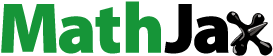
Abstract
Technical and economic performance of mechanized reaping and sickle harvesting methods were assessed under farmer’s field conditions in the Ashanti Region of Ghana for Amankwatia and AGRA rice varieties. Harvesting trials and field measurements were statistically analyzed as a split-plot layout in a randomized complete block design with three replicates at p < 0.05. Breakeven analysis for both harvesting options was conducted. Harvesting efficiency, field capacity, grain loss, and harvesting speed, respectively, ranged from 83.53% to 91.16%, 0.019 ha/h to 0.28 ha/h, 0.16% to 0.26%, and 0.19 km/h to 2.43 km/h for manual cutting with the sickle and mechanized reaping, irrespective of rice variety. Manual cutting was better at reducing grain loss irrespective of rice variety whereas Amankwatia offered significantly lower grain losses. The mechanized reaper was economically viable over manual cutting, breaking even after only 150 h of machine use and offering a substantially higher profit margin.
PUBLIC INTEREST STATEMENT
Information on technical and field performance of rice harvesting methods is critical to the development of locally adapted harvesting technologies and the improvement of the overall performance of existing ones. Information on the economic feasibility of rice harvesting systems helps to guide policy on rice harvesting mechanization. Data generated from this study will serve as an important reference material to students, researchers, and other relevant stakeholders in the field of science and technology. Field performance and economic feasibility of mechanized reaping and sickle harvesting methods were assessed under a farmer’s field conditions in this study. The mechanized reaper was economically viable over manual cutting, breaking even after only 150 h of machine use and offering a substantially higher profit margin. It is expected that results from this study will boost government and private sector interest in supporting smallholder farmers in the quest to increase national total annual rice production while improving on grain quality to international standards for enhanced marketability.
Competing Interests
The authors declares no competing interests.
1. Introduction
Rice (Oryza sativa L.) is one of the major crops cultivated worldwide and continues to be an important staple food crop in most developing economies (Elbehri et al., Citation2013). In sub-Saharan Africa (SSA), rice is the most rapidly growing food commodity and is now SSA’s second-largest source of food energy (Seck et al., Citation2013). Rice has become the second most important food staple after maize in Ghana and its consumption keeps increasing as a result of population growth, urbanization, and change in consumer habits (MoFA, Citation2009). The crop also constitutes 58% of all cereal imports (CARD, Citation2010) and accounts for nearly 15% of the country’s Gross Domestic Product (GDP) (ISSER, Citation2014).
Timely harvesting of rice is critical, as delayed harvesting can contribute to substantial quantitative and qualitative losses (Alizadeh & Bagheri, Citation2009). Rice is traditionally harvested using sickles and cutlasses with their associated high levels of drudgery and low timeliness of operation. Whereas one person can use a mechanized reaper to harvest a hectare of rice field in an hour, it would require about five persons to manually harvest the same field in an hour using sickles (Aung et al., Citation2014; Prakash et al., Citation2015). Due to labor shortages and its associated high cost, ripe paddy fields can be left unharvested for longer periods; a situation that leads to the loss of considerable amounts of food grains annually (Nipa, Citation2016), greatly affecting national food security.
Agricultural production in Ghana and rice cultivation for that matter is generally characterized as predominantly practiced on smallholder, family-operated levels (Amponsah et al., Citation2012). The use of improved rice mechanization options like combine harvesters, though better, are usually not affordable to these resource poor farmers due to the huge initial investment and skilled labor requirement. Even in cases where they are accessible, it is highly uneconomical to patronize them for use on such woefully small farm holdings. The use of rudimentary harvesting tools is a serious disincentive to these smallholder farmers, making it difficult for increased production of the crop. Aung et al. (Citation2014) mentioned that in the case of rice harvesting for developing economies, the use of mechanical reapers is appropriate for smallholdings.
The reaper is a harvesting machine used for cutting paddy crop mechanically while ensuring that cut crop bundles are well arranged aside to facilitate manual collection after harvest. Reapers come in different designs and sizes and offer farmers the luxury of standing while harvesting, unlike traditional harvesting options, as a way of reducing drudgery. For the various heights of a person, the height of the handle can be adjusted accordingly. Fuel consumption and noise levels are usually lower compared to combines. Reapers are also well suited for mountainous and hilly regions where the use of combine harvesters is practically impossible.
