, Waters Corporation, Milford, MA). For total N analyses, samples were mineralized by acid digestion with H2SO4-H2SeO3-H2O2 (KJELDATHERM block digestion unit (Isaac & Johnson, Citation1976)) before their quantification by colorimetry (Nkonge & Ballance, Citation1982). Samples were acid extracted with HNO3-HClO4 before determination of the total P by colorimetry (Tandon, Cescas, & Tyner, Citation1968); K, Ca, Mg, Na, Cu, and Zn by flame atomic absorption spectrometry (AAS, Model 3300, Perkins Elmer, Woodbridge, ON); and As, Cd, Co, Cr, Hg, Mo, Ni, Pb, Se, and Zn by inductive coupled plasma spectrometry (CPVQ, Citation1997).
2.2. Germination assay
Seven different treatments were tested, including leachate (L; 100L:0W); diluted leachate solutions made of 75L:25W, 50L:50W, 25L:75W, 10L:90W, and 1L:99W; and a distilled water control (W, pH 7; 0L:100W). Cress (Lepidium sativum) and sweet corn (Zea mays cv. Luscious) were tested (Machrafi, Prevost, & Beauchamp, Citation2006). Ten cress seeds per Petri dishes were sown on germination papers filled with 5 mL of one of the above solutions. However, for corn, only eight seeds were sown per Petri dishes. The dishes were incubated in the dark at 23°C for three days for cress and five days for corn. Germination and rootlet growth were measured and the germination index (GI) calculated. The GI was calculated relative to a distilled water control using this formula:
The seven treatments were replicated three times in a randomized complete block design (RCBD). All statistical analyses were conducted by using Proc Mixed procedure of SAS 9.3 (SAS, Citation2012). The variance homogeneity was verified by a graphical analysis of the residuals and no transformation was necessary. The significant difference between means was established using the LSD test at p < 0.05.
2.3. Greenhouse assay
Under greenhouse conditions, each 3.7 L plastic pot filled with vermiculite:Turface (1:1; v/v) was sown with two seeds of sweet corn (cv. Providence). Turface MVP is a calcined clay granule (Profile Products LLC., Buffalo Grove, Ill). After emergence, seedlings were thinned to one plant per pot. For three weeks, all pots were watered with tap water once a week and three times a week with 200 mL of a nutrient solution containing KNO3 (Chalifour & Nelson, Citation1988). All pots were watered with a 2 mM N nutrient solution, referred to as low-N control (a level representative of unfertilized field-plot control; CK-), except those watered with a 20 mM N nutrient solution, referred to as high-N control (a level representative of fertilized field-plot control; CK+). Besides the low—and high-N control treatments, seven leachate treatments—10, 20, 30, 40, 50, 60, and 80 m3/ha—were applied once at the stage of 3–4 leaves. The leachate was applied at 10 m3/ha (50 ml/pot with 66 mg N/L, 15 mg P/L, 155 mg K/L), 20 m3/ha (100 ml/pot with 133 mg N/L, 30 mg P/L, 315 mg K/L), 30 m3/ha (150 ml/pot with 199 mg N/L, 45 mg P/L, 470 mg K/L), 40 m3/ha (200 ml/pot with 266 mg N/L, 60 mg P/L, 630 mg K/L), 50 m3/ha (250 ml/pot with 332 mg N/L, 75 mg P/L, 785 mg K/L), 60 m3/ha (300 ml/pot with 396 mg N/L, 90 mg P/L, 945 mg K/L), and 80 m3/ha (400 ml/pot with 532 mg N/L, 120 mg P/L, 1260 mg K/L). Thereafter, each pot was watered with its relevant N nutrient solution. Two weeks later, the plants were harvested and shoots were cut from the roots. The total leaf area per plant was determined with a LI-3000C Portable Area Meter (Li–Cor Biosciences, Lincoln, Nebraska, USA). Shoots and roots were dried to constant weight at 65°C and ground. Samples were digested and the total N content was determined as mentioned above. The total P and K were determined by ashing and dissolving in HCl before quantification as described above. The plant uptake was calculated from the shoot dry weight multiplied by the nutrient concentrations. A RCBD with four replicates was used. All statistical analyses were conducted, as previously described, by using Proc Mixed procedure of SAS 9.3 (SAS, Citation2012). The significant difference between means was established using the LSD test at p < 0.05.
