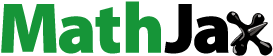
Abstract
Effects of defatted soy meal flour (0, 10 and 20%), barrel temperature (135, 150 and 165°C) and feed moisture content (15, 18 and 21%) on proximate composition, minerals, antinutritional factors and sensory properties of extruded products were studied using factorial design. Increasing the addition level of defatted soy meal flour to sorghum flour resulted in a significant increase (P < 0.05) in moisture, crude protein, fat, ash, fiber, Ca and Fe content but decrease in carbohydrate content. Barrel temperature of 135, 150 and 165°C caused a significant reduction in tannin content by about 61, 76 and 86%, and in phytic acid content by 24, 35 and 45%, respectively. The mean sensory values of all extrudates were 3.31 for crispness on 5-point hedonic scale, 4.93, 5.76 and 5.16 for color, flavor and overall acceptability on 7-point hedonic scale, respectively; these indicate all extrudates were well liked by the judges.
PUBLIC INTEREST STATEMENT
Sorghum is an important crop that has been utilized by millions of people in developing countries, especially in Africa, as a source of energy and protein. However, sorghum is far from satisfying the nutritional requirments of consumers due to the fact that a considerable number of people in the respective countries have been affected by malnutrition and its various forms. For this reason, views have been expressed the need to improve the nutritional quality of sorghum-based foods through enrichment and improved processing technology. Accordingly, this study was conducted to investigate the possibility of producing acceptable sorghum-based extruded product supplemented with defatted soy meal flour. As a result, it was testified that it is possible to get acceptable and nutritionally superior expanded product from sorghum and defatted soy meal flours mixture by extrusion cooking. Such product, therefore, could undoubtedly be contributed to reduce malnutrition and its various forms.
1. Introduction
In the continuous search for solution to the problem of malnutrition and its various forms, mainly among the people of the developing countries including Ethiopia, views have been expressed of the need to improve the nutritive quality of foods of the respective countries through better processing and enrichment.
Sorghum (Sorghum bicolor (L.) Moench) is an important cereal crop grown in the semi-arid tropics of Africa and Asia due to its drought tolerance. It is a staple food crop cultivated on a subsistence level by farmers in these areas for human consumption and plays an important role in food security (Taylor, Schober, & Bean, Citation2006). Starch is the main component of sorghum grain, followed by proteins, non-starch polysaccharides and fat (Dicko, Gruppen, Traoré, Voragen, & van Berkel, Citation2006). While sorghum starch provides all the features for production of highly acceptable extruded products (Devi et al., Citation2013), its nutritional quality is far from satisfying the needs of consumers (Belton & Taylor, Citation2004). For this reason, several attempts have been done to improve the nutritional quality of sorghum-based products using legume flours as a compliments (Anuonye, Onuh, Egwim, & Adeyemo, Citation2010; Gbenyi, Nkama, & Badau, Citation2016; Hegazy, El-bedawey, Rahma, & Gaafar, Citation2017; Kumar, Samuel, Jha, & Sinha, Citation2015; Pelembe, Erasmus, & Taylor, Citation2002; Suksomboon, Limroongreungrat, Sangnark, & Thititumjariya, Citation2011).
Compared with most other protein sources, defatted soy meal flour is a cheap source of protein, as it is a by-product of solvent extraction of soybean oil (Ströher, Stenzel, Pereira, & Zanin, Citation2012), and contains high concentration of essential amino acids such as lysine and tryptophan (Odebode et al., Citation2018). Therefore, it can possibly be considered as a candidate material to complement the protein and amino acid profile of sorghum flour.
Some research works have been done on extrusion cooking of sorghum and soybean flours to improve the nutritional quality of sorghum-based extruded products (Adedeji, Suhr, Bhadriraju, & Alavi, Citation2016; Basediya, Pandey, Shrivastava, Khan, & Nema, Citation2013; Kumar et al., Citation2015). However, there is no information available on the possibility of using defatted soy meal as a complement to produce sorghum-based extruded products. Therefore, the aim of this study was to investigate the effect of sorghum and defatted soy meal flour blending ratio and extrusion processing parameters on the nutritional and sensory quality of extrudates.