Most African countries including Ghana have in the recent past made serious attempts to introduce few rice harvesting technologies from Asia to help boost the rice sector (Rickman et al., Citation2013). Between 2007 and 2010 alone, the Ghana government through the Agricultural Engineering Services Directorate, MoFA imported 30 rice reapers, 30 rice threshers and 39 rice combines to be supplied to smallholder farmers across the country (MoFA SRID., Citation2011). Since these efforts from the government are commendable, they are not enough. Amponsah et al. (Citation2012) indicated that procuring tractors and farm implements alone is not the end but a means to an end to achieving an effective national mechanization scheme. Sadly, most imported machinery are not well suited to prevailing local field conditions. It is therefore not uncommon to see lots of abandoned machinery on rice fields after a short period of use (Addo & Amponsah, Citation2018).
Currently, there is a dearth of information on the technical performance of mechanized rice reapers in Ghana. Such information will be useful to engineers and other relevant mechanization experts when it comes to possible design modifications and technology adaption. Most importantly, it will offer smallholder rice farmers first-hand experience and help answer their doubts on both technical performance and economic feasibility associated with these rice harvesting implements. Farmer acceptance will facilitate future adoption of rice harvesting technologies and consequently lead to increased production of rice nationwide.
1.1. Study objectives
The main aim of this study was to technically and economically assess the performance of mechanized reaping and sickle cutting options for rice harvesting under farmer’s field conditions.
Specific objectives of this study were to:
assess the field performance of a mechanized reaper at different forward speeds in terms of field capacity, field efficiency, fuel consumption, slippage, grain loss, and level of drudgery.
comparatively, evaluate the performance of mechanized reaping and sickle cutting options as influenced by rice variety.
determine the energy expenditure and respective rest periods for mechanized reaping and manual cutting options.
to assess the economic feasibility of both rice cutting options from the smallholder farmer’s perspective.
2. Materials and methods
2.1. Study location and rice variety
The study was conducted at Nobewam in the Ejisu-Juaben municipality located in the Ashanti Region of Ghana under farmer’s field conditions. The field was planted to both Amankwatia and AGRA rice varieties using the seedling transplanting method.
2.2. Machine specification
Figure shows a labeled pictorial view of the Yanmar YAP 120 mechanized reaper (Yanmar Co., Ltd., Osaka, Japan). The following technical specifications of the machine were determined prior to field evaluation; overall dimensions and weight, power source, number of gears, details of cutting mechanism, steering mechanism, and safety arrangements.
Table presents the technical details and specifications of the Yanmar YAP 120 mechanized paddy reaper.
Table 1. Technical specifications of the mechanized paddy reaper
2.3. Manual paddy harvesting
Paddy was manually harvested using a locally made sickle (Figure )). Sizable bundles of rice panicles were held in one hand and the other hand with the sickle was used to cut the crop at a height of 5–10 cm above ground level (Figure )). The cut bundles were gently placed aside after cutting to make way for the farmer to harvest others ahead.
2.4. Crop and soil condition
The test condition of the crop (variety, duration of crop, grain/straw ratio, grain/straw moisture content, grain size, percentage of damaged grain) was determined using appropriate procedures proposed by Smith et al. (Citation1994). Crop spacing (row and hill), crop height, and crop density (plants/m2) were also determined using appropriate procedures.
Before and after harvesting each field, an Eijkelkamp model 0615SA soil penetrologger (Eijkelkamp Soil and Water, Giesbeek, The Netherlands) with a 60° circular steel cone and a base area of 100 mm2 was used to determine the soil strength whereas soil core samplers were used to fetch soil samples for moisture content and bulk density determination at depths of 0–10, 10–20, 20–30, and 30–40 cm as adopted by Amponsah et al. (Citation2014).
2.5. Crop moisture content
From each field to be harvested, 3 paddy crop samples of approximately 500 g each were randomly fetched. The samples were placed in sealed plastic containers and taken to the postharvest laboratory of the Agricultural and Biosystems Engineering department, KNUST, Kumasi where the grains and straw were separated by hand. The straw and grains from each sample were kept paired. After weighing with a sensitive electronic scale, the samples were oven-dried at 130°C for at least 15 h and then reweighed. The moisture content (% w.b.) is calculated using EquationEquation 1(1)
(1) :
2.6. Grain/straw ratio
After determining the weight of the dry samples, the result of the paired samples was used to calculate the mean grain/straw ratio using EquationEquation 2(2)
(2) :
2.7. Size of grains
From a representative sample of the test material, grain and straw were separated by hand and the size of 50 grains measured. From these measurements, the average diameter and length was determined. Grains were also inspected for damage and the damage was calculated as a percentage of the total number of grains sampled.
