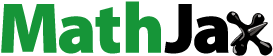
Abstract
The potato is an important tuberous vegetable crop. However, its production and values are constrained by various factors, including the two-spotted spider mite (TSSM) in East Hararghe, Ethiopia. An investigation of the TSSM management by the application of botanicals and synthetic insecticides was conducted in the laboratory and greenhouse. The experiment was arranged in CRD. The treatment was replicated four times in the greenhouse and five times in the laboratory. The efficacy and LC50 bioassays of Amitraz, Profenofos, and Emamectin benzoate, as well as the oils of Azadirachta indica seed and Tagetes minuta leaf, and their leaf aqueous extracts, were tested in the laboratory at three dose levels. Similarly, Amitraz, Profenofos, Emamectin benzoate (EB) and extracted oils of Azadirachta indica seed alone and in combination were tested in a greenhouse The data was collected after 24, 48, and 72 hours of their application in the laboratory. And in the greenhouse, within 7, 14, and 21 days before and after application. In the laboratory, the highest mortality of TSSM adults was recorded with EB at 80 µL (74–97%), followed by profenofos at 35 µL (71–90%). Among the botanicals, the A. indica seed oil at 5% caused the highest mortality of TSSM adults (72–100%). Amitraz also caused the highest mortality of TSSM eggs (72.5–92.5%), and it required in 100ml of tap water to kill 50% of the eggs. T. minuta leaf oil had higher toxic effects on TSSM eggs (0.239%). In the greenhouse, the dose combination of Amitraz, EB, and profenofos caused higher mortality of TSSM than their sole application.
1. Introduction
Potato (Solanum tuberosum L.) is the world’s fourth most important vegetable crop, trailing only maize (Zea mays L.), paddy (Oryza sativa L.), and wheat (Triticum aestivum L.) (Demissie, Citation2019; FAOSTAT, Citation2021; Kafle et al., Citation2019; Liu et al., Citation2021; Shirin et al., Citation2007). It was also ranked third in terms of consumption, trailing only wheat and rice (FAOSTAT, Citation2021). In 2019, global potato tuber production was estimated to be over 370 million metric tons. However, its production and consumption rates vary across the globe. Furthermore, it is produced to help the national economy by generating income or as a source of revenue, as well as to improve food security and food value (Gildemacher et al., Citation2009; Tiruneh et al., Citation2017).
Ethiopia is also ranked ninth in Africa for potato production. At the national level, potato tubers were the most produced and consumed vegetable, followed by cassava, sweet potato, and yam (FAOSTAT, Citation2021; Wondimu Belay et al., Citation2021). Nationally, the average potato yield is estimated to be 13.9 tons/ha, covering approximately 69,610.81 hectares of land (CSA , Citation2010). Its output and productivity vary across Ethiopian regions. In comparison, the Eastern Hararghe zone has the most potential for potato tuber production. The average yield of potato tubers in this zone is estimated to be more than 19.3 tons/ha (CSA , Citation2014), which is greater than the national average yield. However, low-yielding varieties, pests (Ferdu et al., Citation2009; Bezabih & Mengistu, Citation2011), unfavorable climatic conditions, and soil nutrient depletion limit its production and productivity. Among the potato arthropod pests, the two-spotted spider mite (TSSM), Tetranychus urticae, has been causing potato tuber yield losses in the eastern Hararghe zone since 2015 (Belay et al., Citation2018; Goftishu et al., Citation2016) as a result of the drought conditions.
Farmers relied heavily on synthetic chemicals to manage TSSMs for many years, particularly in horticultural crops. However, if it is used frequently and extensively, the TSSM develops resistance to its efficacy (Grbic et al., Citation2011). This occurred as a result of their high reproductive potential, short generation time, haplodiploid sex system, and low gene exchange with susceptible species (Martin et al., Citation2015; Stavrinides et al., Citation2010). As a result, the farmers were unable to control it solely with conventional insecticides.
T. urticae’s susceptibility and resistance to acaricides and insecticides is determined by whether fecundity has increased or decreased. According to James and Price (Citation2003), imidacloprid was ineffective on TSSM because its fecundity increased by 20% after exposure. A pesticide is also costly to purchase and results in the extinction of natural enemies (Stavrinides & Mills, Citation2009). Nonetheless, it could be used as part of an integrated pest management strategy. Tesfay et al. reported the most effective synthetic insecticides against TSSM in potato plants in eastern Ethiopia (2018). However, there was no mention of botanical insecticides or the recommended doses per hectare. Botanical insecticides, on the other hand, could be used as an alternative to synthetic pesticides (Isman, Citation2006). Relying solely on synthetic pesticides causes pesticide resistance, destroys non-target or beneficial organisms, pollutes the environment, and has a negative impact on the health of mammals. As a result, the development of management options such as the use of botanical extracts against TSSM as an alternative to synthetic pesticides is used to extend the shelf life of pesticides, reduce resistance development, and reduce the environmental pollution caused by synthetic insecticides or acaricides. As a result, this study was initiated with the goal of evaluating the efficacy of selected botanicals and synthetic pesticides against two-spotted spider mites in the laboratory and greenhouse.
2. Materials and methods
2.1. Description of the study area
From October 2018 to March 2019, researchers at Haramaya University’s College of Agriculture and Environmental Sciences, School of Plant Sciences, evaluated the efficacy of plant extracts and selected synthetic insecticides against potato TSSMs in the plant protection laboratory and greenhouse.
2.2. Experimental materials, experimental design, and treatments
The Bubu variety of potato was used for the experiment. This variety was developed by the Root and Tuber Crops Improvement Program of Haramaya University, Ethiopia, and approved by the National Variety Release Committee in 2011 (Tsegaw, Citation2002). It was planted in pots (dimensions: 18 cm long, 20 cm, and 14 cm in diameter at the top and bottom, respectively). The experiment was laid out in a completely randomized design (CRD) with four replications in the greenhouse and five in the laboratory. In the greenhouse, the experiment was arranged by 1 m x 1 m on the bench to minimize the possible movement of T. urticae among the potato plants. The experimental treatments were assigned randomly to the experimental units, i.e., Petri dishes in the laboratory and pots in the greenhouse. The applied experimental treatment concentrations or doses are indicated in Table .
Table 1. Doses of the applied synthetic and botanicals pesticides in laboratory
2.3. Source of spider mite colony
A spider mite colony was collected from black nightshade (Solanum indicum L.) to obtain the desired colony. It was raised and fed on haricot bean plants in the greenhouse (Phaseolus vulgaris L.).