2.4. Field plot trial
The study was setup in sandy loam at the horticultural farm. The soil pH and conductivity were 6.1 ± 0.4 and 130 ± 47 µS/cm, respectively. Each plot measured 3 m × 3 m with a 2 m buffer zone between plots. One herbicide treatment was applied before seeding with Impact (37 mL/ha of topramezone, BASF Mississauga, ON), followed 22 days after seeding with triazine (1.60 L/ha triazine, AATREX Liquid 480, Mississauga, ON).
Sweetcorn seeds (cv. Serendipity) were sown in June at 0.75 m between rows and 0.3 m within-row spacing. Four treatments and three replicates were arranged as a RCBD. Each treatment, except the unfertilized control, was calculated to supply about 115 kg N/ha, 25 kg P/ha and 50 kg K/ha, according to local recommendations and soil analysis (Parent & Gagné, Citation2010). The mineral N, P and K fertilizers used were calcium ammonium nitrate (27–0–0, N-P2O5-K2O), triple superphosphate (0–46-0, N-P2PO5-K2O) and granulated KCl (0-0-60, N-P2O5-K2O). The leachate samples were analyzed the previous fall and contained 1.3 g N/L, 0.3 g P/L, 3 g K/L. The treatments were: 1 – unfertilized control, 2 – NPK mineral-fertilized control, 3- leachate-fertilized calculated to provide 50 kg K/ha supplemented with N and P mineral fertilizers to reach the level of the NPK mineral-fertilized control (LF 50K completed with N and P mineral fertilizers), and 4- leachate-fertilized calculated to provide 25 kg P/ha (LF 25P); (Table ). The mineral fertilizers were surface banded by hand 10 cm from the corn plant. The leachate was mixed in the tank by circulating the liquid with a siphon pump and then 20 L Nalgene carboys with spigot were filled and transported to the cornfield, shaken before measuring the required volume per row with a plastic graduated cylinder. The leachate was manually distributed by gravity. The LF-50K leachate treatment was placed by banding at 10 cm from the corn plant, whereas for the LF-25 P treatment, the leachate treatments were placed by banding at 10 cm on both sides of the corn plant.
Table 1. Summary of the different unfertilized, conventional NPK mineral fertilization and leachate treatments applied to the field plots of sweetcorn (cv. Serendipity)
The two center rows—3 m × 1.5 m—were harvested by hand in September after the silks had turned dark brown and the kernels milky. The marketable cob yields and stovers were weighed. Grains and stovers were dried and ground, and analyzed for N, P, and K, as described earlier.
After harvesting, soil samples were taken at depths of 0–20 and 20–40 cm, air-dried, and sieved at 2 mm before pH (1:1 soil:water (w:v)) and electrical conductivity (1:5 soil:water (w:v)) determinations (CPVQ, Citation1997). For total N, soil samples were ground at 100 mesh and analyzed as previously described. Phosphorus and potassium were extracted with Mehlich 3 solution and measured by colorimetry for P (PMehlich-3) and by flame emission spectrometry for K (KMehlich-3); (CPVQ, Citation1997; Tandon et al., Citation1968).
All statistical analyses were conducted, as previously described, by using SAS 9.3 (SAS, Citation2012).
3. Results and discussion
3.1. Leachate physicochemical analyses
The leachate obtained was brown with an unpleasant odor that could be attributed to the organic acids and volatile fatty acids produced from composting food waste (Kim, Kim, Shin, Hwang, & Lee, Citation2016). Other volatile N and sulfur compounds could also have contributed to this odor.