2.8. Reaper field test procedure
With the reaper set up in accordance with the manufacturer’s instructions, runs were made at two forward gear settings (Gear 1 and Gear 2). For each experimental run, bundles of paddy were cut at uniform rates as the reaper was set in forwarding motion and the time requirement for each gear setting was recorded. Any time for stoppages was recorded with the total testing time. Observations on factors affecting the operation of the machine were also recorded together with any adjustments and repairs.
Tests were carried out to determine the following parameters during harvesting with the mechanized reaper: grain loss, field efficiency, field capacity, slippage, harvesting speed, fuel consumption, and operator’s physical energy requirement.
2.9. Grain loss assessment
Grains were lost due to shedding on the ground by the passage of the reaper cutter bar and dividers or manual throwing of sickle during paddy harvesting. After harvesting each row, a wire rectangle (1 m × 0.3 m) was used to mark a piece of ground, and grains within this area were collected by hand. At each sampling point, the wire rectangle was located with its short side against the uncut crop and all grains and heads which lie in the stubble within this area were placed in an envelope after weighing on a sensitive electronic scale. Harvesting grain loss was then calculated as a percentage of total grain yield within the area harvested.
2.10. Field capacity and efficiency
After the machine had been satisfactorily set, each test plot was completed without stopping unless this was necessary due to field adjustments or breakdowns. The time lost in the field due to turning and other factors including failure to use the full width of the implement affects field efficiency. Field capacity and efficiency for both mechanized and manual cutting options were calculated using EquationEquations 3(3)
(3) ,Equation4
(4)
(4) and Equation5
(5)
(5) as defined by ASABE standard S495.1 (ASABE, Citation2005):
2.11. Slippage and speed measurement
Wheel slippage was determined by measuring the reaper’s speed in the transport position on a concrete surface and also under the actual field conditions and computed using EquationEquation 6(6)
(6) adopted by Naderi et al. (Citation2008) and Jenane et al. (Citation1996).
Using ranging poles and a stopwatch, the speed of travel for different field conditions was determined from the time taken to travel a 20 m distance with the harvester in the transport position. This was done on the stubble after paddy harvesting and the process was repeated on the concrete surface. The same distance of 20 m was used in calculating the speed of harvest for manual harvesting with the sickle; where 3 persons were tasked to harvest a meter width of a crop field and time taken to complete recorded for each person.
2.12. Fuel consumption
Fuel consumption was measured by filling the reaper’s engine fuel tank completely at the start and finish of each harvesting period and recording the quantity of fuel added (ASABE, Citation2011; S K Amponsah et al., Citation2014; Smith et al., Citation1994). A graduate cylinder was used for refilling.
2.13. Harvesting drudgery
A heart rate sensing device (Polar RS 800) was used to obtain the heart rate of the operator during experimental trials with the mechanized paddy reaper and manual harvesting with the sickle adopting the procedure by Amponsah et al. (Citation2014). Before and after each physical activity, the person is allowed 10 (10) min period of rest so the heart rate could be stabilized which are referred to as the rest and recovery periods, respectively. Using the mean heart rate obtained for a specific physical activity to trace for a corresponding energy consumption value on the heart rate-energy conversion chart (Jones, Citation1988), the Gross energy consumed (Watts) was determined. Information on energy expenditure for a particular physical work is useful in determining the rest period required by a person after work using EquationEquation 7(7)
(7) as suggested by Jones (Citation1988).
where
Tr = Total rest period (min/hr)
P = Gross energy consumption (Watts)
2.14. Economic feasibility assessment
The cost of harvesting (both mechanized and manual) was calculated by considering the fixed and variable costs. Fixed (ownership) costs include depreciation, interest, taxes, insurance, and shelter. Operating costs, on the other hand including repairs and maintenance, fuel, lubrication, and operator charge. The total cost of the machine is the sum of its total-fixed costs and total variable costs. Depreciation on mechanized reaper was calculated using the straight-line method according to Hunt (Citation1983) using EquationEquation 8(8)
(8) while the interest on machine ownership was calculated using EquationEquation 9
(9)
(9) .
Taxes, insurance, and shelter are usually 1.0% of the purchase price. Where 0.5% each of purchase price is allocated to insurance and shelter and 0% of the purchase price for taxes (Hunt, Citation1983). Fuel cost depends on the vehicle’s fuel consumption (L/ha), cost of fuel (US$/L), field capacity (ha/h), and working hours per year. Lubricant cost is usually calculated as 15% of the fuel cost unless lubricant consumption (L/ha) is otherwise stated. Repairs and Maintenance (R&M) cost is usually 5% of the machinery purchase cost while labor cost depends on the number of farmhands required to complete a specific harvesting task and the rate charged per hectare (Hanna, Citation2001).