2.4. Formulation of synthetic insecticides
Dire Dawa farmer’s cooperative union sold three synthetic insecticides: Amitraz 20 EC, Profenofos 720 EC, and Emamectin benzoate 1.92 EC. Micro-pipettes were used to measure the dose required for laboratory and greenhouse bioassays (10–200 µL). Following measurement, each dose was thoroughly mixed with tap water according to the manufacturer’s recommendations.
2.5. Collection and formulation of botanicals
The neem (A. indica) seed and leaves were collected from the Dire Dawa Tony Farm. The stinking roger (Tagetes minuta) leaves were collected from the Haramaya district. After being collected, they were brought to Haramaya University’s Plant Protection Laboratory for drying under shade for three weeks. Then, the dried plant materials were ground by using a high-speed smashing machine. Finally, the powder was sieved by a 0.25 mm pore size and stored at room temperature by packaging and sealing in a plastic bag until it was utilized.
2.6. Extraction of botanical oils
At Haramaya University’s animal nutrition laboratory, the oil of A. indica (seed & leaves powder) and T. minuta leaves was extracted from a 30-gram powder using the Soxhlet extraction apparatus. This powder was initially mixed into clean thimbles. It was then covered with cotton. Finally, it was placed in an extractor by attaching it to the oil extraction apparatus’s condenser. The glass jars were also attached to the extractor after 250 ml of petroleum ether at 40–60 °C was added to moisten and mix the powder during oil extraction. It took 8 hours to complete one cycle of oil extraction. After 8 hours of oil extraction, the soxhlet heater was removed from the apparatus, as was the thimble that held the residues and flasks. The extracted botanical oil was then poured into the flasks that had been prepared. The thimble’s remaining powder residues were discarded. The adulterate petroleum ether in the oil at 40–60°C was purified by evaporating at 50°C with a Rotary Evaporator. Finally, the extracted botanical oil was refrigerated until it was used at 4 °C.
2.7. Preparation of the aqueous extracts
The powder of the prepared botanicals was divided into 50, 70, and 90 g by weight. Each measured powder mass was added to the flask jars of the five-volume holding capacity. One liter of distilled water was added into each flask jar for dissolving and mixing the powder for shaking. Then, the flask jars were sealed tightly with aluminum film. It was then shaken in shaking incubators for 24 hours to dissolve the mixtures uniformly at a speed of 80 shakes per minute. After 24 hours of shaking, the incubator was siphoned off, the dissolved mixture filtered by using Whatman paper for 1 hour to remove the larger pieces, and the liquid percolated into the cleaned flask jars was served as a stock solution of insecticides. Every botanical extracted in such a way was applied at a 5% rate per 100 ml of tap water.
2.8. Preparation of leaf discs
The leaf discs were made from sterilized cotton wool (used for moistening common bean leaves), fresh common bean leaves with a 4 cm x 4 cm dimension (serving as a food for TSSM), and toilet tissue paper. The toilet paper was made to budge at every dimension of the leaf disc to control the escape of TSSM from the petri dish gorge. The used Petri dish has a diameter of nine centimeters.
2.9. Bioassay of synthetic insecticides under laboratory conditions
Methods of application: A synthetic insecticide was sprayed on the TSSM’s twenty-five adults and ten eggs. The dipping treatment was performed for 5 seconds on 30 adults and 10 eggs. Before applying pesticides, the TSSM adults and eggs were introduced to the prepared leaf disks described in 2.8. Two gravid females were introduced onto the prepared leaf discs to lay eggs for two days to test the efficacy of synthetic insecticides against eggs. The 10 laid eggs were counted after two days and left in each leaf disc per petri dish for spraying. The amount of insecticide used per experimental unit was 0.5 mL. It was applied with a sprayer bottle at a 90-degree angle at a distance of 20 cm, as Belay et al. (Citation2018) demonstrated, while the untreated control was applied with tap water alone. The mite was considered dead if it did not exhibit normal behavior, such as having immobile appendages, whereas the eggs were considered dead if they shrunk, broke, changed color, spoilage/rotten, or dried.
2.10. Collected data
The mortality rate of TSSM adults was determined after 24, 48, and 72 hours. However, the eggs were checked and counted for the 12th day after being sprayed.
2.11. Data analysis
All data collected were statistically analyzed using SAS version 9.0 and subjected to a general linear model (GLM) procedure. For all analyses, a 5% level of significance was used, and Tukey’s test was used to separate the groups.
2.12. Bioassay of botanicals under laboratory conditions
2.12.1. Leaf spray bioassay of TSSM adults
The botanical oils and aqueous extracts were applied to 25 adults and 10 eggs of TSSM under laboratory conditions on the prepared leaf discs described in 2.8. Adults were given 0.5 ml of botanical extracts via spray bottles at a distance of 20 cm and an angle of 90 degrees. The immobile TSSM adults were considered mortal and their deaths were recorded 24 hours, 48 hours, and 72 hours after being tested with a fine brush.
2.12.2. Leaf spray bioassay of eggs
The 10 TSSM eggs laid on the haricot bean leaves were tested by applying the botanical insecticides listed in Table to the prepared leaf discs as described in 2.8.
2.12.3. Leaf dip bioassay of adults
The botanical oils and aqueous extracts were made into 100 ml solutions and used to dip 30 TSSM adults for 5 seconds in Petri dishes while being supported with forceps. They were dipped and placed on the prepared medium described in section 2.8, while the treated control was dipped in 100 ml of pure tap water. Then, under a dissecting microscope, the unescaped TSSM adults on the dipped leaf discs were counted and recorded as the initial population numbers.
2.12.4. Collected data
The mortality rate of TSSM adults was determined after 24, 48, and 72 hours. However, the eggs were checked and counted for the 12th day after being sprayed.
2.12.5. Data analysis
All collected data were statistically analyzed using SAS version 9.0 and then subjected to the ANOVA general linear model (GLM) procedure. For all analyses, a 5% level of significance was used, and Tukey’s test was used to separate means.
2.13. Median Lethal Concentrations (LC50) of insecticides under laboratory conditions
To determine the death rates in the 50% TSSM population, the median lethal concentration (LC50) was calculated. These methods included leaf spray and dip bioassays of adults and eggs at three dose levels to investigate the LC50 values of each insecticide because they are the most stable point for comparisons and are easily estimated.
2.13.1. Data analysis
The collected data was subjected to probit analysis in accordance with Finney’s (Citation1971) work, which was also incorporated into Abbott’s (Citation1925) correction formula, to determine mortality. The SPSS package (SPSS, Citation2007) was used to estimate the LC50 value, regression coefficient (slope), and data were transformed to log, intercept and its standard error, Pearson goodness of fit chi-square, expected mortality, and 95% confidence limits (95% CL) for an effective level of concentration. The LC50 values of botanical extracts and synthetic pesticides required to kill 50% of the treated TSSM population are calculated 72 hours after application in the case of adults, but 12 days after application in the case of eggs.