The means of most physicochemical characteristics of the leachate studied were within the reported ranges of compost leachates (Table ). The leachate was acidic, which could be attributed to the early phase of composting. The pH of 5.4 was within the reported values of 3.7 to 9.3 for compost leachate and of 4 to 9 for agricultural soils (Caceres et al., Citation2015; Das & Kirkland, Citation2008; Gutiérrez-Miceli et al., Citation2017; Romero et al., Citation2013; Zhou et al., Citation2010). The electrical conductivity of the compost leachate was 13.6 mS/cm, a high level (Romero et al., Citation2013) associated with crop-yield reduction (Butcher, Wick, DeSutter, Chatterjee, & Harmon, Citation2016). The presence of weak and strong electrolytes consisting of organic acids and dissolved mineral nutrients in the leachate presumably induced high conductivity, which usually correlates to salinity. High conductivity and salinity interfered with physiological functions and became toxic for plants due to an abundance of ions (Butcher et al., Citation2016). Indeed, caution is required when using this leachate.
Table 2. Some physicochemical characteristics of the fruit and vegetable compost leachate and the ranges reported in the literature of compost leachates.
The total N content of our compost leachate was in agreement with those reported in the literature (Romero et al., Citation2013). About 45% of the total N was organic, consisting mainly of protein, amines, and amino-acid compounds (He et al., Citation2015). Ammonium-N represented approximately 55% of the total N content, while NO3–-N was less than 0.01%. The presence of NH4+-N could be related to the ammonification process of the protein and amino-acid decomposition, and the presence of NO3–-N to the nitrification process of NH4+-N (Caceres et al., Citation2015; Gutiérrez-Miceli et al., Citation2017; Romero et al., Citation2013). These values indicate an early stage of composting. The values of total P, K, PO4−3-P and SO4−2-S of the compost leachate in this study are consistent with the values reported by Caceres et al. (Citation2015); Gutiérrez-Miceli et al. (Citation2017) (Table ). The dissolved PO4−3-P represented 67% of the total P. The K content of the leachate was high, but remained in the range of leachate from manure compost (Caceres et al., Citation2015), potato, and olive-oil-processing waste (Arienzo, Christen, Quayle, & Kumar, Citation2009). The Na, Ca, and Mg contents of our leachate were higher than reported values for compost leachates and agricultural sewage effluents (Arienzo et al., Citation2009; Caceres et al., Citation2015; Gutiérrez-Miceli et al., Citation2017). These results indicate the presence of soluble plant mineral nutrients in the leachate, making it potentially suitable as a fertilizer but also potentially harmful due to possible competitive absorption between NH4+-N and K, and other ions. Moreover, K+, Ca++, Mg++, Na+, and Cl− ions are involved in osmotic and ionic stresses that could result in growth suppression and plant death when present in large quantities (Butcher et al., Citation2016).
The Cu and Zn contents in our leachate were similar to those reported previously (Caceres et al., Citation2015; Romero et al., Citation2013). The other trace elements were below the detection limit, i.e., As <0.5 mg/L, Cd <0.03 mg/L, Co <0.06 mg/L, Cr <0.06 mg/L, Hg <0.6 mg/L, Mo <0.1 mg/L, Ni <0.1 mg/L, Pb <0.4 mg/L, and Se <1 mg/L (Table ). These low concentrations of metallic trace elements indicate no threat to the environment.
3.2. Germination assay
The pH and electrical conductivity of the leachate dilutions varied from 6 to 7 and from 0.3 to 12.3 dS/m, respectively (Table ). When distilled water was added to dilute the leachate, the pH increased and the electrical conductivity decreased, allowing greater germination, rootlet length, and germination index. The presence of toxic compounds in a solution inhibited germination and rootlet growth. The compost leachate at 0%, 25%, and 50% dilution inhibited cress germination, but for corn, 47% of the seeds germinated in the leachate without dilution. NH4+-N and K above 100 mM have been reported to inhibit the germination processes (Barker, Maynard, Mioduchowska, & Buch, Citation1970). Also, Hoekstra, Bosker, and Lantinga (Citation2002) reported that high electrical conductivity inhibited germination. The dilutions reduced the electrical conductivity, and decreased the content in NH4+-N, K, ions, salts, organic acids, and other compounds. Overall, these results imply that the compost leachate is somewhat phytotoxic to the seed germination process.
