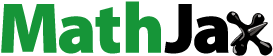
Abstract
Maize lethal necrosis (MLN) disease has emerged as a great threat of maize production in Ethiopia. Thus, field surveys were conducted to determine the spatial distribution and association of MLN disease with biophysical factors in Southern Ethiopia, during 2016 and 2017 main cropping seasons. A total of 240 maize fields were inspected across seven districts in the 2 years. Results showed that MLN disease was 100% prevalent in maize fields though the level of importance varied among districts. Arbaminch, Mihirab Arbaya, Bonkie, Derashie, Konso and Burjdi districts lowered disease severity by 1.46%, 4.13%, 11.38%, 13.40%, 9.24% and 30.82% when compared with Demba Gofa district, respectively. Also, severe weed and insect infestations were evident in the assessed farms. The association of disease severity with biophysical factors was assessed using a logistic regression model. District, NPS fertilization, cropping system, insect and weed infestation and insecticide spray frequency were significantly associated with disease severity in the multiple reduced variable model. High (>50%) disease severity had about four, three, and two times high probability of relationship with Demba Gofa, Arbaminch, and Mihirab Arbaya, Konso, Derashie and Bonkie districts, respectively. Similarly, 0 to 50 kg ha−1 NPS fertilization, sole cropping, high insect and weed infestation and none to once insecticide spray showed a high probability of association with disease severity. The results indicated that MLN disease is highly prevalent, severe and important in Southern Ethiopia. Therefore, weeding, insect vector control, NPS fertilization, intercropping, along with other agronomic practices, are recommended in the study areas and other related agro-ecologies.
PUBLIC INTEREST STATEMENT
Maize lethal necrosis is an emerging disease and looming threat of maize production and productivity in east Africa. The disease is reported from maize production belts of Ethiopia and is causing severe yield losses. However, lack of expertise and less attention to viral diseases enhanced the rapid distribution of the disease to many parts of the country, which calls for a timely response from stakeholders. But, to design appropriate and focused management schemes, information regarding factors that influence the intensity of the disease is a pre-requisite. In this study, therefore, the association of many factors with the disease epidemic has been studied. And, a disease epidemic was found highly and significantly associated with many biophysical factors studied. Thus, the results implied the need for designing effective and integrated pest management options that target the host, weed, insect vectors and their interactions.
Competing interests
The authors declare that they have no conflicting interests.
1. Introduction
Maize (Zea mays L.) is the third most important cereal crop next to wheat and rice worldwide (FAOSTAT, Citation2015). The crop is also the most important grain covering wider acres than other grains in sub-Saharan Africa (Smale, Byerlee, & Jayne, Citation2011), widely grown for food and cash in a broad range of environments (CIMMYT, Citation2014). In Ethiopia, maize is the most principal crop covering 2.14 million ha of land next to Teff [Eragrostis tef (3.02 million ha)]. Out of the total areas covered by maize, 7.85 million tons have been produced in the 2016/17 main cropping season (CSA, Citation2017). Similarly, 1.09 million tons of maize were produced from 0.32 million ha of land in southern Ethiopia. Comparatively, total annual maize production exceeds all other cereals in the area covered and a number of households involved, thus, implying that maize is one of the priority food security crops in Ethiopia (CSA, Citation2017).
In Ethiopia, maize is used as a staple food for human consumption, cash crop, feed for livestock and as raw material for industrial uses (CSA, Citation2017). The crop is the cheapest source of basic calories. It accounts for 17% of the per capita calorie intake, followed by sorghum (14%) and Teff (11%) (Demeke, Citation2012). Maize is also a cheaper source of protein (Rashid & Negassa, Citation2011). However, despite its nutritional and economic values, the national average productivity is only 3.4 t ha−1, which is far below than the world’s average yields of 5.6 t ha−1 (FAOSTAT, Citation2015). The productivity of the crop in the study areas is even lower (3.36 t ha−1) than the national average yield (CSA, Citation2017). Low maize yield is attributed to a combination of constraints that include, but not limited to, weeds, insect pests and diseases (Miano, Citation2014). Amongst the diseases, maize lethal necrosis (MLN) disease has emerged as the single most important production constraints in the country (Demissie et al., Citation2016).
The disease was first identified in Kenya in 2012 (Wangai et al., Citation2012), and subsequently in Rwanda (Adams et al., Citation2014), the Democratic Republic of Congo (Lukanda et al., Citation2014), and in the border districts of Uganda (ASARECA, Citation2013). In July 2014, the disease was detected from maize plants exhibiting severe yellowing and chlorotic mottle symptoms in Ethiopia (Mahuku et al., Citation2015a), signifying the presence of MLN disease in the country. The outbreak and rapid spread of MLN disease in east Africa has emerged as a big challenge to maize production and has significantly affected the productivity of the crop in the region. The disease can cause significant yield reduction, and loss of grain quality and food supplies (Ali & Yan, Citation2012). In highly affected regions, yield losses of 90–100% may occur (Adams et al., Citation2014) and in the East African countries, the disease has raised a major concern as it caused extensive to complete yield loss (Wangai et al., Citation2012). Recent surveys in some parts of Ethiopia have also confirmed up to 100% and 83% disease incidence and severity, respectively (Guadie, Knierim, Winter, Tesfaye, & Abraham, Citation2018b). This could imply that the disease is likely to be present in the major maize-growing areas of the country and often confused with other nutrient deficiency symptoms.
Maize lethal necrosis disease is caused by a synergistic interaction of Maize Chlorotic Mottle Virus (MCMV) and Sugarcane Mosaic Virus (SCMV) or other viruses such as Maize Dwarf Mosaic Virus (MDMV) or Wheat Streak Mosaic Virus (WSMV) (CIMMYT, Citation2013). In Africa, MLN disease symptoms are associated with MCMV and SCMV (Mahuku et al., Citation2015b). In Ethiopia, both MCMV and SCMV were found to cause MLND symptoms in 2014 (Mahuku et al., Citation2015a) either alone or in mixed infections (Guadie et al., Citation2018b). However, more recently, another newly emerging maize virus, Maize Yellow Mosaic Virus (MaYMV) is reported in Ethiopia (Guadie et al., Citation2018a). This could evidence a steadily changing virus situation in the country warning for an urgent response from all stakeholders to address the existing challenges.