2.13.2. Bioassay of selected insecticides against TSSMs under greenhouse conditions
The Bubu potato tubers were planted in the greenhouse at Haramaya University, Rare Research Site. This experiment consisted of 12 treatments (Tables ). A total of 4 kilograms of standard nursery soil, consisting of silt, sand, and manure at a 2:1:1 ratio per pot, was used. One potato tuber was planted per pot. Every two days, 250 ml of tap water was watered into each pot. Manually, in every planted pot, the weed management was done as necessary. The TSSM was infested after three to four weeks of potato plants’ emerging to get enough populations. The treatment application was begun on the five-week-old potato plants. It was continued for three consecutive weeks in the greenhouse by using handheld sprayers that could hold one litre of the solution either alone or in combination.
Table 2. Lists of insecticides applied under greenhouse conditions
Table 3. List of treatments and schedule of application under greenhouse
The number of TSSM was counted before and after one day of spraying. The TSSM was deemed dead when no movement was detected under a dissecting microscope by probing its legs with a fine camel brush, whereas the eggs were deemed dead when they shrank, broke, and dried.
2.13.3. Collected data
The number of healthy and dead TSSM adults, nymphs, and eggs was counted before and after one and seven days of spraying.
2.13.4. Data analysis
The mortality percent reduction of TSSM was calculated using Abbott’s (Citation1925) formula, as shown below.
All collected data were statistically analyzed using SAS version 9.0 (SAS (Statistical Analysis System) Institute, Inc, Citation2002) and the ANOVA general linear model (GLM) procedure. For all analyses, a 5% level of significance was used, and means were separated using Tukey’s test.
3. Results and discussion
3.1. Bioassay of synthetic insecticides against TSSM in laboratory
3.1.1. Adults leaf discs spray
The application of the Amitraz, Emamectin benzoate and Profenofos insecticides to the TSSMs indicated a significant mortality of their eggs, nymphs, and adults. Hence, they were effective in reducing the TSSM population in potato plants when compared with untreated control. But there were also highly significant (p < 0.0001) different among their dose rates in causing mortality after 24 hr (F = 100.26; DF = 9), 48 hr (F = 85.6; DF = 9) and 72 hr (F = 76.77; DF = 9) of application (Table ).
Table 4. Mean percentages (± SEM) mortality of TSSMs adult after 24, 48 and 72 synthetic pesticides spraying in laboratory
The highest mortality percentage was caused by Emamectin benzoate at 80 µL. After 24, 48, and 72 hours of spraying, it caused 89, 90, and 97% adult mortality, respectively. Nonetheless, Amitraz at 40 µL caused the least mortality, followed by the untreated control. Adult mortality rates were 45, 52, and 58%, respectively, while untreated controls were 12.5, 22, and 28% within 24, 48, and 72 hours. Based on this discovery, it was confirmed in the laboratory that Emamectin benzoate at 80 µL and profenofos at 35 and 25 µL doses caused the highest percentage mortality of TSSM adults after three days of application when compared to Amitraz (Table ).
3.1.2. Bioassay of botanical insecticides against TSSM in laboratory
The percent reduction of TSSM adult was significantly different after 24 hours (F = 307.57; DF = 15; p 0.0001), 48 hours (F = 251.3; DF = 15; p 0.0001), and 72 hours (F = 49.89; DF = 15; p 0.0001). T. minuta leaf oil at 5% caused 78, 89, and 92% mortality of TSSM adults at 24, 48, and 72 hours of spraying, respectively (). A. indica seed oil at 3% caused 82, 86, and 97% mortality of TSSM adults at 24, 48, and 72 hours of spraying, respectively. The least mortality was recorded from the treatment applied by leaf aqueous extracts at 50 and 70 g/L of A. indica leaf and T. minuta leaf after 24 h, 48 h, and 72 hours of spray, respectively.
Table 5. Mean percentage (± SEM) mortality of TSSMs adult at 24, 48 and 72 hr. after botanical pesticides spray in laboratory
TSSM mortality, on the other hand, increased as botanical concentration and exposure hours increased. According to this finding, A. indica seed oil at 5 and 3%, followed by T. minuta leaf oil at 5%, resulted in better mortality after 3 days of application in decreasing order. This meant that A. indica seed oil was more toxic to TSSM adults than the other botanical extracts tested. This result also indicated that A. indica seed oil at 5 and 3% concentration levels, as well as T. minuta leaf oil at 5% concentration levels, are promising in reducing TSSM adults in potato plants. However, the toxicity of A. indica seed oil against TSSM adults was greater than that of T. minuta leaf oil. Farmers can therefore use this dose to reduce the number of TSSM adults on potato farms.
Cenusa et al. (Citation2016) reported that A. indica seed oil could reduce the number of red spider mites, which is consistent with this study. According to Venzon et al. (Citation2008), the use of A. indica extract resulted in higher mite density mortality than the untreated control (water). Brito et al. (Citation2006) also found that neem extracts reduced T. urticae adults and eggs more effectively than Phytpseiulus. persimilis at lower doses. This finding confirmed that, even at lower doses, neem extract was more effective against TSSM than P. persimilis. Tsolakis et al. (Citation2002) found that neem oil causes 100% mortality of young female TSSMs and significantly reduces oviposition in the laboratory.
Mmbone et al. (Citation2014) reported that T. minuta leaf extract caused 55% mortality of T. urticae within 24 hours. Additionally, T. minuta oil’s mode of action was confirmed and registered by the United States Environmental Agency as a repellent. This report also agreed with the report of Jankowska et al. (Citation2009), which indicated that T. minuta is used to repel insects when grown with a host crop rather than monocropping of cabbage. The active ingredients of T. minuta extracts are also used to control other arthropods such as whiteflies, aphids, thrips, mealy bugs, and scale insects on a different variety of crops (USEPA, Citation2012). For instance, it was reported that T. minuta extracts were effective on the fecundity of cabbage aphids (Brevicoryne brassicae (Phoofolo et al., Citation2013) and also reduced in reproductive potential or fecundity (Muzemu et al., Citation2011).
minuta leaf were also effective in reducing the Indian mustard aphid (Lipaphis erysimi Kalt) by 96% after spraying (Ali et al., Citation2010). Another study discovered that its extraction caused larval mortality in sugar beet root maggots (Tetanopsmyopae formis) at higher concentrations and prevented pupation at lower concentrations (Dunkel et al., Citation2010). T. minuta extract was also used to control stored product beetles (Keita et al., Citation2000) andmosquitoes (Basabose et al., Citation1997)
The current finding also agreed with the findings of Mmbone et al. (Citation2014) and Premalatha et al. (Citation2009), who reported the acaricidal activity of aqueous extracts of T. minuta to TSSM in the laboratory. The efficiency of plant extracts for the control of mites is considered satisfactory when mortality rates are higher than 60% and excellent when they exceed 80% (Potenza et al., Citation2006). Therefore, the A. indica seed oil extract was satisfactory in TSSM management, with an average control efficiency of 81.56% over 72 hours. This shows that, if used correctly, the botanical acaricide could be extremely efficient in the integrated management of arthropod pests. Naturally, their products contain compounds that degrade more rapidly than most synthetic chemicals, signifying a decrease in the chance of pest arthropods developing resistance, minor residual effects, and low toxicity to humans (Veronez et al., Citation2012).