2. Materials and methods
2.1. Experimental materials
Sorghum (Sorghum bicolor (L.) Moench) was obtained from Haramaya University research center. Sorghum grain variety (Muyera I) was selected based on its bulk production in the country. Defatted soy (Glycine max) meal was obtained from Health Care Food Manufacturer PLC, Addis Ababa.
2.2. Material preparation
Before milling, the sorghum grains were cleaned for physical impurities. About 20% water was added to temper the grains to a moisture content of 16% (Taylor & Dewar, Citation2001) to toughen the pericarp and soften the endosperm. Then the grains were mechanically dehulled, using a commercial dehuller, and milled using a stone mill. The defatted soy meal was cleaned and oven dried at 70°C for 2 h, and milled using stone mill. Finally, both the flours were sifted to pass through 480 μm mesh sieve (Perez et al., Citation2008), packed in plastic bags and stored at room temperature (25°C).
2.3. Extrusion operation
Samples prepared as described in the above section were subjected to extrusion test at all combinations of the operating conditions (feed moisture content, barrel temperature and defatted soy meal flour level). Real values for barrel temperature (135–165°C), feed moisture content (15–21%) and amount of defatted soy meal flour (0–20%) were used (Table ). These values of the parameters were set based on preliminary test.
Table 1. Experimental levels of the variables
Extrusion was performed using a pilot scale co-rotating twin-screw food extruder (model Clextral, BC-21 No 124, Firminy, France) at preset temperature of zone 2 and zone 3 of the extruder barrel sections. The barrel temperature was fixed in zone 2 at 70°C. Feed rate and screw speed was kept constant at 9 kg/h (Solomon, Citation2007) and 150 rpm (Perez et al., Citation2008), respectively throughout the experiment. The pump was adjusted to give the amount of water required to bring the moisture to 15%, 18% and 21% in the mixes at constant material feed rate by using the following hydration equation (Golob, Farrell, & Orchard, Citation2002):
where Wa is the weight of water added (g), Sw is sample flour weight (g), mo is original flour moisture content (% wwb), and m is the required dough moisture level in (% wwb).
Samples were extruded as straight rope (rod) for a time interval of 10 s (Mason & Hoseney, Citation1986). The extruded products were placed on a Table and allowed to cool for 30 min at room temperature and sealed in moisture proof plastic bags after equilibration for 24 h at ambient condition (25°C).
2.4. Experimental design
A factorial design was used to study the effect of defatted soy meal flour level, barrel temperature and feed moisture contents on nutritional and sensory properties of extruded products. Thus, there were 27 treatment combinations: 3 (soy meal flour levels) × 3 (barrel temperatures) × 3 (feed moisture contents). Experiment was conducted in duplicates.
2.5. Determination of proximate composition, mineral contents and anti-nutrition factors
Standard methods of American Association of Cereal Chemists (American Association of Cereal Chemists [AACC], Citation2000) were used for determination of proximate composition (moisture content, crude protein, crude fat, ash content, and crude fiber and carbohydrate contents) and mineral contents (calcium, iron and zinc) of defatted soy meal flour, sorghum flour and extrudates. Vanillin-HCl methanol method of Price, Van Scoyoc, and Butler (Citation1978) was used for the determination of condensed tannins using spectrophotometer at 450 nm. The method described by (Wheeler & Ferrel, Citation1971) was used to determine the phytic acid content of the raw materials and extrudates using spectrophotometer at 822 nm.
2.6. Sensory evaluation
Color, texture (crispiness), flavor and overall acceptability of extrudate samples were evaluated by 25 panels selected from students and staffs of the department of Food Technology and Food Process Engineering, Bahir Dar University, Ethiopia. In order to reduce the number of samples to be provided to the panels, preliminary selection of extrudate samples were carried out. Twelve (12) extrudate samples were selected out of 27 extrudates based on representativeness and their physical properties. A 7-scale hedonic tests were applied for color, flavor and overall acceptance, and 5-scale hedonic tests were applied for crispiness. Extrudates were served to panelists at room temperature using 3 digit codes for sensory analysis.
2.7. Data analysis
Analysis of variance (ANOVA) was carried out to investigate the effect of blending ratio and operating conditions on proximate, mineral, anti-nutrition contents and sensory quality of extruded products using SAS software (SAS Institute and Cary, NC, USA). Duncan Multiple Range Test (DMRT) was used for multiple mean separations at 5% probability level.