Based on calculated total harvesting cost and assumed per hour hiring cost, the expected revenue, profit, and break-even cost were determined for each harvesting method as recommended by Fairhurst (Citation2012).
2.15. Experimental design
The results of paddy harvesting trials and field measurements were statistically analyzed as a split-plot layout in a randomized complete block design (RCBD) with three replicates, using MINITAB Statistical Software Release 15 (MINITAB Inc, Citation2007). The main plot treatment was rice variety and harvesting method the subplot treatment. The least significant difference (LSD) was used at a 5% significance level to identify the difference between treatment means. Analysis of variance (ANOVA) was performed to determine the effects of harvesting method and rice variety and their interaction on harvesting efficiency and grain loss.
3. Results and discussion
3.1. Soil physical properties
Figure depicts the soil moisture content before and after paddy harvesting with the mechanized reaper. Soil moisture content before harvesting increased from 23.36% d.b. to 29.59% d.b. with increasing soil depth (10 cm to 40 cm) while soil moisture after harvesting ranged from 22.02% to 29.11% d.b.
The graph indicates that soil moisture content before reaper harvesting was generally greater than after harvesting, though differences were not significant (p < 0.05).
The mean bulk density and soil penetration resistance before and after reaper harvesting of paddy are presented in Figure . Mean soil bulk density before harvesting ranged from 1.19 to 1.31 g/cm3 while after harvesting, it ranged from 1.21 to 1.33 g/cm3 and generally increased as soil depth increased from 10 cm to 40 cm.
Figure 4. Mean bulk density (BD) and soil penetration resistance (SS) before and after reaper harvesting
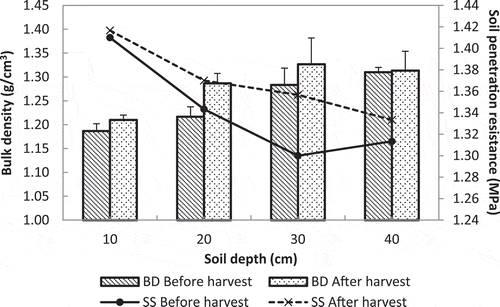
Mean penetration resistance ranged from 1.30 MPa to 1.41 MPa while after harvesting, it ranged from 1.33 to 1.42 MPa and generally increased as soil depth increased from 10 cm to 40 cm. The mechanized reaper may have caused some compaction of the soil during harvesting, though differences in soil bulk density and soil penetration resistance before and after harvesting were not significant at p < 0.05 regardless of soil depth.
3.2. Crop condition
Details of crop conditions for Amankwatia and AGRA rice varieties before mechanized and manual harvesting are shown in Table .
Table 2. Crop condition at harvest
Except for percentage grain damage, Amankwatia variety recorded greater values for all other parameters (grain moisture, straw moisture, grain–straw ratio, grain diameter, grain length, crop height, and crop diameter) than AGRA rice variety.
3.3. Performance evaluation
Figure shows the mechanized reaper harvesting speed and wheel slippage at the first and second forward gear settings.
Reaper harvesting speed ranged from 1.49 to 2.43 km/h for the first and second gear, respectively. Also, the mean wheel slippage ranged from 2.26% to 3.44% for the second and first gear settings, respectively.
Reaper harvesting speed at the second gear was significantly (p < 0.05) greater than at the first gear setting, irrespective of the rice variety harvested. Conversely, wheel slippage at the second gear setting was significantly lower than at the first gear setting. Results also indicate that wheel slip decreased with increasing reaper harvesting speed. This could be attributed to the fact that at relatively faster travel speed, the wheels move faster making it possible to reduce losses in travel distance than at lower speeds.
The mean fuel consumption and field capacity at the first and second gear settings during harvesting with the mechanized reaper is shown in Table . Fuel consumption and field capacity ranged from 6.57 L/ha to 7.45 L/ha and 0.28 ha/h to 0.17 ha/h for secondand firstgear settings, respectively. Fuel consumption was greater at the first gear than the second gear setting and showed a significant difference.
Table 3. Fuel consumption and field capacity at first and second gear settings for mechanized reaper
Similarly, field capacity was significantly (p < 0.05) greater at the first gear than the second gear setting. This could be due to the fact that at lower gear speed, the machine’s total working time is increased which means, more fuel is required to harvest the same field than at a higher gear speed setting. Hignight and Watkins (Citation2007) cited that the amount of fuel consumed usually decreases with increased efficiency.