3.1.3. Leaf dip bioassay of synthetic insecticides
Table shows the mean mortality of TSSM adults after 24 hours (F = 45.67; Df = 9; p 0.0001), 48 hours (F = 109.79; Df = 9; p 0.0001), and 72 hours (F = 74.25; DF = 9; P 0.0001). The maximum percentage of TSSM adults’ mortality after one day of dipping was recorded by Emamectin benzoate at concentrations of 80 µL (97.5%), 60 µL (97%), and 40(97%) L, respectively. After two days of dipping, Amitraz 80 µL, Emamectin benzoate 60 µL, and profenofos 25 and 35 µL caused the highest percentage mortality (100%), but after three days of application, there was no significant difference at a 5% significance level (P 0.0001) using the Tukeys test (p 0.05), while Amitraz 40 µL caused the lowest mortality (53, 67, and 89% mortality), followed by untreated control (7, 19 and 22% mortality) within 24 hours., This data confirmed that Amitraz 80 µL, Emamectin benzoate 60 µL, Profenofos 25, and 35 µL greatly reduced the TSSM adults on potato plants. In all the treatments, the mortality rate increased as the days of observation and chemical concentrations increased. These results coincided with the finding of Belay et al. (Citation2018), who concluded that Profenofos and Amitraz were effective in controlling TSSM. All the treatments were significantly different from the control Tukeys test (p 0.05) within 24, 48, and 72 hours (Table ).
Table 6. Mean percentage (± SEM) mortality of TSSM adults at 24, 48 and 72 hr. after pesticides dipping in laboratory
The current study’s findings were consistent with those of Ruth Buba et al. (Citation1992), who discovered that the TSSM population was susceptible to Emamectin benzoate. Rabea (Citation2009) also reported that profenofos caused the lowest TSSM mortality, which could be attributed to the lower dose he used in comparison to the current study.
3.1.4. Leaf dip bioassay of botanical insecticides
After 24 hours (F = 40.5; DF = 15; p0.0001), 48 hours (F = 55.02; DF = 15; p0.0001), and 72 hours (F = 51.32; DF = 15; p0.0001) of application, all of the tested botanical pesticides significantly increased the mortality of potato TSSM adults compared to the control (Table ). The highest percentage mortality was recorded from A. indica seed oil at 5% concentration (81.13, 88.93, and 95.73% mortality) within 24, 48, and 72 hours after dipped, respectively. After 24, 48, and 72 hours of observation, the least mortality of (20.33, 35.1, and 47%) TSSM adults was recorded from A. indica leaf oil 1%, followed by untreated control (12, 18, and 21% mortality).
Table 7. Means percentage (± SEM) mortality of TSSMs adult dipped by botanical pesticides in laboratory
The result of this finding confirmed that A. indica seed oil at 5, 3, 1%, and 90 g/L A. indica aqueous leaf extract at 5% performed better in the reduction of TSSM adults after 3 days of exposure in decreasing order. From both adult leaf disc and dip methods, A. indica seed oil at 5% concentration performed better than the other tested botanical pesticides, and it was found promising to use against TSSM. It is also confirmed that A. indica seed oil and T. minuta leaf oil 1, 3, and 5%, and their aqueous extracts of 50, 70, and 90 g/L significantly reduced the TSSM adults in increasing order of their concentration when compared with the control (water) in Table . For all the treatments, mortality was clearly time-dependent, i.e., mortality increased proportionately with time duration.
3.1.5. Eggs leaf disc spray with insecticides
The TSSM eggs were observed for 12 days after spraying with synthetic insecticides at three different dose levels. According to the counted mortality, all of the tested doses caused a significant difference in TSSM egg mortality (at p 0.05; Tukeys test; DF = 9; F = 100.89; P 0.0001) (Table ). Amitraz at doses of 80 and 60 µL killed 92.5 and 82.5% of TSSM eggs, respectively. Despite this, Emamectin benzoate at a 40 µL dose resulted in the lowest mortality (37.5%), followed by the untreated control (2.5%). The untreated control had the highest percentage of hatched eggs (97.5%). It has been confirmed that Amitraz at 80 µL caused the greatest reduction in the production of TSSM offspring.
Table 8. Mean percentage (+SEM) mortality of TSSMs eggs` sprayed by synthetic pesticides in laboratory
3.1.6. Bioassay of Botanicals against TSSM eggs
The TSSM eggs were observed and counted daily for 12 days after spraying botanicals at three dose levels. Based on the count, all the tested botanical doses exhibited a significant difference in causing mortality of TSSM eggs (at p 0.05; Tukeys test; DF = 9; F = 132.96; P 0.0001; Table ).
Table 9. Mean percentage (+SEM) mortality of TSSMs eggs after application of botanical pesticides in laboratory
T. minuta leaf oil at 5% also caused the highest mortality percentage of TSSM eggs (83.75%), followed by T. minuta leaf oil at 3% (73.75% mortality) and 1% (67.5% mortality). The lower mortality of TSSM eggs was counted from A. indica leaf oil at 1% (18.25%). The highest hatching of TSSM eggs to offspring was recorded from A. indica seed oil at 1% (81.75%), followed by untreated control (92.5%; Table ). The extracts of A. indica had a lower effect on TSSM eggs than T. minuta when compared after 12 days of exposure. There were not enough previous studies conducted on these botanicals to compare or contrast the results. But the study is different from the previous report by Mwandila et al. (Citation2009), who stated that A. indica had significant effects on the hatching of red spider mites’ eggs. The current study is supported by the findings of Bhuyan et al. (Citation2017), who discovered that Azadirachtin 5% reduces hatchability by 70% when compared to 58.75%. In contrast, Martinez-Villar et al. (Citation2005) found that Azadirachtin had no effect on TSSM fertility or offspring development. Pentane extracts of neem oil were effective against TSSM’s nymphal and ovicidal effects (Sanguanpong and Schmutterer, Citation1992). Sarmah (Citation2016) also found that higher doses of neem extract had higher ovicidal activity than lower doses (6–10%: 25% at 5%, 56.67% at 8, and 65% at 10%). Mourao et al. (Citation2004) discovered a significant reduction in TSSM egg (25%) at 4% and higher percent doses of neem kernel extracts.