Different production practices including weeding, pesticide application, insect vectors control, nutrient management and cropping systems, along with agroecology and cropping years could influence disease occurrence, development and level of crop damages. Thus, management of viral diseases should be directed at creating awareness among farmers, studying disease epidemiology, alternative hosts and screening for possible sources of resistance and the survival of the viruses to design an effective management strategy (Miano, Citation2014). Development and implementation of disease management in an integrated system against any disease is guided by precise and accurate information on the disease and factors that influence its development (Mudde et al., Citation2018). However, the existing body of knowledge reveals lack of comprehensive survey research to single out the association of factors influencing MLN disease in Ethiopia, except a few attempts made to characterize and identify causative viruses of the disease (Fentahun, Feyissa, Abraham, & Kwak, Citation2017; Guadie et al., Citation2018b; Mahuku et al., Citation2015a). The objectives of this study were, therefore, to determine (1) the spatial distribution, relative importance and severity of maize lethal necrosis disease in Southern Ethiopia and (2) the association of factors influencing emerging maize lethal necrosis disease in the study areas.
2. Materials and methods
2.1. Description of surveyed areas
Surveys of maize lethal necrosis disease, cropping systems, field management practices and other important factors were conducted in seven major maize-growing districts of southern Ethiopia during 2016 and 2017 main cropping seasons. The districts were selected based on their maize production potentials. The altitude of the selected study areas ranged from 846 to 2060 meters above sea level (m.a.s.l.). Monthly average temperatures and total rainfall of the districts during the cropping years were obtained from nearby meteorological stations and depicted in Figure . The areas surveyed and their respective geographic locations are shown in Figure . The survey was commenced starting from vegetative to hard doughing growth stages of maize.
2.2. Survey methodology and disease assessment
Maize fields were directly inspected and growers were interviewed to capture the status of the fields, the geographic and temporal distribution of MLN disease, and weeds and insect vectors supposed to harbor and vector MLN disease-causing viruses in each district. Briefly, maize fields were randomly taken at intervals of 5–6 km along accessible main and feeder roads. Within each selected field, four quadrats (2 m x 2 m) about 12 m apart were sampled by making diagonal moves. All plants in each quadrat were served as sampling units. Quadrats were set inside the field to avoid edge effects. Disease prevalence was determined as the percentage of maize fields with MLN disease symptoms over the total number of maize fields assessed in each district. Also, disease severity was recorded from 10 randomly selected plants within the quadrats by observing apparent symptoms of the target disease.
Disease severity was scored on a rating scale of 1 to 5, where 1 = no disease symptoms, 2 = fine chlorotic streaks/mottling on lower leaves, 3 = chlorotic mottling and mosaic throughout the whole plant, 4 = excessive chlorotic mottling, mosaic, plant necrosis, and/or dead heart, and 5 = dead plant and complete plant necrosis (Beyene et al., Citation2017). Disease severity scores were converted into percentage severity index (PSI) for analysis (Wheeler, Citation1969):
In addition to disease data, altitude ranges, crop growth stages, cropping systems, weeding practices, bean genotypes grown, sources of seed, crops grown for the last 2 years, fertilizer application and pest management practices were recorded for each field. Relative field location and altitude were recorded using a geographical positioning system. Weed management practices were recorded as good (any weed is removed or weed-free); intermediate (few weeds are present) and poor weeding (no weeding practiced and/or high weed infestation). Diseased maize samples were also collected to confirm the identity of the disease and causative viruses. Plant samples were selected on the basis of apparent symptoms of MCMV and SCMV infection. Detection of viruses in samples was performed using a double-antibody sandwich (DAS) ELISA procedure. The ELISA protocol was run separately on tissues from individual plants, and multiple plants were tested at each sampling location using procedures of Jiang, Meinke, Wright, Wilkinson, and Campbell (Citation1992).
2.3. Insect pest and weed species assessment
Insect pests and weed species were assessed by counting each species category within the quadrats through direct visual examination of farms. Count of insect pests was done from randomly selected plants within the quadrats and each individual insect pest recorded was added. Weed species recording and identification were taken from 4 m2 quadrat by direct counting of both the total and individual species based on their importance. The collected weed data were then summarized as relative abundance, relative dominancy, relative frequency and importance value following the methods of Shabbir and Bajwa (Citation2006).
2.4. Data analysis
Descriptive analysis was done to describe the distribution and relative importance of maize lethal necrosis disease across districts. Disease severity was classified into distinct groups of binomial qualitative data (Yuen, Citation2006). Contingency tables of the disease severity and independent variables were built to represent the bivariate distribution of the fields according to data classifications (Table ). Class boundaries (≤50 and >50%) were selected so that binary variable classes were set for disease severity. The association of mean disease severity with biophysical factors was analyzed using a logistic regression model (Yuen, Citation2006) using the SAS procedure of GENMOD (SAS, Citation2014). The importance of the independent variables was evaluated twice in terms of their effect on disease severity. First, the association of all the independent variables with MLN disease severity was tested in a single-variable model. Selected independent variables that have a significant association with disease severity were sequentially added to a reduced multiple-variable model (Yuen, Citation2006). Deviance reduction and odds ratios were computed for each independent variable as it was added to the reduced multiple-variable model. The deviance, the logarithm of the ratio of two likelihoods was used to compare the single- and multiple-variable models. The difference between the likelihood ratio tests (LRTs) was used to examine the importance of the variable and was tested against the χ2 value (McCullagh & Nelder, Citation1989).