Table 3. Effect of dilution of compost leachate (L) with distilled water (W) on the average pH, electrical conductivity (EC), germination, rootlet length, and germination index of cress and corn
3.3. Greenhouse assay
Under greenhouse conditions, the impacts of seven leachate rates were investigated to determine the level of phytotoxicity when applied once at a growing stage of 3–4 leaves (Figures and ). Compared to the low-N control, the application of 10 to 50 m3/ha of leachate significantly increased corn leaf area, shoot and root dry weights (P < 0.05). At these rates, the leachate demonstrated that it could be a fertilizer source. Applications of 60 and 80 m3/ha of leachate decreased the leaf area (Figure ) and shoot and root weights (Figure ). At rates above 60 m3/ha, the application of the leachate could be harmful, likely due to ionic and osmotic stresses.
Figure 1. Effect of leachate application rate on total leaf area per plant compared to low (2 mM N; CK−) and high mineral N-fertilized controls (20 mM N; CK+) of corn grown for 5 weeks in the greenhouse and in 3.7 L pots filled with vermiculite and Turface calcined clay. The leachate contained 133 mg N/100 ml, 30 mg P/100 ml, and 315 mg K/100 ml, and the volume added per pot varied from the equivalent of 10 m3/ha (50 ml) to 80 m3/ha (400 ml). The dotted line represents leaf area obtained with CK- treatment. Data are mean values of four replicates with one plant per pot. Means associated with the same letter are not significantly different according to the LSD test (P < 0.05).
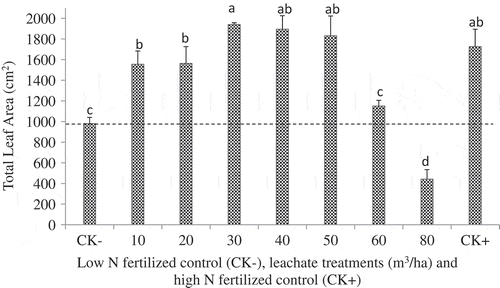
Figure 2. Effect of increasing leachate application rates on shoot and root dry matter yields per plant compared to low (2 mM N; CK−) and high (20 mM N; CK+) mineral N-fertilized controls of corn grown for 5 weeks in the greenhouse in 3.7 L pots filled with vermiculite and Turface calcined clay. The leachate contained 133 mg N/100 ml, 30 mg P/100 ml, and 315 mg K/100 ml, and the volume added per pot varied from the equivalent of 10 m3/ha (50 ml) to 80 m3/ha (400 ml). Dotted and black lines are, respectively, shoot and root yields obtained with the CK− treatment. Data are mean values of four replicates with one plant per pot. Means associated with the same capital or lowercase letter are not significantly different according to the LSD test (P < 0.05).
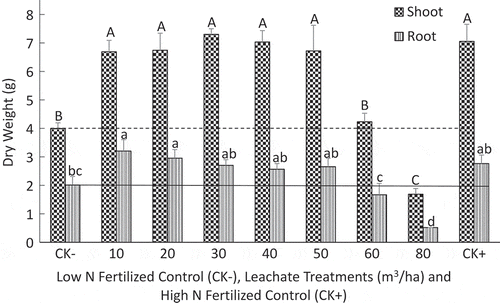
The low (CK−) and high-N (CK+) fertilized controls were used to compare shoot concentration and uptake of corn nutrients, but only the 20, 40, 60, and 80 m3/ha leachate treatments were analyzed (Table ). The concentration and uptake of most nutrients with the 20 m3/ha leachate treatment were lower than in the low-N control, which could indicate nutrient deficiencies. The plant nutrition, when 40 m3/ha of leachate were added, was similar to the high-N control. The N, Ca, and Mg concentrations for the 60 and 80 m3/ha of leachate treatments were higher than the high-N control. Also, for these treatments, the uptake of P, K, Cu, and Zn was lower than the high-N control. The concentration of several ions in the leachate treatments above 40 m3/ha presumably unbalanced the ion uptake compared to the high-N control. The high level of NH4+-N in the leachate could impair cation uptake, leading to a toxic level of plant N. Also, the plants could accumulate NH4+-N in response to high salinity and conductivity (Butcher et al., Citation2016). In this study, the leaf area, the shoot and root biomasses, and the nutrient concentration and uptake of corn assessed the relative risk of phytotoxicity, and established that the leachate application should be limited to less than 40 m3/ha per application.