Table 10. Median lethal concentrations (LC50) toxicity of sprayed synthetic pesticides against TSSM adults after 72 hours
3.2. Median Lethal Dose (LC50) for each insecticide in laboratory
3.2.1. Toxicity of sprayed synthetic insecticide against TSSM Adults
Table shows the LC50 results from probit analysis after 72 hours of application. Emamectin benzoate (EB) was found to be more toxic to TSSM adults at lower doses (33.425 µL) than Amitraz (47.388 µL), with significantly different values after 72 hours of spray. The synthetic insecticides tested were toxic to TSSM adults, but the degree of toxicity varied with dose. This finding was contradicted by Islam’s (Citation2019) discovery that TSSM was moderately susceptible to EB. Because the 95% confidence limits of the estimated doses did not overlap, the lethal dose values were considered significantly different (AL-Antary et al., Citation2012; Brown et al., Citation2017).
3.2.2. Determination of LC50 values for each sprayed botanicals
The results of the determined LC50 of sprayed botanicals on TSSM adults after 72 hrs are presented in Table and show a significant difference between the LC50 values of each evaluated botanical. The result indicated that A. indica seed oil was the most toxic to TSSM adults (0.778%) than T. minuta leaf oil (0.938%) and A. indica leaf oil (4.349%). The result also indicated that the LC50 of T. minuta leaf aqueous extract was more toxic than A. indica leaf aqueous extracts after 72 hrs of application. This finding agreed with the finding of Martinez-Villar et al. (Citation2005), who reported that azadirachtin was the most toxic compound against spider mites with an LC50 value of 14.90 mg/L on jute leaf at 24 hours. Mmbone et al. (Citation2014) also found that T. minuta aqueous extracts at 150 g/L had good insecticide potential and could repel TSSM after 48 hours of application.
Table 11. Median lethal concentration (LC50) of different botanical extracts on TSSM adults after 72 hr of exposure
3.2.3. Determination of LC50 for each dipped synthetic pesticides
Table shows the results of probit analysis for determining the LC50 after 72 hours of exposure. When compared to Amitraz and profenofos after 72 hours of dipping, EB at a lower concentration (9.431 µL) was required to kill 50% of the TSSM population. The LC50 values for profenofos, EB, and Amitraz did not differ significantly. Because the 95% confidence limit values overlapped, the results indicated that the tested pesticides were not significantly different in dose responses for causing TSSM adult mortality. This discovery revealed that EB is more toxic to TSSM adults when dipped than when sprayed ().
Table 12. Median lethal concentration (LC50) of different Synthetic pesticides on TSSM adults after 72 hr of dipped
3.2.4. Determination LC50 for each dipped botanicals
Table shows the results of probit analysis for determining LC50 after 72 hours of exposure. Because the 95% confidence limit values overlapping, the LC50 of all the tested botanical oils were not significantly different from one another. The LC50 of A. indica seed oil is the most toxic to TSSM (2.14%), followed by A. indica leaf oil (2.31%). After 72 hours of dip, A. indica oil was more toxic than T. minuta leaf oil against TSSM adults, but the toxicity of A. indica leaf aqueous extracts was statistically insignificant (Table ).
Table 13. Median lethal concentration (LC50) of different botanicals at different concentration on TSSM adults after 72 hr of exposure
3.2.5. Determination of LC50 of leaf spray bioassay of synthetic pesticides against TSSM Eggs
The relative toxicity of synthetic pesticides at medial lethal concentrations (LC50) determined by probit analysis after 72 hours of spraying is shown in Table . The Pearson X2-values indicated that the probit model was fit for all of the synthetic pesticides tested. According to Finney (Citation1971), in basic binary bioassays, comparisons are made at the LC50 because it is the most stable point for comparison and is simple to estimate. On TSSM eggs, the LC50 values of the pesticides tested differed significantly (P 0.05). In laboratory tests, the estimated LC50 for Amitraz was 24.350 (17.993–29.166), Emamectin benzoate 54.146 (47.988–60.069), and Profenofos 12.737 (9.103–15.288) µL per Petri dish, in decreasing order of susceptibility.
Table 14. Median lethal concentration (LC50) of different synthetic pesticides on TSSM eggs after 72 hr of exposure
After 12 days, the TSSM eggs were more susceptible to Amitraz than Emamectin benzoate and Profenofos based on the LC50 confidence limit (CL). This finding confirmed Amitraz’s toxicity to TSSM eggs over EB and Profenofos. The information gleaned from this study could be used as a tool in integrated pest management strategies to effectively control the pest. According to Roy et al. (Citation2012), the descending order of ovicidal toxicity of the acaricides tested to O. coffeae eggs was fenazaquin, followed by profenofos, propargite, fenpropathrin, ethion, dicofol, and abamectin based on LC50 values.
3.2.6. Leaf spray bioassays for the determination of LC50 botanicals against TSSM Eggs
The LC50 values of botanicals on TSSM eggs are shown in Table . For all of the botanicals tested, the p-values in the goodness of fit statistic for the Pearson X2 indicated a good fit with the probit model. On TSSM eggs, there were significant differences between the botanicals tested. The LC50 of A. indica leaf aqueous extracts (5.499 (5.151–6.094) g/L) was not statistically significant (Table ). To kill 50% of TSSM eggs in the laboratory, T. minuta leaf aqueous extracts required 63.897 (59.126–68.332) g/L, A. indica leaf oil 9.072 (5.069–110.39%), A. indica seed oil 1.162 (0.081–1.808) %, and 0.239 (0.054–0.469) %.
Table 15. Median lethal concentration (LC50) of different botanicals against TSSM eggs after 72 hr of exposure
Table 16. Mean % efficacy of the pesticides against TSSMs adults, immature and eggs in the greenhouse
3.3. In greenhouse bioassay of selected insecticides against TSSMs
The pesticides were applied weekly, and there was a significant difference in the reduction of eggs, immatures, and adults of TSSM after an application using Tukey’s test (p 0.05) and were indicated in Table .