Table 1. Categorization of variables and independent variable x disease contingency table for logistic regression analysis of maize lethal necrosis disease severity (%) in seven districts (n = 240) of Southern Ethiopia, during the 2016 and 2017 main cropping seasons
3. Results
3.1. General features of surveyed fields
Maize lethal necrosis disease surveys were undertaken from 240 maize fields in seven major maize-growing districts of Southern Ethiopia during the 2016 and 2017 main cropping seasons. The surveyed areas are characterized by different ago-ecologies, cropping systems, field management practices (nutrient management) and land uses amongst other characteristic features. The altitude ranged from 1101 in Arbaminch areas to more than 3500 m.a.s.l. in Bonke district. Field sizes of 0.21 (Derashe district) to 0.35 ha (Demba Gofa district) were recorded across districts. The highest maize population density was observed from farms surveyed in Demba Gofa district within a given quadrat of 4 m2.
The most common maize varieties grown in these areas included BH140, BH540, BH660, BH661, Melkasa 2, Melkasa 4, Melkasa 6Q, Shala PH, Shone and PH3253. About 48.75% and 51.25% of maize fields were sole and intercropped, respectively. Component crops in the intercropping system were mainly common bean, sorghum, Teff, mango, banana, papaya, pigeon pea, Moringa sp., sunflower and vegetables. Cropping system was dominated by maize-bean intercrops in row planting pattern in most of the districts. Planting was done from June to September; however, sowing time differed in each agro-ecological area depending on response to the onset of precipitation. In this regard, sowing was made in the month of July in Demba Gofa, Bonke, Derashe, Konso and Burjdi districts, while late August to September was found ideal sowing time in Arbaminch and Mihirab Abaya areas. During the survey periods, vegetative to dough growth stages were encountered.
Farmers often applied Urea (N) and NPS blended fertilizers at a less or recommended rate of 100 kg ha−1. With respect to seed source, agricultural office of each respective district supplied improved maize varieties prior to sowing time, and most of the surveyed fields were covered with improved maize varieties. However, farmers were observed to follow poor agricultural practices during field management. For instance, only 18.75% of maize fields were weed-free; while the rest maize fields were severely weed-infested (Figure ). Accordingly, weeds recorded during the survey were mostly members of the Poaceae family in terms of relative frequency, abundance, dominance and importance. Inspected farms were also infested by Thrips, Aphids, Stalk borer and Fall armyworm. Thrips were also found infesting the nearby onion farms.
Figure 3. Linear regression relating maize lethal necrosis disease severity (%) and weed population density (m−2) in maize fields in Southern Ethiopia, during the 2016 and 2017 main cropping seasons.
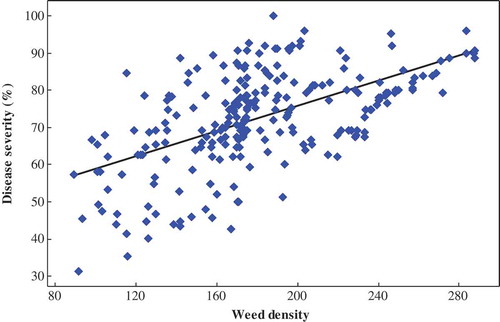
Symptoms conspicuous for virus diseases that were found on leaves mostly those consisted of chlorosis, mosaic and mottling, chlorotic streaks, “dead heart” symptoms, shortened internodes, shortened male inflorescence, premature drying of leaf sheath, leaf necrosis, malformed or lacking ear sets, bright chlorosis, bleaching and stunting symptoms were typical for MLN disease. Maize lethal necrosis disease severity varied from 40% in Burji district to 100% in Demba Gofa and Mihirab Abaya districts. To control MLN disease damages, farmers used to undertake cultural and insecticide control (Malathion 25% EC at the rate of 1 L ha−1 with 200 L of water) options. Insecticide applications were made with different spray frequencies ranging from none to more than twice.
3.2. Maize lethal necrosis disease distribution, severity and relative importance
Maize samples showing chlorotic mottling to severe stunting symptoms were tested positive to the maize lethal necrosis disease (data not shown). And, the disease was prevalent in all maize fields, irrespective of maize genotypes grown and other factors. However, different levels of disease importance were noted among districts (Table ). Mean disease severity was higher in 2016 (75.85%) than in 2017 (69.84%). The highest mean disease severity of 78.69% was recorded at Demba Gofa, followed by Arbaminch (77.69%) and Mihirab Arbaya (75.06%) districts. Conversely, the lowest mean disease severity of 54.44% was recorded at Burjdi district in the two cropping years. Accordingly, productivity of maize at Demba Gofa suffered reductions from the disease of 30.82%, 13.40%, 11.38%, 9.24%, 4.13% and 1.46% compared with maize farms at Burjdi, Derashie, Bonkie, Konso, Mihirab Arbaya and Arbaminch districts, respectively.
Table 2. Severity (mean ± SE) of maize lethal necrosis disease for different independent variables in the 2016 and 2017 main cropping seasons, Southern Ethiopia
Of maize fields assessed, farms inspected at >2000 m.a.s.l. (24.44%); covered with pioneer hybrid and/or Melkassa composite maize genotypes (15.19%) and N (19.63%) and NPS (21.55%) fertilizers at a rate of 51–100 kg ha−1 in an intercropped system (17.91%) reduced disease severity as compared with their respective variable classes in the 2 years. Similarly, maize planting made during July (24.64%); at vegetative and/or milking and/or doughing growth stages (15.73%) and sprayed with insecticides two and more times (28.76%) also reduced disease severity when compared with late planting (September), silking growth stage and non-sprayed fields, respectively. However, fields covered with farm-saved maize seeds (81.7%), severely infested by weeds (86.59%) and insects (87.94%) recorded the highest mean disease severity compared with their counterpart classes. Among severely insect-infested farms, aphid and thrip infestations apparently predisposed maize plants for high MLN disease epidemics, which recorded up to 78.99% on average, along with other factors (Table ).
3.3. Association of maize lethal necrosis disease with biophysical factors
The association of all independent variables with MLN disease severity is presented in Table . Among the biophysical factors, district, year, altitude, genotype, seed source, NPS and N fertilization, planting date, growth stage, cropping system, insect infestation, insecticide spray frequency and weed infestation showed very highly significant (P < 0.0001) association with disease severity when entered into the logistic model as a single variable. However, when all variables entered last into the model, district, NPS fertilization, growth stage, cropping system, insect infestation, insecticide spray frequency and weed infestation remained significantly associated with disease severity. Meanwhile, others lost their importance when entered into the reduced variable model.