3. Results and discussion
3.1. Proximate composition of sorghum and defatted soy meal flours
Proximate composition of sorghum and defatted soy meal flours used in this study are presented in Table .
Table 2. Proximate composition of sorghum and defatted soybean flour
3.2. Effect of defatted soy meal flour proportion, barrel temperature and feed moisture content on proximate composition of extrudates
The effects of soy meal flour proportion, barrel temperature and feed moisture content on proximate composition of extrudates are presented in Table . Soy meal flour proportion showed significant (p ≤ 0.05) effect on product proximate composition (Table ). Results (Table , ) show that the moisture content of extrudates was in the range of 8.15 to 8.66%, suggesting the products had moisture content low enough to have an extended shelf life. In dry food systems, moisture content of between 6 and 10% has been established to prolong the shelf life of foods, beyond which the storability of the system could be impeded by chemical and microbiological agents (Brncic et al., Citation2006).
Table 3. Main effect of extrusion variables on product proximate composition
Table 4. Effect of extrusion variables on product proximate compositions
The moisture content of the products was significantly increased (P < 0.0001) from 8.37 to 8.50% with increase in level of defatted soy meal flour from 0 to 20% of sorghum flour (Table , ). This could be due to the increased water hydration capacity of the blend with increased soy meal flour level. The product moisture content significantly increased (P < 0.0001) from 8.30 to 8.50% with an increase in feed moisture content from 15 to 21% (Table , ). However, the barrel temperature did not show significant (p ≥ 0.05) change on the moisture content of the resulting extrudates (Table ). The increase in moisture content with increase in feed moisture content could be due to insufficient vaporization occurring at high moisture content.
Result (Table , ) shows the interaction of the three variables was found to have a significant effect (p < 0.0006) on the moisture content of the resulting extrudates. Generally, the highest moisture content (8.66%) of extrudates was found at the highest levels of soy meal flour (20%) and feed moisture content (21%), and the lowest barrel temperature (135°C). The lowest moisture content (8.15%) was observed at 0% soy meal flour addition, 165°C barrel temperature and 21% feed moisture content (Table ).
The protein content of the extrudates significantly increased (P < 0.0001) from 12.84 to 20.08 with increasing level of soy meal flour from 0 to 20% (Table , ). This could obviously be due to the higher amount of protein content in soy meal flour. The crude protein content of extruded products was significantly decreased (P < 0.05) from 16.69 to 16.31% with increase in barrel temperature from 135 to 150°C (Table , ). However, further increasing the barrel temperature to 165°C did not show significant (p ≥ 0.05) change in protein content of the extrudates (Table ). The reduction in crude protein content could be due to the denaturation and maillard reaction of protein as a result of high temperature. The protein content of the extrudates was not significantly (p ≥ 0.05) affected by feed moisture content (Table ). Result (Table , ) shows the interaction of the three variables was not significantly (p ≥ 0.05) affected the protein content of the resulting extrudates. However, the highest protein content (20.87%) was found from the highest levels of soy meal flour (20%), the lower barrel temperature (135°C) and feed moisture contents of 18% and 21%. The lowest protein content of extrudates (12.20%) was found at the lowest levels of soy meal flour (0%) and feed moisture content (15%), and the middle level of barrel temperature (150°C).
With increasing the level of soy meal flour from 0 to 20%, the ash and fiber contents of the extrudates significantly (p < 0.0001) increased from 1.53 to 2.40% and 0.84 to 1.57%, respectively (Table , ). These could obviously due to the higher amount of ash and fiber content of soy meal flour. The crude fiber content of extrudates was significantly (p < 0.027) decreased from 1.24 to 1.22% as the barrel temperature increased from 135 to 150°C, but further increase in barrel temperature to 165°C did not significantly (p ≥ 0.05) change the crude fiber content (Table , ). However, the ash content of the resulting extrudates was not significantly (p ≥ 0.05) affected by barrel temperature (Table ). The interaction of the three variables did not show significant (p ≥ 0.05) effect on the ash and fiber content of the extrudates (Table , ). As can be seen in Table , there were no significant differences in ash and fiber contents observed among the extrudates produced from the same level of soy meal flour at different barrel temperatures and feed moisture contents. The higher values of ash and fiber content were found from 20% soy meal flour addition at all levels of barrel temperatures and feed moisture contents. These indicate that soy meal flour is the most dominant factor affecting ash and fiber contents.