3.3.1. Efficacy on eggs, immatures, and adults of T. urticae in the first round of spray
The highest mortality percentages were obtained from the combined half dose of profenofos and half dose of emamectin benzoate (EB) (93.43%), followed by Amitraz alone (92.72%), and one-third dose of Amitraz, profenofos, and EB (90.12%) after application (Table ). The moderate result was obtained from half doses of A. indica seed oil with profenofos (82.78%), half doses of EB and A. indica seed oil (88.74%), and EB alone (82.74%). A lower percentage reduction in mortality of adult TSSM was recorded in profenofos alone (77.04%), A. indica seed oil alone (65.72%), and one-third dose of A. indica seed oil, EB, and Amitraz (50.86%). The last kill was obtained in half doses of A. indica seed oil and Amitraz (49.14%) when compared with the untreated control (7.15%) after application. All potato plants sprayed with A. indica seed oil resulted in phytotoxicity. However, the phytotoxicity evidenced was not of economic severity and was even lower on the combined treatments. The phytotoxicity became lower and lower along with the A. indica seed oil from the first to the third spray. Half doses of EB and profenofos resulted in the highest percentage mortality of TSSM immature (93.480%), followed by a half rate of EB and A. indica seed oil (90.46%), Amitraz alone (80.84%), and a half dose of A. indica seed oil and profenofos (78.92%), while A. indica seed oil alone (48%), a half dose of A. indica seed oil and Amitraz (42.4%). Half the rate of A. indica seed oil and profenofos was the most effective against the eggs of TSSM (87.1%) on sprayed potato, followed by half the rates of EB and A. indica seed oil (75%) and half the rate of profenofos and EB (72.55%). A. indica seed oil alone and its half-rate with Amitraz caused the lowest percentage reduction (49.54%) of TSSM eggs, followed by the untreated control (5.72%; Table ).
3.3.2. Efficacy on EGGS, IMMATURE AND Adults of T. urticae in the second round spray
In the second round of spray, half the rate of EB and A. indica seed oil caused the highest percentage of mortality (97.4%) of TSSM adults. This was followed by half the rate of profenofos and EB (95%), Amitraz alone (94.47%), one-third rates of Amitraz, profenofos, and EB (92.4%), and half the rate of A. indica seed oil and profenofos (90%). The lowest adult reduction was recorded from a one-third dose combination of A. indica seed oil, EB, and Amitraz (55.2%), followed by the untreated control (7.49%; Table ).
The immature of TSSM was highly reduced by the application of half the doses of EB and A. indica seed oil (99.91%), followed by half-rate application of profenofos and EB (96.89%) and half doses of EB and A. indica seed oil (93.2%). The least effective was recorded from untreated control (6.551%) Table ).
Half doses of EB and A. indica seed oil rate application caused the highest ovicidal effects (98.97%) after the second spray, followed by EB alone (95.37%) and half doses of profenofos and EB (93.84%). Amitraz alone (90.42%), and profenofos alone (90.31%) were moderate activities, while the least amount of eggs was recorded from the application of half doses of A. indica seed oil and Amitraz (56.02%), followed by untreated control (7.93%; Table ).
3.3.3. Efficacy on eggs, immature, and adults of T. urticae in the third round spray
After the third application of synthetic and botanical pesticides, there were significant differences among the applied treatments (p 0.0001). EB alone; half doses of EB and A. indica seed oil; half doses of Amitraz and EB; one-third of the doses of Amitraz, profenofos, and EB; and half the doses of profenofos and EB caused the highest percentage mortality of TSSM adults (100%). The potato, therefore, became free from infestation after the third application in the greenhouse. The potato applied with one-third the rates of A. indica seed oil EB and Amitraz also reduced adult red mites (99.88%), while the least effective was recorded from a potato applied by A. indica seed oil alone (81.6%), followed by untreated control (10.88%; Table ). The highest reduction percentage of TSSM immature was recorded from the application of Profenofos, half doses of A. indica seed oil and Amitraz, half doses of A. indica seed oil and profenofos, half doses of Amitraz and EB, one-third dose of Amitraz, profenofos and EB, and half doses of profenofos and EB on potatoes in the greenhouse (100%) after three applications. A. indica seed oil was less effective comparatively (81.2%), followed by the untreated control (8.97%; Table ).
EB alone, half the rate of EB and A. indica seed oil, half doses of Amitraz and EB, one-third doses of Amitraz, profenofos, and EB, and profenofos alone caused the highest reduction of TSSM eggs on potato plants after the third spray (100%). A. indica seed oil alone caused the least percent reduction of eggs (75.2%), followed by the untreated control (8.89%). The potato plants killed completely by TSSM that were applied by tap water alone after the third application. Therefore, based on this finding, it was confirmed that the application of Azadirachtin seed oil alone was not efficient to manage T. urticae on potato plants. Its combination with synthetic pesticide was resulted in a better reduction of TSSM than applied alone. The potato plant grown with tap water was completely destroyed by TSSM. The results showed that all the tested pesticides had the capacity to reduce the TSSM when applied alone and in combinations compared with control. To generalize the greenhouse finding, the combination of profenofos and EB, one-third doses of Amitraz, profenofos, and EB, and half doses of Amitraz and EB caused a high reduction of adults and immatures. The current study was very similar to the report of Ghosh and Chakraborty (Citation2014), who confirmed that a mixed formulation of Azadirachtin and chemical pesticide, dicofol (73.42%), resulted in better suppression of TSSM than when applied individually (45.57% suppression). Martinez-Villar et al. (Citation2005) also reported that Azadirachtin affects fecundity but had no effect on fertility and offspring development of TSSM. In contrast to this finding, in the current study, Azadirachtin extract affected immature development and egg hatching. Belay et al. (Citation2018) reported that Amitraz and profenofos were effective against TSSM on the potato plants. Profenofos was reported from China to manage spider mites as acaricides (Dandan et al., Citation2018). Bernardi et al. (Citation2012) reported that Azadirachtin reduces fecundity by 50% in the greenhouse.
Mixtures of pesticides are more toxic than individual constituents because they are more difficult to detoxify than a single molecule (Koul, Citation2008). The mixture of two or more pesticides is very common among farmers to control the pest population in the tank mixture. Complex pesticides gave the best results in control with varying susceptibilities to the different components of mixtures. Pests that are resistant to one or more pesticides may be susceptible to a combination of toxicants and synergism may be exhibited by the components by identifying their compatibility (Das, Citation2014). Badawy et al. (Citation2022); El-Sherbeni et al. (Citation2019), and Khan et al. (Citation2013) were reported that the mixture is allowable for pesticides belonging to different categories but more toxic against pests. Saminathan et al. (Citation2011) reported that the combination of Azadirachtin 1EC at 1 ml/lit with half-dose profenofos was more effective than when applied alone in percent plant infestation and population of mealybug, Coccidohystrix insolita. They also reported that the bio-pesticide combinations with synthetic pesticides resulted in controlling the whitefly population without apparent severity to plants and reduced the load of pesticides, costs, and natural enemy damage (Guleria, Citation2013).