Table shows the field efficiency, grain loss, and physical energy expenditure during harvesting with the mechanized reaper at the first and second gear settings. For the first and second gear settings, the mean-field efficiency, grain loss, and physical energy requirement, respectively, ranged from 88.68% to 91.16%, %,0.17% to 0.26%, and 546 to 615 W. The mean-field efficiency and physical energy expenditure during mechanized reaping at the second gear was greater than at the first gear setting though no significant difference was observed at the 5% level. This trend could be attributed to the fact that at higher gear speed, harvesting can be completed in the shortest possible time; however, more effort is also required to control the machine resulting in the greater energy requirement.
Table 4. Mean-field capacity, grain loss, and physical energy expenditure at different gear settings during mechanized reaping
Likewise, the mean grain loss during harvesting with the mechanized reaper at the first gear was lower than at the second gear setting but the difference was significant (p < 0.05). This is because at higher gear settings, the impact and vibration of the reaper cutting unit on the crop is faster and greater resulting in the shattering of more grains and relatively greater losses. This agrees with justification by Nipa (Citation2016) on the possible causes of mechanized reaper shattering losses.
The mean percentage grain loss during mechanized reaping at various gear settings and manual cutting of paddy with a sickle for Amankwatia and AGRA rice varieties is shown in Figure . Harvesting with the mechanized reaper at the second gear setting for AGRA rice variety recorded the greatest grain loss of 0.36% which was significantly greater than the least (0.20%) recorded during manual harvesting. Similarly, harvesting with the mechanized reaper at second gear setting for Amankwatia rice variety recorded the greatest grain loss of 0.16% which was significantly greater than the least (0.11%) recorded with the first gear setting.
Figure 6. Mean percentage grain loss using mechanized and manual paddy cutting options for Amankwatia (AMK) and AGRA rice varieties
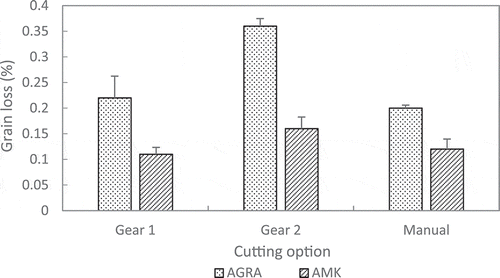
AGRA rice recorded significantly (p < 0.05) greater grain losses than Amankwatia variety across all paddy cutting options. This was because aside from the fact that Amankwatia naturally has a lower shattering ability, its grain moisture content at harvest was greater than AGRA rice variety (Table ). This agrees with studies by FAO (Citation1976) on factors affecting rice harvesting.
Figure depicts the mean-field capacity associated with mechanized reaping at first and second gear settings and manual harvesting with the sickle for Amankwatia and AGRA rice varieties. Mechanized reaping of AGRA rice using the second gear setting recorded the highest field capacity of 0.322 ha/h which was significantly greater than the least (0.022 ha/h) under manual cutting with the sickle. Similarly, mechanized harvesting of Amankwatia with the reaper at second gear setting gave the greatest field capacity of 0.254 ha/h which was significantly greater than the least (0.016 ha/h) recorded for manual cutting with the sickle.
Figure 7. Mean-field capacity using mechanized and manual paddy cutting options for Amankwatia (AMK) and AGRA rice varieties
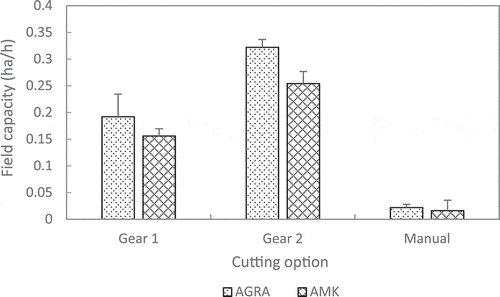
AGRA rice generally gave significantly lower field capacities than Amankwatia variety across all paddy cutting options. This was probably because, at harvest, crop density and moisture content for AGRA rice was lower than Amankwatia (Table ), facilitating faster and easier cutting. Nipa (Citation2016) also cited plant hill density as having an effect on the speed of mechanized reaping of paddy.
The mean-field capacity, field efficiency, grain loss, and harvesting speed associated with mechanized harvesting with reaper at first and second gear settings and manual harvesting with the sickle is presented in Table .