The carbohydrate content of the resulting extrudates was significantly (p < 0.0001) decreased from 73.32 to 63.52% with increasing the level of soy meal flour from 0 to 20% (Table , 10). However, barrel temperature and feed moisture content was not significantly (p ≥ 0.05) affected the carbohydrate content of the resulting extrudates (Table 10). A result (Table , ) shows the carbohydrate contents of the extrudates were in the range of 62.60–72.51% with no significant (p ≥ 0.05) differences. Decrease in carbohydrate and increase in protein, fat, ash and crud fiber contents could obviously be due to the higher amount of protein, fat, ash and fiber contents of soy meal flour. Similar finding supporting these results were reported (Abioye, Ade-Omowaye, Babarinde, & Adesigbin, Citation2011; Adunni & Olaposi, Citation2010; Shogren, Hareland, & Wu, Citation2006), showing increases of protein, fiber, and ash content with increasing soy meal flour.
Table 5. Main effect of extrusion variables on extrudate mineral contents
3.3. Effect of defatted soy meal flour proportion, barrel temperature and feed moisture on extrudate mineral contents
The effects of soy meal flour level, barrel temperature and feed moisture on extrudate mineral contents are presented in Table . The Ca and Fe content of extrudates were significantly increased (P < 0.05) from 51.82 to 112.9, and 7.04 to 14.42 mg/100 g, respectively, with an increase in level of soy meal flour from 0 to 20%. However, proportion of soy meal flour did not have significant effect (P ≥ 0.05) on the Zn content of the extrudates. The increase in Ca and Fe contents of extrudates were expected as soy meal flour contain relatively higher levels than sorghum. Asare et al. (Citation2010) reported the incorporation of cowpea and groundnut resulted in a significant increase in the calcium and iron contents of the sorghum–legume extrudates.
Fe content of extrudates was significantly affected (P < 0.05) by barrel temperature whereas the Ca and Zn contents were not (P ≥ 0.05) significant (Table , ). Increasing barrel temperature from 135 to 150°C did not significantly (P ≥ 0.05) change the Fe content of the extrudates. Further increasing the barrel temperature to 165°C significantly (P < 0.05) increased the Fe content from 10.48 to 11.13mg/100g. High content of Fe at the highest temperature might be due to the reduction of antinutritional contents during extrusion cooking. Phytate, the major phosphorus bearing, compounds in cereals and pulses, chelates divalent and trivalent cations, such as Ca, Fe and Zn, forming insoluble complexes and thereby decreases the in vitro availability of minerals (Dhingra & Jood, Citation2002). The feed moisture content did not show a significant effect (P ≥ 0.05) on Ca, Zn and Fe content of the extrudates (Table ).
The interaction effect of soy meal flour, barrel temperature and feed moisture content on minerals content of extrudates is presented in Table . There were no significant differences in Ca contents observed among the extrudates produced from the same level of soy meal flour at different barrel temperatures and feed moisture contents combinations except extrudates produced from the highest level of soy meal flour (20%), the highest barrel temperature (165°C) and the lowest feed moisture content (15%) which was recorded as the highest value of Ca (118.08 mg/100 g). These indicate that soy meal flour is the dominant factor that affects the calcium contents of extrudates. The interaction among the three independent variables showed a significant effect (P < 0.0021) on Fe content of the resulting extrudates (Table , ). The highest value of Fe (15.76 mg/100 g) was obtained at the highest soy meal flour proportion (20%), barrel temperature (165°C) and lowest feed moisture content (15%), and the least value (5.99 mg/100 g) was observed at 0% soy meal flour proportion, 135°C barrel temperature and 21% feed moisture. Though, the Zn contents of the resulting extrudates were not significantly different (p > 0.05), it was found in the range of 4.74–4.90 mg/100 g (Table 6, ). A serving of 100 g of extrudates produced from 20% soy meal flour proportion could provide about 11, 32 and 78% of the recommended dietary allowance (RDA) for Ca, Zn and Fe, respectively.