Table 3. Logistic regression model for maize lethal necrosis disease severity (%) and likelihood ratio test on independent variables in Southern Ethiopia, during the 2016 and 2017 main cropping seasons
Amongst the independent variables, district was the most important variable in its association with disease severity when entered first and last into the logistic model (χ2 = 497.25 and 134.17, 6 df). District, NPS fertilization, cropping system, insect infestation, insecticide spray frequency and weed infestation were tested in the reduced variable model and analysis results are presented in Table . The output from the reduced model indicated the importance of all the variables and variable classes tested. The parameter estimates and standard error and odds ratio are also presented in Table . The probability of the lowest (≤50%) disease severity was associated with Burjdi district, NPS fertilization at a recommended rate, blistering to dough growth stages, intercropping systems, low to medium level of insect infestation, good weeding and twice and more times insecticide spray frequencies in the cropping seasons.
Table 4. Analysis of deviance, natural logarithms of odds ratio and standard error of maize lethal necrosis disease severity (%) and likelihood ratio test on independent variables in reduced regression model in Southern Ethiopia, during the 2016 and 2017 main cropping seasons
Conversely, high (>50%) disease severity was highly associated with Demba Gofa, Arbaminch, Mihirab Arbaya, Konso, Derashie and Bonkie districts, zero to 1–50 kg ha−1 NPS fertilization, sole cropping, high insect infestation, none to once insecticide spray frequency and poor weeding practice. Maize production at Arbaminch and Demba Gofa districts showed a 3 and 4 times high probability of association with high disease severity when compared with maize farming at Burjdi district, respectively. Similarly, Mihirab Arbaya, Bonkie, Derashie and Konso districts each recorded twice the high probability of relationship with high disease severity compared with Burjdi district. Absence of NPS fertilization also demonstrated one and half times greater probability of association with high disease severity than other rates of NPS fertilization of maize farms (Table ).
4. Discussion
Maize lethal necrosis disease continues to severely reduce production and productivity of maize and threatens food security in Ethiopia. It is supposed to cause an estimated yield loss of up to 65% in the study areas, which is the most affected region of the country (Demissie et al., Citation2016). Symptoms observed during the survey periods that included chlorotic mottling, leaf necrosis, yellow streaks, leaf deformation and premature drying of leaf sheath are all similar to the typical symptoms of MLN diseased plants reported in various areas including Ethiopia (Adams et al., Citation2014; Guadie et al., Citation2018a; Mahuku et al., Citation2015a; Nelson, Brewbaker, & Hu, Citation2011).
Representative symptomatic leaf samples of maize all tested positive for MCMV and SCMV, and the disease. This could confirm that MLN disease is widespread across the studied areas, irrespective of the factors influencing disease epidemiology, and vector and alternative host population dynamics in the 2 years. Studies by Guadie et al. (Citation2018b), Fentahun et al. (Citation2017) and Demissie et al. (Citation2016) also reported the widespread presence of both MCMV and SCMV alone and in mixed infections in major maize-growing areas of the country. They also noted the association of MCMV and SCMV with MLN disease in the studied areas.
District wise comparisons, however, revealed that productivity of maize at Demba Gofa suffered from MLN disease damage levels of 30.82%, 13.40% and 11.38% compared with maize farms at Burjdi, Derashie and Bonkie districts in the 2 years, respectively. The temporal analysis also showed that in the 2017 cropping year disease severity was lowered by 7.92% compared with 2016. These spatio-temporal differences in disease epidemics might be attributed to variability in weather variables (Figure ) and altitudinal ranges of assessed farms along with other factors (Table ). In this regard, Mudde et al. (Citation2018) indicated that development of MLN disease is influenced by warm temperatures ranging from 22°C to 24°C and low- to mid-latitudes (Guadie et al., Citation2018b). Also, the study confirmed that altitudinal ranges of >2000 m.a.s.l. reduced disease severity by 24.44% compared with lower altitudes, which were characterized by warm temperatures.
Similarly, Guadie et al. (Citation2018b) reported closely 100% disease incidence in major maize-growing areas of the country, which are located at low- and mid-altitude ranges. On the contrary, De Groote, Oloo, Tongruksawattana, and Das (Citation2016) noted a progressive decrease in disease outbreak towards highland areas. Moreover, MLN disease development is positively correlated with rainfall, and the disease is highly favored by warm temperatures and high relative humidity (Mudde et al., Citation2018). Humid regions with high precipitation are found in the most suitable areas for wild grasses that harbor MCMV and SCMV, which are responsible for MLN disease development in Kenya (Kusia, Citation2014). Similarly, surveyed districts received relatively higher average rainfall in 2016 than in 2017. Warm environments also favor insect population, which are vectors of MLN disease-causing viruses (Guadie et al., Citation2018b; Kiyyo, Citation2015).
Insect vectors are responsible for transmitting of MLN disease-causing viruses and enhance disease epidemics across maize fields (Brault, Uzest, Monsion, Jacquot, & Blanc, Citation2010; Demissie et al., Citation2016; Mahuku et al., Citation2015b; Namikoye, Kariuki, Kinyua, Githendu, & Kasina, Citation2018; Zhang, Rao, Gaur, Ruan, & Singh, Citation2008; Zhao, Ho, Wu, He, & Li, Citation2014). In this study, four major insect species, namely thrips, aphids, stalk borer and fall armyworm were found infesting maize. Of course, thrips and aphids dominated farms which recorded high (83.6–84.14%) disease severity in both years. Also, thrips were found even infesting neighboring onion farms. Likewise, Demissie et al. (Citation2016) reported a high population of aphids, thrips and beetles, which could vector the disease and indicated their presence to the spread of the disease in major maize-growing areas.