Table 5. Mean-field capacity, field efficiency, grain loss, and harvesting speed for mechanized and manual cutting options
Reaper harvesting at the second gear gave the greatest field capacity of 0.28 ha/h which was significantly greater than the least (0.019 ha/h) recorded for manual cutting with the sickle. Conversely, for field efficiency, reaper harvesting at the second gear setting recorded the greatest (91.16%) which was significantly greater than the least (83.53%) recorded during manual cutting with the sickle. Mean grain loss ranged from 0.16% to 0.26% for manual harvesting with a sickle and mechanized reaping at the secondgear setting respectively. Harvesting speed also ranged from 0.19 km/h to 2.43 km/h for manual harvesting with the sickle and reaper harvesting at the second gear setting, respectively.
Manual harvesting with the sickle recorded the least significant grain loss compared to mechanized harvesting with the reaper, irrespective of rice variety. This could be due to the fact that with manual cutting using the sickle, the harvesting speed is significantly (p < 0.05) slower hence there is minimal impact on crop stands and reduced grain shattering compared to mechanized reaping. Moreover, the manual harvester is more careful during harvesting so as to reduce shattering losses.
Table shows the mean heart rate with corresponding energy expenditure and the rest period for mechanized reaping at first and second gear settings and during manual harvesting with the sickle. Mean heart rate ranged from 102 bpm to 119 bpm with manual harvesting using the sickle requiring the greatest energy expenditure of 740 W which was significantly greater than the least (546 W) required when using the mechanized reaper at first gear setting.
Table 6. Mean heart rate with corresponding energy expenditure and rest period for mechanized and manual cutting options
[*] Within each column, means followed by the same letter are not significantly different at α = 0.05
Also, the rest period ranged from 32.53 min/h to 39.73 min/h for mechanized reaping at first gear setting and manual harvesting with the sickle, respectively.
3.4. Economic analysis
Table presents the total cost of mechanized reaping using the Yanmar YAP 120 paddy reaper and manual harvesting with the sickle based on relevant assumptions. At an investment cost of US$ 1500.00, the mechanized reaper offered a total annual cost of US$ 3121.00 while the manual harvesting with the sickle option, at an investment cost of US$ 16.00, yielded a total cost of US$ 1504.00 per annum.
Table 7. Total cost for mechanized and manual paddy harvesting options
The break-even chart for mechanized and manual harvesting options using the Yanmar YAP 120 paddy reaper and the sickle, respectively, is presented in Figure . Break-even calculation was based on the assumption that the cost of harvesting was US$ 5.00 and US$ 0.20 per hour for mechanized and manual options, respectively, for a maximum of 1000 h of work per annum.
Mechanized harvesting using the reaper offered greater total annual cost and revenue than the manual cutting with the sickle option. At 1000 h of annual machine use, the mechanized Yanmar YAP 120 paddy reaper is yielding a total revenue of US$ 5000.00 as compared to US$ 200.00 for manual harvesting using the sickle. The break-even point for mechanized harvesting using the reaper was at 150 h of machine use, equivalent to 43 hectares of harvested area. For manual harvesting with the sickle, on the other hand, the total cost far exceeded the revenue, and therefore even after 1000 h of machine use, realizing any profit was impossible. This is in agreement with studies by Nipa (Citation2016) citing mechanized reaping as economically beneficial than manual harvesting options.
Table shows the cost of mechanized harvesting using the Yanmar YAP 120 reaper and manual harvesting with the sickle expressed as a percentage of total per hectare cost of paddy rice production using the Nobewam rice fields as a case study as of November 2016.
Table 8. Cost of mechanized and manual paddy harvesting options as a percentage of total per hectare production cost
The total cost of paddy rice production per hectare of land cultivated, excluding harvesting cost, was at US$ 1025.00. The cost of manual harvesting per hectare using the sickle was at US$ 125.00 representing 11% of the total production cost of paddy. Conversely, the cost of mechanized harvesting using the reaper per hectare was at US$ 112.50 representing 10% of the total paddy production cost and a 10% reduction in total harvesting cost over manual cutting with the sickle.
4. Conclusions
Paddy harvesting (manual and mechanized) was possible on soils with moisture content, bulk density, and penetration resistance ranging from 23.36% d.b. to 29.59% d.b, 1.19 g/cm3 to 1.31 g/cm3, and 1.30 MPa to 1.41 MPa, respectively. There was however no change in soil physical properties after harvesting. Harvesting with the mechanized reaper at the second gear setting was better at reducing wheel slippage, fuel-saving and offered better timeliness of operation than at the first gear. Harvesting at the first gear setting was however better at reducing grain loss and physical energy expenditure but associated with lower harvesting efficiency. Manual cutting was better at reducing grain losses than mechanized reaping irrespective of rice variety. However, Amankwatia offered significantly lower grain loss compared to the AGRA rice variety, regardless of the cutting option used.