4. Summary and conclusions
Under favorable dry weather conditions, T.urticae is the potato’s production constraint. Unless proper management techniques are developed to mitigate the problem during favorable weather conditions, it causes devastating yield losses. The goal of this study was to manage TSSM on potato plants in the Haramaya University laboratory and under greenhouse conditions. Amitraz, Profenofos, Emamectin benzoate, A.indica seed oil, A.indica leaf oil, T.minuta leaf oil, leaf aqueous extracts of A.indica and T.minuta at three doses, and the untreated control were evaluated against adults and eggs of T.urticae in the laboratory using leaf dipping and spraying bioassays, with Emamectin benzoate 80 µL, Amitraz.
For TSSM adults and eggs, the most effective botanicals were A.indica seed oil at 5%, T.minuta leaf oil at 5%, and T.minuta leaf aqueous extract at 90g/L, respectively. The laboratory findings confirmed that the TSSM mortality increased with sampling time and concentration. The LC50 of EB determined by leaf spray bioassay demonstrated that EB was more toxic to TSSM adults than Amitraz and profenofos. In contrast to this discovery, Amitraz is more toxic to TSSM eggs than to TSSM adults when compared to the synthetic pesticides used. Concentrations of A.indica seed oil extract is highly toxic and effective in killing TSSM adults within 72hours of application.
The leaf spray bioassay of botanicals indicated that the LC50 of A. indica seed oil is more toxic to TSSM adults than T. minuta leaf oil. From the present investigation in the greenhouse, it can be concluded that the combination of Amitraz with Emamectin benzoate, Amitraz with Emamectin benzoate and profenofos, and profenofos with Emamectin benzoate resulted in better mortality (100%) of adults, immatures, and eggs than their individual application on potatoes in the greenhouse. This pesticide had no phytotoxic effects on potato plants, except when it was combined with A. indica seed oil. Based on this finding, it could be recommended that the farmers use Amitraz 80 µL (at 2.55 L/ha), Emamectin benzoate 80 µL (at 2 L/ha), Profenofos 35 µL (at 0.9 L/ha), A. indica seed oil at 5% (or alternatively, T. minuta leaf oil 5%) either alone or in combination to manage TSSM adults, but their combination is more effective than alone. Considering the strong acaricidal activity of petroleum ether and aqueous extracts, further studies are required to identify the active compounds. Under laboratory and greenhouse conditions, the pesticides tested significantly reduced TSSM numbers. More research is needed under a variety of field conditions to more accurately assess effective rate doses, timings, and formulations of botanicals and synthetic pesticides. Furthermore, when combined with other management techniques, these pesticides would undoubtedly become more effective. The repellency of A. indica seed and leaf oil, T. minuta leaf oil, and their aqueous extracts against TSSM, as well as the determination of their median lethal concentrations, is required for future research to identify its bioactivity and toxic substances with a pesticide effect on TSSM. As a result, this research finding implicates TSSM management in potato crops, and it was hoped that it would provide farmers with information for sustainable TSSM management in potato cultivated areas. According to research, providing farmers with a resistant variety of potato plants, chemical pesticides, irrigation water, and an improved variety of potato plants will help them increase potato plant production and productivity by reducing yield losses caused by TSSM. Seasonal abundance, the effect of microclimate, natural enemies, and management practices on pest population dynamics, and the economics of two-spotted spider mites have also been proposed in the country’s major potato-growing areas. More research is suggested for future work on farms, with multiple locations to manage TSSM under field conditions.
Disclosure statement
No potential conflict of interest was reported by the author(s).
Additional information
Funding
References
- Abbott, W. S. (1925). A method of computing the effectiveness of an insecticide. Journal of Economic Entomology, 18(2), 265–22. https://doi.org/10.1093/jee/18.2.265a
- AL-Antary, T. M., Kamel Al-Lala, M. R., & Abdel-Wali, M. I. (2012). Response of seven populations of the two-spotted spider mite (Tetranychus urticae Koch) for amitraz acaricide on) Cucumber (Cucumis sativus L.) under plastic houses in Jordan. Advances in Environmental Biology, 6(3), 951–954.
- Ali, A., Rizvi, P. Q., & Khan, F. R. (2010). Bio-efficacy of some plant leaf extracts against mustard aphid, Lipaphis erysimi Kalt on Indian mustard, Brassica juncea. Journal of Plant Protection Research, 50(2), 130–132. https://doi.org/10.2478/v10045-010-0022-4
- Badawy M E, Mahmoud M S and Khattab M M. (2022). Toxicity, joint action effect, and enzymatic assays of abamectin, chlorfenapyr, and pyridaben against the two-spotted spider mite Tetranychus urticae. JoBAZ, 83(1). https://doi.org/10.1186/s41936-022-00287-6
- Basabose, K. M., Bagalwa, M., & Chifundera, K. (1997). Anophelinocidal activity of volatile oil from Tagetes minuta L. (Asteraceae). Tropicultura, 15, 8–9.
- Belay, T., Goftishu, M., & Kassaye, A. (2018). Management of an emerging pest, Tetranychus urticae Koch (Acari: Tetranychidae), with pesticides in Eastern Ethiopia. African Crop Science Journal, 26(2), 291–304. https://doi.org/10.4314/acsj.v26i2.10
- Bernardi, D., Botton, M., Cunha, U. S., Bernardi, O., Malausa, T., Garciac, M. S., & Nava, D. E. (2012). Effects of azadirachtin on Tetranychus urticae (Acari: Tetranychidae) and its compatibility with predatory mites (Acari: Phytoseiidae) on strawberry. Pest Management Science, 69(1), 75–80. https://doi.org/10.1002/ps.3364
- Bezabih, E., & Mengistu, N. (2011). Potato value chain analysis and development in Ethiopia case of Tigray and SNNP regions. International Potato Centre (CIP-Ethiopia).
- Bhuyan, K. K., Saikia, G. K., & Deka, M. K. (2017). Evaluation of indigenous biopesticides against Red Spider Mite, Oligonychus coffeae (Nietner) in tea. Journal of Entomology and Zoology Studies, 5(2), 731–735.