The presence of the MCMV and SCMV along with their potential vectors in the surveyed areas confirms the potential of the spread of the disease among districts and across regions. Studies indicated that the viruses causing MLN disease are transmitted by insect vectors which include thrips and beetles for MCMV and aphids for SCMV and MDMV (Cabanas, Watanabe, Higashi, & Bressan, Citation2013; Nelson et al., Citation2011; Wangai et al., Citation2012). Pertinent literature also indicated that SCMV is primarily transmitted by several aphid species (Brault et al., Citation2010; Wangai et al., Citation2012). On the other hand, MCMV is transmitted by several insect vectors including thrips in the genus Frankliniella (Cabanas et al., Citation2013; Namikoye et al., Citation2018; Zhao et al., Citation2014) and beetles (Braidwood et al., Citation2017; Nelson et al., Citation2011). These MLN disease-causing viruses transmitting insect vectors are also endemic in East Africa (Cabanas et al., Citation2013; De Groote et al., Citation2016).
Management of MLN disease is very difficult since the disease is caused by a combination of viruses and the involvement of two or more different insect vectors. Therefore, several control options should be considered including a maize-free period, insecticidal control of vectors and roguing of diseased plants (Jiang et al., Citation1992). In addition, weed control (Karanja, Derera, Gubba, Mugo, & Wangai, Citation2018), variation in planting time and cropping systems or a combination of options are currently in use in east Africa (Braidwood et al., Citation2017). Growers used to apply synthetic insecticides either once or twice and more to control insect vectors and subsequent development of MLN disease in the studied areas. Of which, frequent application of insecticides reduced disease severity by 28.76% in comparison with none sprayed fields. Therefore, chemical products can be used to control vectors that transmit and spread those viruses responsible for MLN disease (Fatma, Tileye, & Ndakidemi, Citation2016).
In this regard, Nelson et al. (Citation2011) reported effective control of vectors that transmit MCMV and SCMV viruses through a regular foliar spray of insecticides in Hawaii. Other studies also concluded that the corn leaf aphids can be controlled by the use of systemic insecticides and these insecticides have the capability to sustain populations of beneficial insects and reduce aphid problems (Tang, Wu, Ali, & Ren, Citation2013). Moreover, granular or spray formulated applications are recommended to manage aphids, rootworms, stem borers, mites and thrips that transmit MLN disease-causing viruses (Tropical Pesticides Research Institute [TPRI], Citation2011). Epidemiological modelling also suggests that clean seed and pesticides to control vectors will attain good MLN disease control results, which is suitable for larger farms (Hilker et al., Citation2017). However, there is no clear evidence whether these vectors are facilitating the transmission of MCMV and SCMV in Ethiopia.
On the other hand, cropping systems are known to influence population dynamics of insect vectors and development of MLN disease epidemics in maize. This study found that intercropping systems lowered disease severity by 17.9% compared with sole crop cultures, which recorded up to 79.81% disease severity. A significant number of evaluation trials also reported disease reductions in intercropped versus monocropped systems (Boudreau, Citation2013; Gopal, Muniyappa, & Jagadeeswar, Citation2010; Ramkat, Wangai, Ouma, Rapando, & Lelgut, Citation2008). For instance, intercropping increases spatial separation, imposes a physical barrier between plants, modifies the micro-climate and influences color, smell and insect vision in which pest or disease development is affected (Potts, Citation1990). Intercropping also allows for the possible exploitation of diversity so as to optimize beneficial effects and reduce disease development (Altieri, Ponti, & Nicholls, Citation2005), pest outbreaks (Zehnder et al., Citation2007), and weeds that could serve as alternate hosts (Hauggaard-Nielsen, Ambus, & Jensen, Citation2001).
Namikoye et al. (Citation2018) indicated that sole maize crops recorded higher MLN disease incidence than maize with companion crops that reduced the level of corn thrips and aphid infestations. These authors also reported a highly significant negative correlation between corn leaf aphid infestations on companion crops and corn thrips infestations on maize. This was because of the presence of component crops in the system that reduced foraging sites for insect vectors (Nelson et al., Citation2011) and feeding on non-host crops might have caused the insect to reduce or lose the inoculum required to establish infection (Comstock & Lentini, Citation2002). Karungi, Adipala, Nampala, Ogenga-Latigo, and Kyamanywa (Citation2000) had reported similar results where fewer flower thrips were observed on pepper and cowpea intercrop as compared with the maize monocrops.
In this study, poorly weeded farms recorded the highest (86.59%) disease severity, which is 37.22% higher than for weed-free farms. Many weed species in the Poaceae family were observed, which established a positive relationship with disease severity (Figure ). The presence of weeds do have an avoidable effect on disease development and insect infestations. This might be partly due to reduced crop vigor as a result of intense competition for available resources, which in turn could predispose the crop to diseases (Yimer et al., Citation2018). Thus, good field sanitation such as weed control eliminates alternative hosts for the potential buildup of insect vectors and viral load (Trigiano, Windham, & Windhan, Citation2008; Wangai et al., Citation2012) and subsequently reducing MLN disease (Jones, Citation2018). Otherwise, lack of proper weed management practices could have increased maize susceptibility and also aided in offering alternative hosts to MCMV and SCMV (Karanja et al., Citation2018). In this study, only 18.75% of assessed maize farms were weed-free, which found to increase disease severity.
The host range for both SCMV and MCMV is limited to members of the Gramineae family with maize and sugarcane being the natural hosts of MCMV and SCMV, respectively (Scheets, Citation2004). However, many species of the Poaceae family are claimed to be alternative hosts of MLN disease-causing viruses and many of these grasses are also tested positive for either both viruses or single virus (Braidwood et al., Citation2017; Kusia, Citation2014; Mariki, Citation2017; Nelson et al., Citation2011; Scheets, Citation2004). This reaffirms our assertion that the presence of weeds could serve as a reservoir of MCMV and SCMV for maize plants in severely affected districts. In line with this finding, Kusia (Citation2014) clearly reported that maize, wild grasses, domesticated grasses and crop cereals were found to host MCMV and SCMV either singly or in combination. However, further studies are required to confirm the role of weeds as alternative hosts of MCMV, SCMV and others causing MLN disease in major maize-growing regions of Ethiopia.