Harvesting efficiency, field capacity, grain loss, and harvesting speed, respectively, ranged from 83.53% to 91.16%, 0.019 ha/h to 0.28 ha/h, 0.16% to 0.26%, and 0.19 km/h to 2.43 km/h for manual cutting with a sickle and mechanized reaping, irrespective of rice variety. Energy expenditure ranged from 546 W to 740 W with a corresponding rest period ranging from 32.53 min/h to 39.73 min/h for mechanized reaping at the first gear setting and manual harvesting with the sickle, respectively. Mechanized harvesting using the Yanmar YAP 120 paddy reaper was economically viable to the smallholder rice farmer than manual cutting using the sickle with break-even occurring after only 150 h of machine use (43 ha harvested area) and a substantially huge profit margin. The cost of harvesting with the mechanized reaper was at US$ 112.50 per hectare representing 10% of the total paddy production cost and a 10% reduction in total harvesting cost over the manual cutting option using the sickle.
This study should be repeated for other existing mechanized reapers in different agro-ecologies under varying field conditions. Both the government and the private sector should harness the opportunity of making huge returns through the promotion and supply of mechanized reapers to smallholder rice farmers across the country to help reduce harvesting drudgery while increasing rice production. Rice breeding programs should focus on releasing varieties with characteristics like Amankwatia to help reduce grain-shattering losses during harvesting.
Acknowledgements
The authors wish to acknowledge the financial support from the Africa Rice Centre, through the Support to Agricultural Research for Development of Strategic Crops in Africa (SARD-SC) project. We wish to also express our gratitude to the field staff of the CSIR-Crops Research Institute, Kumasi, Ghana, and the rice farmers at Nobewam, Ashanti Region, Ghana who played a part in making the study a success.
Additional information
Funding
Notes on contributors
Shadrack Kwadwo Amponsah
Shadrack Kwadwo Amponsah (PhD), corresponding and lead author for this study, is an Agricultural Engineer by profession and training with specialisation in Agricultural Mechanisation. Shadrack is a Research scientist at the CSIR-Crops Research Institute, Kumasi and a Lecturer (part-time) at the Agricultural and Biosystems Engineering Department, KNUST, Kumasi. His research and academic activities are focused on developing demand-driven agricultural technologies. He has 12 years’ experience in research with over 20 peer reviewed articles to his credit. Shadrack won the Engineering excellence “Most Published Author” award and RUFORUM Young Scientist award in 2017 and 2019, respectively. He successfully led a team of researchers in 2017 to assess the performance of the Chinese Sifang mini rice combine under farmers conditions in Benin. This study was a branch of his previous studies on rice harvesting technologies. His current research activities include the development of multi-crop threshers, multi-crop seeders and improved sun drying platforms.
References
- Addo, A., & Amponsah, S. K. (2018). Present status and future prospects of agricultural machinery industry in Ghana. AMA, Agricultural Mechanization in Asia, Africa and Latin America, 49(2), 87-94.
- Alizadeh, M. R., & Bagheri, I. (2009). Field performance evaluation of different rice threshing methods. International Journal of Natural & Engineering Sciences, 3(3), 139–18. http://www.ijnes.org/index.php/ijnes/article/view/484
- Amponsah, S. K., Oteng-Darko, P., & Kumi, F. (2012). Potential and constraints of agricultural mechanisation in Ghana—A review. International Agricultural Engineering Journal, 21(2), 38–45. http://www.iaej.cn/EN/abstract/abstract135.shtml
- Amponsah, S. K., Sheriff, J. T., & Byju, G. (2014). Comparative evaluation of manual cassava harvesting techniques in Kerala, India. Agricultural Engineering International: CIGR Journal, 16(2), 41–52. https://cigrjournal.org/index.php/Ejounral/article/view/2793/1892
- Amponsah, S. K., Bobobee, E. Y. H., Agyare, W. A., Okyere, J. B., Aveyire, J., & King, S. R. (2014). Mechanical cassava harvesting as influenced by seedbed preparation and cassava variety. Applied Engineering in Agriculture, 30(3), 391–403. https://doi.org/10.13031/aea.30.10495
- ASABE. (2005). Uniform terminology for agricultural machinery management (S495.1). ASABE standards. American Society of Agricultural and Biological Engineers, St. Joseph, MI.