Table 6. Effect of extrusion variables on mineral content of the products
3.4. Effect of defatted soy meal flour proportion, barrel temperature and feed moisture content on antinutritional contents of extrudates
3.4.1. Condensed tannin content
Table summarizes the main effect of extrusion variables on antinutritional content of extrudates. The tannin content of extrudates was significantly affected (P ≤ 0.05) by feed moisture content and barrel temperature (Table 10). However, proportion of soy meal flour did not show a significant effect (P ≥ 0.05) on the tannin content of extrudates (Table ). This was probably due to the lower amount of tannin in soy meal flour than sorghum.
Table 7. Main effect of extrusion variables on antinutritional content of extrudates
Extrusion of sorghum and soy meal flour blend at 15, 18 and 21% feed moisture content significantly reduced (P < 0.0001) the tannin content of extrudates by 76.45, 72.96 and 71.41%, respectively, from raw sorghum flour (303.75 mg/100 g; Table 7, ). Lower reduction of tannin content at maximum feed moisture content could be the short residence time of the dough in extruder barrel due to the high lubricating effect of the high feed moisture content. This result support the finding by Nwabueze (Citation2007), when extruding African breadfruit based spaghetti, observed 89% reduction in tannin content at lower feed moisture content.
Barrel temperature also found to have a significant effect (P < 0.0001) on tannin content of extrudates (Table , ). Extruding sorghum and soy meal flour blend at 135, 150 and 165°C, at the same feed moisture content and level of soy meal flour, caused a significant reduction in tannin content of the raw sorghum flour (303.75 mg/100 g) by about 61, 76 and 86%, respectively. This could be due to destruction of tannin at high temperature (Anton, Fulcher, & Arntfield, Citation2009).
The interaction between extrusion variables did not show a significant (P>0.05) effect on tannin content of extrudates (Table 10) . Numerically, however, the highest tannin content reduction (88.3%) was found at 20% soy meal flour addition, 15% feed moisture content and 165°C barrel temperature (Table ).
Table 8. The effect of combination of extrusion variables on antinutritional content of extrudates
3.4.2. Phytic acid content
The phytic acid content of extrudates was significantly affected (P < 0.0001) by the proportion of defatted soy meal flour and barrel temperature whereas feed moisture content had not a significant effect (P ≥ 0.05) on phytic acid content of the extrudates (Table , ). Increasing the level of addition of soy meal flour from 0 to 20% caused a significant (P < 0.0001) increase in phytic acid content of extrudates from 323.47 to 428.33 mg/100 g. This could obviously be due to the high amount of phytic acid in soy meal flour. Extruding sorghum and soy meal flour at 135, 150 and 165°C, at the same soy meal flour level and feed moisture content, caused a significant (P < 0.0001) reduction in phytic acid content of raw sorghum flour (553.28 mg/100 g) by about 24, 35 and 45%, respectively. This could be due to degradation of phytate to simple and lower molecular weight components as barrel temperature increased (Anton et al., Citation2009). Nwabueze (Citation2007) reported 27.7–32.7% reduction in phytic acid by extrusion cooking.
Generally, the highest phytic acid reduction of about 55% was observed at 0% soy meal flour proportion, 165°C barrel temperature and 15% feed moisture content while the lowest (26%) reduction was observed at 20% soy meal flour amount, 135°C barrel temperature and 21% feed moisture content (Table ). Reduction in phytic acid content of cereals and legume blend extruded products has been reported (Anton et al., Citation2009). The reduction in phytic acid during extrusion cooking was attributed to the hydrolyzation of inositol hexaphosphate to penta-, tetra- and triphosphates (Anton et al., Citation2009). This reduction in phytic acid may be useful in improving nutritional quality of sorghum with respect to mineral bioavailability.
3.5. Sensory evaluation of sorghum and defatted soy meal flour extrudates
The mean sensory scores for the selected products are summarized in Table . The sensory evaluation for color, flavor, crispness and overall acceptance revealed significant difference (P < 0.0001) exist between the selected extruded products (Table ).