Table 4. Effect of the rate of leachate application on shoot concentration and uptake of nutrients compared to low (2 mM; CK−) and high (20 mM; CK+) nitrogen fertilizer controls for the Providence corn cultivar grown in the greenhouse for five weeks.
3.4. Field plot trial
The corn nutrient requirements were used to determine the amount of leachate to be used under field conditions at three corn-growing stages: seedling, 3–4 leaves and 7–8 leaves. As recommended for field corn (Parent & Gagné, Citation2010), the addition of N + K at seeding, either for leachates or a combination of leachates and mineral fertilizers, was kept under 100 kg/ha. The LF 50K (9 m3/ha leachate completed with N and P mineral fertilizers) and the LF 25P (72 m3/ha leachate) treatments were compared to unfertilized and NPK mineral-fertilized controls. Indeed, the LF 50K treatment could meet the K fertilizer requirement of corn with the application of 9 m3/ha of leachate. The LF 25P could meet the NPK fertilizer requirements of corn with the application of 72 m3/ha of leachate. After leachate applications, no leaf yellowing was noted, and the plant heights were similar among all treatments (data not shown). The leachate and control treatments gave similar cob and stover fresh matter yields (Table ). All treatments fulfilled the corn fertilizer requirements and confirmed the absence of phytotoxicity when the leachate was used at an agronomic rate to meet plant nutrition. The corn biomass increased in response to available N but not to available K, and demonstrated that the yield remained similar even at 269 K kg/ha (Rehm & Sorensen, Citation1985), which is similar to the current study with a total of 226 K kg/ha. Past studies have reported similar yields regardless of the potassium fertilization rate (Zhang et al., Citation2010).
Table 5. Effect of different leachate and fertilizer treatments on the cob and stover fresh matter yields, and grain and stover N, P, and K concentrations of sweet corn (cv. Serendipity) grown under field conditions.
For the plant nutrition, the LF 50K treatment gave grain and stover N concentrations similar to the mineral fertilized control (Table ). The LF 25P treatment, however, significantly decreased the grain and stover N concentrations compared to the mineral fertilized control, but they were similar to the unfertilized control. Presumably, the LF 25P treatment could not provide the optimum N nutrition (lack of 16 kg N/ha) that could partly explain the lower N concentration in the corn, but the competitive uptake of cations versus that of NH4+-N could also be involved (Zhang et al., Citation2010). Both leachate treatments gave similar grain and stover P and K concentrations.
At the end of the growing season, soil samples at depths of 0–20 and 20–40 cm were analyzed. No significant differences among the treatments and controls were measured in terms of soil pH, electrical conductivity, total N, and available P and K at depths of 0–20 cm (Table ) and 20–40 cm (data not shown). Arienzo et al. (Citation2009) reported that K built up in the soil after waste amendment. In this study, the sandy loam and rainy weather could have prevented K accumulation in the soil. Lastly, the acidic property of the compost leachate could have prevented the buildup of soil K.
Table 6. Residual effect of different leachate and fertilizer treatments on surface field soil (0–20 cm) pH; electrical conductivity; N, P, and K concentrations after corn harvest
4. Conclusion
The results of our study pointed out that the compost leachate contained plant nutrients that could be used on farms to close the plant nutrient and water cycles. This studied compost leachate contained N, mainly as ammonium, and K. The high level of ions gave a high electrical conductivity that predicted the phytotoxicity of leachate in plant germination and bioassays. The leachate-fertilized treatment calculated to provide 50 kg K/ha supplemented with N and P mineral fertilizers presumably minimized the environmental impacts of K, but was difficult to apply since it combined leachate and mineral fertilizers. The leachate-fertilized treatment calculated to provide 25 kg P/ha was easy to apply since it consisted of only leachate, but implied the application of large amounts of potassium for which there is no regulation. Within these limits, the leachate-fertilized treatment calculated to provide 25 kg P/ha treatment could be used to substitute most mineral fertilizers for corn.