- ASABE. (2011) . Agricultural machinery management data (D497.7). ASABE standards. American Society of Agricultural and Biological Engineers, St. Joseph, MI.
- Aung, N. N., Myo, W. P. P., & Htet, Z. M. (2014). Field performance evaluation of a power reaper for rice harvesting. International Journal of Scientific Engineering and Technology Research, 3(12), 2631–2636. https://studylib.net/doc/8335478/field-performance-evaluation-of-a-power-reaper-for-rice-h.
- CARD. (2010). Mapping of poverty reduction strategy papers (PRSPs), sector strategies and policies related to rice development in Ghana. Accra, Ghana: University of Ghana.
- Elbehri, A., Kaminski, J., Koroma, S., Iafrate, M., & Benali, M. (2013). West Africa food systems: An overview of trends and indicators of demand, supply, and competitiveness of staple food value chains. In A. Elbehri (Ed.), Rebuilding West Africa’s Food Potential (pp. 1–44). FAO/IFAD.
- Fairhurst, D. S. (2012). Using Excel for business analysis: A guide to financial modelling fundamentals. Wiley Publishing.
- FAO. (1976). Mechanization of rice production: India-Nigeria-Senegal, an international coordinated research project project: 1970-1976. Food and Agriculture Organization of the United Nations.
- Hanna, M. (2001). Estimating field capacity of field machines. Cooperative Extension Service, Iowa State University of Science and Technology, PM, 696,1–4. https://blogs.cornell.edu/capitalareaagandhortprogram/files/2016/03/Estimating-Field-Capacity-of-Farm-Machines-1yiwytr.pdf
- Hignight, J. A., & Watkins, K. B. (2007). The value of rice harvesting efficiency. AAES Research Series 560, 283–287. https://scholarworks.uark.edu/cgi/viewcontent.cgi?article=1087&context=aaesser
- Hunt, D. R. (1983). Farm power and machinery management. Ames, IA (8th ed.). Iowa State University Press.
- ISSER. (2014) . The state of the Ghanaian economy.
- Jenane, C., Bashford, L. L., & Monroe, G. (1996). Reduction of fuel consumption through improved tractive performance. Journal of Agricultural Engineering Research, 64(2), 131–138. https://doi.org/10.1006/jaer.1996.0054
- Jones, N. L. (1988). Clinical Exercise Testing (3rd ed.). W.B. Saunders.
- MINITAB Inc. (2007). MINITAB statistical software release 15 for windows.
- MoFA. (2009). Ministry of food and agriculture the republic of ghana national rice development strategy (NRDS) draft report. Accra: Ministry of Food and Agriculture.
- MoFA SRID.. (2011). Agriculture in Ghana - Facts and figures (2010). Accra: Ministry of Food and Agriculture.
- Naderi, M., Alimardani, R., Abbaszadeh, R., & Ahmadi, H. (2008). Assessment of dynamic load equations through drive wheel slip measurement. American Eurasian Journal of Agricultural & Environmental Sciences, 3(5), 778–784. http://www.idosi.org/aejaes/jaes3(5)/18.pdf
- Nipa, J. F. (2016, March 8-10). Study on performance evaluation of self-propelled reaper. In Proceedings of the 2016 International Conference on Industrial Engineering and Operations Management, (pp. 1527–1536).
- Prakash, R. J., Kumar, B. A., Reddy, G. A., & Reddy, K. V. S. R. (2015). Performance evaluation of reaper-binder in rice crop. International Journal of Agricultural Engineering, 8(2), 232–238. https://doi.org/10.15740/HAS/IJAE/8.2/232-238
- Rickman, J., Moreira, J., Gummert, M., & Wopereis, M. C. S. (2013). Mechanizing Africa’s rice sector. In M. C. S. Wopereis, D. E. Johnson, N. Ahamdi, E. Tollens, & A. Jalloh (Eds.), Realizing Africa’s rice promise (Vol. 1, pp. 332–342). CAB International.
- Seck, P. A., Touré, A. A., Coulibaly, J. Y., Diagne, A., & Wopereis, M. C. S. (2013). Africa’s rice economy before and after the 2008 rice crisis. In M. C. S. Wopereis, D. E. Johnson, N. Ahamdi, E. Tollens, & A. Jalloh (Eds.), Realizing Africa’s rice promise (pp. 24–34). CAB International.
- Smith, D. W., Sims, B. G., & O’Neill, D. H. (1994). Testing and evaluation of agricultural machinery and equipment: Principles and practices. Food and Agriculture Organization.