Table 9. Sensory mean scores of extruded products
Table 10. P-Values
Among the extrudates prepared from different level of soy meal flour addition at different operating conditions, extrudate prepared from 10% soy meal flour proportion and at process condition of 165°C barrel temperature and 15% feed moisture content was most preferred (6.68) in terms of color while extrudate prepared from 0% soy meal flour proportion (sorghum flour alone) at 150°C barrel and 18% feed moisture was rated lowest (2.96) for color by judges (Table ). The judges rated the highest color score for extrudate prepared from 10% soy meal flour. This may be due to the yellow color of extrudates. Pelembe et al. (Citation2002) found that an increased proportion of cowpeas resulted in an increase in the desirable yellow color of extrudates. The relative higher color score at high barrel temperature and lower feed moisture content could be related to maillard and caramelization reactions, high expansion of extrudate and starch dextrinization. Shi et al. (Citation2011) reported that the product with least expansion have a compact structure and appear dull in color.
Mean sensory scores for flavor are shown in Table . The mean value of all extrudates for flavor was 5.76, indicating that all products were well liked by the judges. Extrudate from 10% soy meal flour which were processed at 165°C barrel temperature and 15% feed moisture content had the higher flavor score (6.80). However, the flavor score of 4.88 for extrudates made from 0% soy meal flour level (sorghum flour alone) at 150°C and 18% feed moisture content was recorded as the lowest score. The higher flavor score at higher temperature and lower moisture content could be due to the increased in volatile compounds and flavor intensity because of high degree of maillard and caramelization reaction.
The mean value of all selected extrudates sensory score for crispness was 3.31, indicating that the products were well liked by the judges (Table ). The highest crispness score of 4.66 and 4.48 were observed at 0% soy meal flour proportion (sorghum flour alone), 165°C barrel temperature and 15% feed moisture content; and 10% soy meal flour, 165°C barrel temperature and 15% feed moisture content, respectively. Extrudate product prepared from 20% soy meal flour proportion at 150°C and 18% feed moisture content was rated as the least crispness score (1.96). Increasing temperature progressively results in greater porosity of extrudates due to formation of large number of large sized air pockets, which resulting an increase in crispness (Shi et al., Citation2011). Garg and Singh (Citation2010) also reported that crispness increased with increase in temperature and decreased with increase in moisture content.
4. Conclusions
Based on the findings from this particular study, it is possible to obtain expanded product from sorghum/defatted soy meal flour mixture by extrusion cooking that have better sensory properties with nutritional advantages. Extruded products from sorghum and defatted soy meal flour blend can have some potential to solve the problem of malnutrition in its various forms that are mainly common among the people of the developing countries. A serving of 100 g of sorghum and soy blend extrudate produced from 10 and 20% soy meal flour proportion could provide about 29 and 36% of the recommended dietary allowance (RDA) for protein, respectively.
Acknowledgements
The authors are grateful to the Center of Research on Grain Quality, Processing and Technology Transfer (CRGQPTT), department of Food Science and Postharvest Technology, Haramaya University, Ethiopia, for supporting with laboratory facilities to this work.
Additional information
Funding
Notes on contributors
Solomon Abebaw Tadesse
Solomon Abebaw Tadesse is a PhD candidate in Food Engineering at the department of Chemical Engineering, Addis Ababa Institute of Technology, Addis Ababa University, Ethiopia. He has a strong research interest in the field of functional and nutraceutical foods, emerging food processing technologies, and novel ingredient identification and characterization from underutilized resources.
Prof. Geremew Bultosa Beri is currently working as a professor of Food Science/Food Chemistry at the department of Food Science and Technology, Botswana University of Agriculture and Natural Resource, Gaborone, Botswana. He has research experts in the area of food science/food chemistry, grain science and technology, postharvest handling, functional foods, starches, food nutrition, weaning foods development and value additions.
Dr. Eng. Solomon Abera is an assistant professor in the department of Food Technology and Process Engineering, and Food Science and Postharvest Technology, Haramaya University, Ethiopia. He has a great aspiration to work in the research area of agro-food engineering.
References
- AACC. (2000). Approved methods of the American association of cereal chemists. St. Paul, MN: Inc.