Further research on compost leachate is, however, needed to understand the fate of nutrients in plant uptake for different crops. Also, this study falls short of characterizing the leachate microflora, so more data are needed to address the effect of compost leachate on soil microflora.
Datasets are available on request
The raw data supporting the conclusions of this manuscript will be made available by the authors, without undue reservation, to any qualified researcher.
Additional information
Funding
Notes on contributors
Papa Malick Sall
Papa Malick Sall (sampling compost on this picture; orcid.org/0000-0002-0913-9688) received his M. Sc. from Département de phytologie, Faculté des Sciences de l’Agriculture et de l’Alimentation (FSAA), Université Laval and completed his Ph. D. in soil chemistry in the Département des sols et génie agroalimentaire, FSAA, Université Laval. H. Antoun (orcid.org/0000-0001-9309-420X) is a professor emeritus in the Département des sols et génie agroalimentaire, FSAA, Université Laval. F.-P. Chalifour (orcid.org/0000-0002-3669-1822) and C.J. Beauchamp (orcid.org/0000-0003-3981-3431) are full professors in the Département de phytologie at Université Laval. Professor Chalifour works on knowledge development pertaining to crop physiology. Professor Beauchamp’s area of research is waste–plant–microbe interactions to improve crop and soil health.
References
- Arienzo, M., Christen, E. W., Quayle, W., & Kumar, A. (2009). A review of the fate of potassium in the soil–plant system after land application of wastewaters. Journal of Hazardous Materials, 164(2–3), 415–422. doi:10.1016/j.jhazmat.2008.08.095
- Barker, A. V., Maynard, D. N., Mioduchowska, B., & Buch, A. (1970). Ammonium and salt inhibition of some physiological processes associated with seed germination. Physiologia Plantarum, 23(5), 898–907. doi:10.1111/ppl.1970.23.issue-5
- Beaudette, V. (2014). Caractérisation des liquides issus du compostage de résidus agroalimentaires végétaux. ( Master’s thesis), Université Laval, Québec. Retrieved from http://hdl.handle.net/20.500.11794/25340(30693)
- Butcher, K., Wick, A. F., DeSutter, T., Chatterjee, A., & Harmon, J. (2016). Soil salinity: A threat to global food security. Agronomy Journal, 108(6), 2189–2200. doi:10.2134/agronj2016.06.0368
- Caceres, R., Magri, A., & Marfà, O. (2015). Nitrification of leachates from manure composting under field conditions and their use in horticulture. Waste Management, 44, 72–81. doi:10.1016/j.wasman.2015.07.039
- Chalifour, F.-P., & Nelson, L. M. (1988). Effects of time of nitrate application on nitrate reductase activity, nitrate uptake, and symbiotic dinitrogen fixation in faba bean and pea. Canadian Journal of Botany, 66(8), 1646–1652. doi:10.1139/b88-224
- CPVQ (Conseil des productions végétales du Québec). (1997). AGDEX 533. Protocoles d’analyses des sols, des fumiers et des tissus végétaux (2nd ed.). Québec.
- Das, K. C., & Kirkland, J. T. (2008). Quantification of water extractable contaminants from food waste and biosolids blends at different stages of composting. Compost Science & Utilization, 16(3), 200–206. doi:10.1080/1065657X.2008.10702378
- Gooch, M. V., & Felfel, A. (2014). The cost of Canada’s annual food waste. Value Chain Management International Inc. http://vcm-international.com/wp-content/uploads/2014/12/Food-Waste-in-Canada-27-Billion-Revisited-Dec-10-2014.pdf
- Gutiérrez-Miceli, F. A., García-Gómez, R. C., Oliva-Llaven, M. A., Montes-Molina, J. A., & Dendooven, L. (2017). Vermicomposting leachate as liquid fertilizer for the cultivation of sugarcane (Saccharum sp.). Journal of Plant Nutrition, 40(1), 40–49. doi:10.1080/01904167.2016.1193610
- He, X.-S., Xi, B.-D., Zhang, Z.-Y., Gao, R.-T., Tan, W.-B., Cui, D.-Y., & Yuan, Y. (2015). Composition, removal, redox, and metal complexation properties of dissolved organic nitrogen in composting leachates. Journal of Hazardous Materials, 283, 227–233. doi:10.1016/j.jhazmat.2014.09.027
- Hoekstra, N. J., Bosker, T., & Lantinga, E. A. (2002). Effects of cattle dung from farms with different feeding strategies on germination and initial root growth of crossed (Lepidium sativum L.). Agriculture, Ecosystems and Environment, 93(1), 189–196. doi:10.1016/S0167-8809(01)00348-6
- Isaac, R. A., & Johnson, W. C. (1976). Determination of total nitrogen in plant tissue, using a block digestor. Journal- Association of Official Analytical Chemists, 59, 98–100.