The study also recognized the importance of NPS soil fertilization as part of the crop management options to reduce MNL disease epidemic. As a general principle, optimal fertility of any crop reduces plant stress, improves physiological resistance and decreases disease risks (Dordas, Citation2008; Veresoglou, Barto, Menexes, & Rillig, Citation2013). However, only 37.5% of surveyed farms received optimum (up to 100 kg ha−1) NPS fertilizer applications, whereas, about 62.5% of the inspected farms obtained an insufficient dose of 0 to ≤50 kg ha−1 of fertilizer application. Thus, optimum fertilization reduced disease severity by 21.55% in both cropping seasons. Also, Mariki (Citation2017) recommended planting of maize, on the onset of the main rainy season, with fertilizer application to boost plant vigor. Application of fertilizer and manures also boosts crop vigor against MLN disease and the vectors, and was recommended as a protection option (Wangai et al., Citation2012).
The logistic regression model demonstrated that district, crop growth stages, NPS fertilizer application, insect infestation, insecticide spray frequency, cropping systems and weed infestation were associated with MLN disease severity and found important. These variables had significant contributions to the development of epidemics alone or in combination. The model quantified the relative importance of independent variables/variable classes, implying that an MLN disease epidemic was the function of these variables. Some of the variables were more important and tend to reduce or enhance MLN disease development than others (Tables and ).
5. Conclusions
The study concluded that MLN disease is widely distributed and is an important maize production constraint in the study areas. However, disease distribution and relative importance varied among districts. The regression model analysis identified that district (except Burjdi), none to low dose NPS fertilization, sole cropping system, high insect infestation, poor weed management and none to once insecticide spray frequency had a high association with disease severity and significantly contributed to the dynamics of the epidemic of the disease. The results implied the need for developing and implementing integrated pest management systems targeting hosts, weeds, insect vectors and their interactions in the affected areas and other related agro-ecologies. The management option should also consider optimal fertilization of the soil and other virus transmission mechanisms. However, a comprehensive study should be undertaken to identify alternative hosts that harbor MLN disease-causing viruses and to designate whether thrips and aphids or other insect species are responsible for viral transmission under the maize production systems of Ethiopia.
Acknowledgements
The authors would like to acknowledge the Southern Agricultural Research Institute, Ethiopia for financing the study. We are also very thankful to toiled growers of maize for their very keen support during data collection in the study areas.
Additional information
Funding
Notes on contributors
Habtamu Terefe
Habtamu Terefe (PhD) is research and teaching staff of the School of Plant Sciences of Haramaya University, Ethiopia. He has seasoned experiences of undertaking comprehensive survey studies to understand the interaction and association of different biophysical factors to reduce or enhance plant disease epidemics. Dr. Habtamu Terefe has designed and executed many epidemiological studies under both controlled and natural conditions. He appreciates biopesticides and encourages subsistence farmers to exploit the integrated potential of cultural practices to manage plant diseases and sustain productivity in the face of climate dynamics. He is involved in many national and international projects focusing on crop protection and climate smart crop production systems, along with different teams.
References
- Adams, I. P., Harju, V. A., Hodges, T., Hany, U., Skelton, A., Rai, S., … Boonham, N. (2014). First report of maize lethal necrosis disease in Rwanda. New Disease Reports, 29, 22. doi:10.5197/j.2044-0588.2014.029.022
- Ali, F., & Yan, J. (2012). Disease resistance in maize and the role of molecular breeding in defending against global threat. Journal of Integrative Plant Biology, 54, 134–20. doi:10.1111/j.1744-7909.2012.01105.x
- Altieri, M. A., Ponti, L., & Nicholls, C. (2005). Enhanced pest management through soil health: Toward a belowground habitat management strategy. Biodynamics, 33–40.
- ASARECA. (2013). Workshop to develop a strategic plan for maize lethal necrosis disease for eastern and central Africa. Nairobi, Kenya. Retrieved from http://www.ndrs.org.uk/article.php?id=029022
- Beyene, Y., Gowda, M., Suresh, L. M., Mugo, S., Olsen, M., Oikeh, S. O., … Prasanna, B. M. (2017). Genetic analysis of tropical maize inbred lines for resistance to maize lethal necrosis disease. Euphytica, 213–224). doi: 10.1007/s10681-017-2012-3
- Boudreau, M. A. (2013). Diseases in intercropping systems. Annual Review of Phytopathology, 51, 499–519. doi:10.1146/annurev-phyto-082712-102246
- Braidwood, L., Quito-Avila, D. F., Cabanas, D., Bressan, A., Wangai, A., & Baulcombe, D. C. (2017). Maize chlorotic mottle virus exhibits low divergence between differentiated regional subpopulations. Scientific Reports, 8, 1173. doi:10.1038/s41598-018-19607-4
- Brault, V., Uzest, M., Monsion, B., Jacquot, E., & Blanc, S. (2010). Aphids as transport devices for plant viruses. Compets Rendus Biologies, 333, 524–538. doi:10.1016/j.crvi.2010.04.001
- Cabanas, D., Watanabe, S., Higashi, C. H. V., & Bressan, A. (2013). Dissecting the mode of maize chlorotic mottle virus transmission (Tombusviridae: Machlomovirus) by Frankliniella williamsi (Thysanoptera: Thripidae). Journal of Economic Entomology, 106, 16–24. doi:10.1603/EC12056
- CIMMYT. (2013). Maize lethal necrosis (MLN) disease in Kenya and Tanzania: Facts and actions. Retrieved from https://www.rairarubiabooks.com/related-pdf-maize-lethal-necrosis-disease-mlnd-in-kenya.html.
- CIMMYT. (2014). Improved maize varieties and poverty in rural Ethiopia. Standing Panel on Impact Assessment (SPIA). Brief Number 45. CGIAR Independent Science and Partnership Council (ISPC) Secretariat, FAO. Rome, Italy. Retrieved from http://ispc.cgiar.org & http://impact.cgiar.org.