- Abioye, V. F., Ade-Omowaye, B. I. O., Babarinde, G. O., & Adesigbin, M. K. (2011). Chemical, physico-chemical and sensory properties of soy-plantain flour. African Journal of Food Science, 5(4), 176–19.
- Adedeji, A. A., Suhr, E., Bhadriraju, S., & Alavi, S. (2016). Drying characteristics of bean analog – A sorghum based extruded product. Journal of Food Processing and Preservation, 41, 2. doi:10.1111/jfpp.12856
- Adunni, A. O., & Olaposi, A. R. (2010). Effect of partially defatted soybean flour substitution on the proximate, pasting and sensory properties of banana flour. African Journal of Agricultural Research, 5(13), 1696–1700.
- Anton, A. A., Fulcher, R. G., & Arntfield, S. D. (2009). Physical and nutritional impact of fortification of corn starch-based extruded snacks with common bean (Phaseolus vulgaris L.) flour: Effects of bean addition and extrusion cooking. Food Chemistry, 113(4), 989–996. doi:10.1016/j.foodchem.2008.08.050
- Anuonye, J. C., Onuh, J. O., Egwim, E., & Adeyemo, S. O. (2010). Nutrient and antinutrient composition of extruded acha/Soybean blends. Journal of Food Processing and Preservation, 34, 680–691. doi:10.1111/(ISSN)1745-4549
- Asare, E. K., Sakyi-dawson, E., Asare, E. K., Sefa-dedeh, S., Afoakwa, E. O., & Budu, A. S. (2010). Response surface methodology for studying the effects of feed moisture and ingredient variations on the chemical composition and appearance of extruded sorghum-groundnut-cowpea blends. International Journal of Food Engineering, 6(6), 1–20. doi:10.2202/1556-3758.2025
- Basediya, A. L., Pandey, S., Shrivastava, S. P., Khan, K. A., & Nema, A. (2013). Effect of process and machine parameters on physical properties of extrudate during extrusion cooking of sorghum, horse gram and defatted soy flour blends. Journal of Food Science and Technology, 50(1), 44–52. doi:10.1007/s13197-011-0319-y
- Belton, P. S., & Taylor, J. R. N. (2004). Sorghum and millets : protein sources for Africa. Trends in Food Science & Technology, 15, 94–98. doi:10.1016/j.tifs.2003.09.002
- Brncic, M., Tripalo, B., Jezek, D., Semenski, D., Drvar, N., & Ukrainczyk, M. (2006). Effect of twin-screw extrusion parameters on mechanical hardness of direct-expanded extrudates. Sadhana, 31(5), 527–536. doi:10.1007/BF02715911
- Devi, N. L., Shobha, S., Tang, X., Shaur, S. A., Dogan, H., Alavi, S., … Alavi, S. (2013). Development of protein-rich sorghum-based expanded snacks using extrusion technology. International Journal of Food Properties ISSN:, 16(2), 263–276. doi:10.1080/10942912.2011.551865
- Dhingra, S., & Jood, S. (2002). Organoleptic and nutritional evaluation of wheat breads supplemented with soybean and barley flour. Food Chemistry, 77, 479–488. doi:10.1016/S0308-8146(01)00387-9
- Dicko, M. H., Gruppen, H., Traoré, A. S., Voragen, A. G. J., & van Berkel, W. J. H. (2006). Sorghum grain as human food in Africa : relevance of content of starch and amylase activities. African Journal of Biotechnology, 5(5), 384–395.
- Garg, S. K., & Singh, D. S. (2010). Optimization of extrusion conditions for defatted soy-rice blend extrudates. Journal of Food Science and Technology, 47(6), 606–612. doi:10.1007/s13197-010-0117-y
- Gbenyi, D. I., Nkama, I., & Badau, M. H. (2016). Modeling mineral profile of extruded sorghum bambara groundnut breakfast cereals. British Journal of Applied Science and Technology, 17(4), 1–14. doi:10.9734/BJAST/2016/28700
- Golob, P., Farrell, G., & Orchard, J. E. (2002). Crop post-harvest : Science and technology (Vol. 1). Black well: Black well science Ltd.