- Jarecki, M. K., Chong, C., & Voroney, R. P. (2012). Evaluation of compost leachate for growing nursery trees on a waste-rehabilitated field site. Compost Science & Utilization, 20(3), 171–180. doi:10.1080/1065657X.2012.10737043
- Kim, H., Kim, J., Shin, S. G., Hwang, S., & Lee, C. (2016). Continuous fermentation of food waste leachate for the production of volatile fatty acids and potential as a denitrification carbon source. Bioresource Technology, 207, 440–445. doi:10.1016/j.biortech.2016.02.063
- Machrafi, Y., Prevost, D., & Beauchamp, C. J. (2006). Toxicity of phenolic compounds extracted from bark residues of different ages. Journal of Chemical Ecology, 32(12), 2595–2615. doi:10.1007/s10886-006-9157-1
- Nkonge, C., & Ballance, G. M. (1982). A sensitive colorimetric procedure for nitrogen determination in micro-Kjeldahl digests. Journal of Agricultural and Food Chemistry, 30(3), 416–420. doi:10.1021/jf00111a002
- Parent, L.-É., & Gagné, G. (2010). Guide de référence en fertilisation. In Centre de référence en agriculture et agroalimentaire du Québec (2nd ed., pp. 473). Quebec, Canada: CRAAQ, Commission chimie et fertilité des sols.
- Rehm, G., & Sorensen, R. (1985). Effects of potassium and magnesium applied for corn grown on an irrigated sandy soil. Soil Science Society of America Journal, 49(6), 1446–1450. doi:10.2136/sssaj1985.03615995004900060023x
- Romero, C., Ramos, P., Costa, C., & Márquez, M. C. (2013). Raw and digested municipal waste compost leachate as potential fertilizer: Comparison with a commercial fertilizer. Journal of Cleaner Production, 59, 73–78. doi:10.1016/j.jclepro.2013.06.044
- Sall, P. M., Antoun, H., Chalifour, F.-P., & Beauchamp, C. J. (2016). On farm composting of fruit and vegetable waste from grocery stores: A case under cold climatic conditions of Eastern Canada. Paper presented at the SUM2016; Third Symposium on Urban Mining and Circular Ecology, Bergamo, Lombardy, Northern Italy.
- SAS. (2012). The SAS system for windows. Version 6.12. Cary, N.C.: Author.
- Savage, A. J., & Tyrrel, S. F. (2005). Compost liquor bioremediation using waste materials as biofiltration media. Bioresource Technology, 96(5), 557–564. doi:10.1016/j.biortech.2004.06.016
- Tandon, H., Cescas, M., & Tyner, E. (1968). An acid-free vanadate-molybdate reagent for the determination of total phosphorus in soils. Soil Science Society of America Journal, 32(1), 48–51. doi:10.2136/sssaj1968.03615995003200010012x
- Zhang, F., Niu, J., Zhang, W., Chen, X., Li, C., Yuan, L., & Xie, J. (2010). Potassium nutrition of crops under varied regimes of nitrogen supply. Plant and Soil, 335(1–2), 21–34. doi:10.1007/s11104-010-0323-4
- Zhou, C., Wang, R., & Zhang, Y. (2010). Fertilizer efficiency and environmental risk of irrigating impatiens with composting leachate in decentralized solid waste management. Waste Management, 30(6), 1000–1005. doi:10.1016/j.wasman.2010.02.010