- Comstock, J. C., & Lentini, R. S. (2002). Sugarcane mosaic virus disease. In H. Sandhu (Ed.), Sugarcane Handbook. Gainesville: University of Florida/IFAS extension. Retrieved from http://edis.ifas.ufl.edu/
- CSA. (2017). Agricultural sample survey, 2016/2017. Report on area and production of crops (private peasant holdings, main season) (Central Statistical Agency, No. 584.1). Ethiopia: Addis Ababa.
- De Groote, H., Oloo, F., Tongruksawattana, S., & Das, B. (2016). Community-survey based assessment of the geographic distribution and impact of maize lethal necrosis (MLN) disease in Kenya. Crop Protection, 82, 30–35. doi:10.1016/j.cropro.2015.12.003
- Demeke, M. (2012). Analysis of incentives and disincentives for maize in Ethiopia (Technical notes series, MAFAP). Rome: FAO. doi:10.1094/PDIS-11-11-0999-PDN
- Demissie, G., Deressa, T., Haile, M., Bogale, G., Dida, M., Chibsa, T., … Azmach, G. (2016). Prevalence, distribution and impact of maize lethal necrosis disease (MLND) in Ethiopia. Pest Management Journal of Ethiopia, 18, 37–49.
- Dordas, C. (2008). Role of nutrients in controlling plant diseases in sustainable agriculture: A review. Agronomy for Sustainable Development, 28(1), 33–46. doi:10.1051/agro:2007051
- FAOSTAT. (2015). FAO statistical pocketbook, world food and agriculture. Rome, Italy: Food and Agriculture Organization of the United Nations.
- Fatma, H. K., Tileye, F., & Ndakidemi, P. A. (2016). Insights of maize lethal necrotic disease: A major constraint to maize production in East Africa. African Journal of Microbiology Research, 10(9), 271–279. doi:10.5897/AJMR2015.7534
- Fentahun, M., Feyissa, T., Abraham, A., & Kwak, H. R. (2017). Detection and characterization of Maize chlorotic mottle virus and Sugarcane mosaic virus associated with maize lethal necrosis disease in Ethiopia: An emerging threat to maize production in the region. European Journal of Plant Pathology, 149, 1011–1017. doi:10.1007/s10658-017-1229-2
- Gopal, K., Muniyappa, V., & Jagadeeswar, R. (2010). Management of peanut bud necrosis disease in groundnut through intercropping with cereal and pulse crops. Achives of Phytopathology and Plant Protection, 43, 883–891. doi:10.1080/03235400802075666
- Guadie, D., Abraham, A., Tesfaye, K., Winter, S., Menzel, W., & Knierim, D. (2018a). First report of maize yellow mosaic virus infecting maize (Zea mays) in Ethiopia. Plant Disease, 102(5), 1044. doi:10.1094/PDIS-08-17-1290-PDN
- Guadie, D., Knierim, D., Winter, S., Tesfaye, K., & Abraham, A. (2018b). Survey for the identification and geographical distribution of viruses and virus diseases of maize (Zea mays L.) in Ethiopia. European Journal of Plant Pathology. doi:10.1007/s10658-018-1568-7
- Hauggaard-Nielsen, H., Ambus, P., & Jensen, E. S. (2001). Interspecific competition, N use and interference with weeds in pea-barley intercropping. Field Crops Research, 70(2), 101–109. doi:10.1016/S0378-4290(01)00126-5
- Hilker, F. M., Allen, L. J. S., Bokil, V. A., Briggs, C. J., Feng, Z., Garrett, K. A., … Cunniffe, N. J. (2017). Modelling virus coinfection to inform management of maize lethal necrosis in Kenya. Analytical and Theoretical Plant Pathology, 107(10), 1095–1108.
- SAS Institute. (2014). SAS/STAT user’s guide, version 9.3. Cary NC: SAS Institute Inc.
- Jiang, X. W., Meinke, L. J., Wright, R. J., Wilkinson, D. R., & Campbell, J. E. (1992). Maize chlorotic mottle virus in Hawaiian-grown maize: Vector relations, host range and associated viruses. Crop Protection, 11, 248–254. doi:10.1016/0261-2194(92)90045-7
- Jones, D. (2018). Early season maize lethal necrosis disease (MLND) and fall armyworm update. Thinking agronomy newsletter. Retrieved from https://cropnuts.com/maize-lethal-necrosis-disease/.
- Karanja, J., Derera, J., Gubba, A., Mugo, S., & Wangai, A. (2018). Response of selected maize inbred germplasm to maize lethal necrosis disease and its causative viruses (sugarcane mosaic virus and maize chlorotic mottle virus) in Kenya. Open Agriculture Journal, 12, 215–225. doi:10.2174/1874331501812010215
- Karungi, J., Adipala, E., Nampala, P., Ogenga-Latigo, M. W., & Kyamanywa, S. (2000). Pest management in cowpea. Part 3. Quantifying the effect of cowpea field pests on grain yields in eastern Uganda. Crop Protection, 19, 343–347. doi:10.1016/S0261-2194(00)00027-2
- Kiyyo, J. G. (2015). Developing maize hybrids resistant to maize lethal necrosis disease from diverse maize inbred lines in Tanzania. Master’s thesis. Sokoine University of Agriculture, Morogoro, Tanzania.
- Kusia, E. S. (2014). Characterization of Maize chlorotic mottle virus and Sugarcane mosaic virus causing maize lethal necrosis disease and spatial distribution of their alternative hosts in Kenya (Master’s thesis). Institute for Basic Sciences Technology and Innovation, Pan African University.