- Hegazy, H. S., El-bedawey, A. E. A., Rahma, E. H., & Gaafar, A. M. (2017). Effect of extrusion processs on nutritional, functional properties and antioxidant activity of germinated chickpea incorporated corn extrudates. American Journal of Food Science and Nutrition Research, 4(1), 59–66.
- Kumar, T. V. A., Samuel, D. V. K., Jha, S. K., & Sinha, J. P. (2015). Twin screw extrusion of sorghum and soya blends : A response surface analysis. Journal of Agricultural Science and Technology, 17, 649–662.
- Mason, W. R., & Hoseney, R. C. (1986). Factors affecting the viscosity of extrusion cooked wheat starch. Cereal Chemistry, 63(5), 436–441.
- Nwabueze, T. U. Ã. (2007). Effect of process variables on trypsin inhibitor activity (TIA), phytic acid and tannin content of extruded African breadfruit-corn-soy mixtures : A response surface analysis. LWT - Food Science and Technology, 40, 21–29. doi:10.1016/j.lwt.2005.10.004
- Odebode, F. D., Ekeleme, O. T., Ijarotimi, O. S., Malomo, S. A., Idowu, A. O., Badejo, A. A., … Fagbemi, T. N. (2018). Nutritional composition, antidiabetic and antilipidemic potentials of flour blends made from unripe plantain, soybean cake, and rice bran. Journal of Food Biochemistry, 42(4), 1–9. doi:10.1111/jfbc.12447
- Pelembe, L. A. M., Erasmus, C., & Taylor, J. R. N. (2002). Development of a protein-rich composite sorghum-cowpea instant porridge by extrusion cooking process. LWT - Food Science and Technology, 35, 120–127. doi:10.1006/fstl.2001.0812
- Perez, A. A., Drago, S. R., Carrara, C. R., Greef, D. M., De, Torres, R. L., & Gonzalez, R. J. (2008). Extrusion cooking of a maize/soybean mixture : Factors affecting expanded product characteristics and flour dispersion viscosity. Journal of Food Engineering, 87, 333–340. doi:10.1016/j.jfoodeng.2007.12.008
- Price, M. L., Van Scoyoc, S., & Butler, L. G. (1978). A critical evaluation of the vanillin reaction as an assay for tannin in sorghum grain. Journal of Agriculture and Food Chemistry, 26(5), 1214–1218. doi:10.1021/jf60219a031
- Shi, C., Shi, C., Wang, L., Wu, M., Adhikari, B., & Li, L. (2011). Optimization of twin-screw extrusion process to produce okara-maize snack foods using response surface methodology. International Journal of Food Engineering, 7(2), 1–24. doi:10.2202/1556-3758.1909
- Shogren, R. L., Hareland, G. A., & Wu, Y. V. (2006). Sensory evaluation and composition of spaghetti fortified with soy flour. Journal of Food Science, 71(6), 428–432. doi:10.1111/j.1750-3841.2006.00061.x
- Solomon, W. K. (2007). Effect of barrel temprature on rehydration kinetics of direct-expanded Tef flour breakfast cereal. Journal of Food Process Engineering, 31, 469–487. doi:10.1111/j.1745-4530.2007.00160.x
- Ströher, R., Stenzel, M., Pereira, N. C., & Zanin, G. M. (2012). Enzymatic extraction of protein from toasted and not toasted soybean meal. Procedia Food Science, 1, 463–469. doi:10.1016/j.profoo.2011.09.071
- Suksomboon, A., Limroongreungrat, K., Sangnark, A., & Thititumjariya, K. (2011). Effect of extrusion conditions on the physicochemical properties of a snack made from purple rice (Hom Nil) and soybean flour blend. International Journal of Food Science and Technology, 46, 201–208. doi:10.1111/j.1365-2621.2010.02471.x
- Taylor, J. R. N., & Dewar, J. (2001). Developments in sorghum food technologies. Advances in Food and Nutrition Research, 43, 218–264.
- Taylor, J. R. N., Schober, T. J., & Bean, S. R. (2006). Novel food and non-food uses for sorghum and millets. Journal of Cereal Science, 44, 252–271. doi:10.1016/j.jcs.2006.06.009
- Wheeler, E., & Ferrel, R. (1971). A method for phytic acid determination in wheat and wheat fractions. American Association of Cereal Chemists, 48, 312–320.