- Lukanda, M., Owati, A., Ogunsanya, P., Valimunzigha, K., Katsongo, K., Ndemere, H., & Kumar, P. L. (2014). First report of maize chlorotic mottle virus infecting maize in the Democratic Republic of the Congo. Plant Disease, 98(10), 1448. doi:10.1094/PDIS-05-14-0484-PDN
- Mahuku, G., Lockhart, B. E., Wanjala, B., Jones, M. W., Kimunye, J. N., Stewart, L. R., … Redinbaugh, M. G. (2015b). Maize lethal necrosis (MLN), an emerging threat to maize-based food security in sub-Saharan Africa. Phytopathology, 105(7), 956–965. doi:10.1094/PHYTO-12-14-0367-FI
- Mahuku, G., Wangai, A., Sadessa, K., Teklewold, A., Wegary, D., Ayalneh, D., … Prasannal, B. M. (2015a). First report of Maize chlorotic mottle virus and maize lethal necrosis on maize in Ethiopia. Plant Disease, 99, 1870. doi:10.1094/PDIS-04-15-0373-PDN
- Mariki, A. (2017). Distribution of maize lethal necrosis disease, its causal viruses and alternative hosts in the north- central regions of Tanzania (Master’s thesis). Makerere University, Uganda.
- McCullagh, P., & Nelder, J. A. (1989). Generalized linear models (2nd ed.). London: Chapman and Hall.
- Miano, D. W. (2014). Maize lethal necrosis disease: A real threat to food security in the eastern and central Africa region. Nairobi, Kenya. Retrieved from http://www.nuruinternational.org
- Mudde, B., Olubayo, F. M., Miano, D. W., Asea, G., Kilalo, D. C., Kiggundu, A., … Adriko, J. (2018). Distribution, incidence and severity of maize lethal necrosis disease in major maize growing agro-ecological Zones of Uganda. Journal of Agricultural Science, 10(6), 72–85. doi:10.5539/jas.v10n6p72
- Namikoye, E. S., Kariuki, G. M., Kinyua, Z. M., Githendu, M. W., & Kasina, M. (2018). Cropping system intensification as a management method against vectors of viruses causing maize lethal necrosis disease in Kenya. East African Agriculture and Forestry Journal. doi:10.1080/00128325.2018.1456298
- Nelson, S., Brewbaker, J., & Hu, J. (2011). Maize chlorotic mottle virus. Plant Disease, 79, 1–6.
- Potts, M. J. (1990). Influence of intercropping in warm climates on pests and diseases of potato, with special reference to their control. Field Crops Research, 25, 133–144. doi:10.1016/0378-4290(90)90078-P
- Ramkat, R. C., Wangai, A. W., Ouma, J. P., Rapando, P. N., & Lelgut, D. K. (2008). Cropping system influences Tomato spotted wilt virus disease development, thrips population and yield of tomato (Lycopersicon esculentum). Annals of Applied Biology, 153, 373–380. doi:10.1111/aab.2008.153.issue-3
- Rashid, S., & Negassa, A. (2011). Policies and performance of Ethiopian cereal markets (IFPRI/EDRI, Ethiopia Strategy Support Program II (ESSP II) ESSP II Working Paper No. 21).
- Scheets, K. (2004). Maize chlorotic mottle: Viruses and virus diseases of Poaceae (Gramineae). Paris: National Institute of Agronomic Research.
- Shabbir, A., & Bajwa, R. (2006). Distribution of parthenium weeds (Parthenium hysterophorus L.): An alien invasive weed species threatening the biodiversity of Islamabad. Weed Biology and Management, 6, 89–95. doi:10.1111/j.1445-6664.2006.00202.x
- Smale, M., Byerlee, D., & Jayne, T. (2011). Maize revolutions in Sub-Saharan Africa (World Bank Policy Research Working Paper, No. 5659). Retrieved from http://documents.worldbank.org/curated/en/475801468209965095/pdf/WPS5659.pdf.
- Tang, L., Wu, J., Ali, S., & Ren, S. (2013). Establishment of the baseline toxicity data to different insecticides for Aphis Craccicirora Koch and R. maidis (Fitch). Homoptera: Aphididal) by glass tube residue film technique. Pakistan Journal of Zoology, 45(2), 411–415.
- TPRI. (2011). List of registered pesticides in Tanzania. Arusha, Tanzania: Tropical Pesticides Research Institute.
- Trigiano, R. N., Windham, M. T., & Windhan, A. S. (2008). Plant pathology, concepts and laboratory exercises (2nd ed.). London: Taylor and Francis.
- Veresoglou, S. D., Barto, E. K., Menexes, G., & Rillig, M. C. (2013). Fertilization affects severity of disease caused by fungal plant pathogens. Plant Pathology, 62(5), 961–969. doi:10.1111/ppa.2013.62.issue-5
- Wangai, A. W., Redinbaugh, M. G., Kinyua, Z. M., Miano, D. W., Leley, P. K., Kasina, M., … Jeffers, D. (2012). First report of Maize chlorotic mottle virus and maize lethal necrosis in Kenya. Plant Disease, 96(10), 1582–1583. doi:10.1094/PDIS-06-12-0576-PDN
- Wheeler, B. J. (1969). An introduction to plant diseases. London: John Wiley and Sons, Ltd.
- Yimer, S. M., Ahmed, S., Fininsa, C., Tadesse, N., Hamwieh, A., & Cook, D. R. (2018). Distribution and factors influencing chickpea wilt and root rot epidemics in Ethiopia. Crop Protection, 106, 150–155. doi:10.1016/j.cropro.2017.12.027
- Yuen, J. (2006). Deriving decision rules. The Plant Health Instructor. doi:10.1094/phi-A-2006-0517-01
- Zehnder, G., Gurr, G. M., Kühne, S., Wade, M. R., Wratten, S. D., & Wyss, E. (2007). Arthropod pest management in organic crops. Annual Review of Entomology, 52, 57–80. doi:10.1146/annurev.ento.52.110405.091337
- Zhang, M. Q., Rao, G. P., Gaur, R. K., Ruan, M. H., & Singh, M. (2008). Characterization, diagnosis and management of plant viruses. Industrial Crops, 1, 111–144.
- Zhao, M., Ho, H., Wu, Y., He, Y., & Li, M. (2014). Western flower thrips (Frankliniella occidentalis) transmits maize chlorotic mottle virus. Journal of Phytopathology, 162, 532–536. doi:10.1111/jph.2014.162.issue-7-8