- Brito, H. M., Gondim, M. G. C., Oliveira, J. V., & Camara, C. A. G. (2006). Toxicidae De Formulacoses De Nim (Azadirachta Indica A. Juss) Ao Acaro-Rajado E a Euseius Altus De Leon E Phytoseiulus Marcopilis (Banks) (Acari: Phytoseiidae). Neotropical Entomology, 35(40), 500–505. https://doi.org/10.1590/S1519-566X2006000400012
- Brown, S., Kerns, D. L., Gore, J., Lorenz, G., & Stewart, S. (2017). Susceptibility of twospotted spider mites (Tetranychus urticae) to abamectin in Mid-south cotton. Crop Protection, 98, 179–183. https://doi.org/10.1016/j.cropro.2017.04.002
- Cenusa, A. E., Şovarel, G., Costache, M., Bratu, E., & Velea, M. (2016). Effect of some “Bio-insecticides” used against two spotted spider mites (Tetranychus urticae Koch.). In the cucumbers crop under plastic tunnel conditions. Scientific Papers. Series B, Horticulture. Vol, LX, 139–143.
- CSA (Central Statistical Authority). (2010). Report on area and production of major crops (private peasant holdings, Meher season) in Ethiopia. Agriculture sample survey 2017/18. The FDRE Statistical Bulletin, 1, 1–53.
- CSA (Central Statistical Authority). (2014). Agriculture sample survey 2013/2014. Report on Area and production of major crops (Private peasant holdings, Meher season). Addis Ababa, Ethiopia. The FDRE Statistical Bulletin, 01–532.
- Dandan, X., Yanyan, H., Youjun, Z., Wen, X., Qingjun, W., & Shaoli, W. (2018). Status of pesticide resistance and associated mutations in the two-spotted spider mite, Tetranychus urticae, in China.J. Pesticide Biochemistry and Physiology, 150, 89–96. https://doi.org/10.1016/j.pestbp.2018.07.008
- Das, S. K. (2014). Scope and relevance of using pesticide mixtures in crop protection: A critical review. International Journal of Environmental Science and Toxicology Research, 2(5), 119–123.
- Demissie, Y. T. (2019). Integrated potato (Solanum tuberosum L.) late blight (Phytophthora infestans) disease management in Ethiopia. American Journal of BioScience, 7(6), 123–130. https://doi.org/10.11648/j.ajbio.20190706.16
- Dunkel, F. V., Jaronski, S. T., Sedlak, C. W., Meiler, S. U., & Veo, K. D. (2010). Effects of steam-distilled shoot extract of tagetes minuta (Asterales: Asteraceae) and Entomopathogenic fungi on larval Tetanops myopaeformis. Environmental Entomology, 39(3), 979–988. https://doi.org/10.1603/EN09259
- El-Sherbeni A El-Hakeem, Khaleid M Sengab, AbdAllah S Abd and Ali O Saber. (2019). Effect of some insecticides alone and in combination with salicylic acid against aphid, Aphis gossypii, and whitefly Bemisia tabaci on the cotton field. Bull Natl Res Cent, 43(1), 10.1186/s42269-019-0103-0
- FAOSTAT. (2021). World food and agricultural organization data of statistics. Food and agricultural organization. http://www.fao.org/faostat/en/#data
- Ferdu, A., Bayeh, M., Emana, G., Temesgen, A., Eyob, T., Messele, G., & Brook, W. (2009). Review of entomological research on root and tuber crops in Ethiopia. In T. Abraham (Ed.), Increasing crop production through improved plant protection (Vol. II, pp. 1–46). PPSE and EIAR.
- Finney, G. (1971). Probit Analysis. Cambrige University Press.
- Ghosh, S., & Chakraborty, K. (2014). Bio-efficacy of plant extracts against red spider mite (Tetranychus Sp.) infesting brinjal (Solanum Melongena L.). Research Journal of Agricultural and Environmental Sciences, 1(1), 26–31.
- Gildemacher, P. R., Demo, P., Barker, I., Kaguongo, W., Woldegiorgis, G., Wagoire, W. W., Wakahiu, M., Leeuwis, C., & Struik, P. C. (2009). A description of seed potato systems in Kenya, Uganda and Ethiopia. American Journal of Potato Research, 86(5), 373–382. https://doi.org/10.1007/s12230-009-9092-0
- Goftishu, M., Dejene, M., Kassaye, A., & Belay, T. (2016). Red spider mite, Tetranychus urticae Koch (Arachnida: Acari-Tetranychidae): A threatening pest to potato (Solanum Tuberosum L.) production in Eastern Ethiopia. Pest Management Journal of Ethiopia, 19, 53–59.
- Grbic, M., Van Leeuwen, T., Clark, R. M., Rombauts, S., Rouzé, P., Grbić, V., Osborne, E. J., Dermauw, W., Ngoc, P. C. T., & Ortego, F. (2011). The genome of Tetranychus urticae reveals herbivorous pest adaptations. Nature, 479, 487–492. https://doi.org/10.1038/nature10640
- Guleria, S. J. (2013). Field efficacy of biopesticides and pesticide combinations against whitefly infesting gerbera. Munis Entomology & Zoology, 8(2), 895–899.
- Islam, T. (2019). Host plant-induced susceptibility of two-spotted spider mite Tetranychus urticae (Acari: Tetranychidae) to some reduced-risk acaricides. American Journal of Agricultural and Biological Sciences, 14(1), 11–15. https://doi.org/10.3844/ajabssp.2019.11.15
- Ismail, M., Tag, H. M., & Rizk, M. (2019). Acaricidal, ovicidal, and repellent effects of Tagetes patula leaf extract against Tetranychus urticae Koch (Acari: Tetranychidae). Journal of Plant Protection Research, 59(2), 151–159. https://doi.org/10.24425/jppr.2019.129285
- Isman, M. B. (2006). Botanical Insecticides, deterrents, and repellents in modern agriculture and an increasingly regulated worldwide. Annual Review of Entomology, 51(1), 45–66. https://doi.org/10.1146/annurev.ento.51.110104.151146
- James, D. G., & Price, T. S. (2003). Fecundity in two spotted spider mite (Acari: Tetranychidae) is increased by direct and systemic exposure to imidacloprid. Journal of Economic Entomology, 95(4), 730–732.
- Jankowska, B., Poniedziałek, M., & Jędrszczyk, E. (2009). Effect of intercropping white cabbage with French Marigold (Tagetes patula nana L.) and Pot Marigold (Calendula officinalis L.) on the colonization of plants by pest insects. Folia Horticulturae, 21(1), 95–103. https://doi.org/10.2478/fhort-2013-0129
- Kafle, K., Shriwastav, C. P., & Marasini, M. (2019). Influence of integrated nutrient management practices on soil properties and yield of potato (Solanum tuberosum. L) in an inceptisol of Khajura, Banke. International Journal of Applied Sciences and Biotechnology, 7(3), 365–369. https://doi.org/10.3126/ijasbt.v7i3.25134
- Keita, S. M., Vincent, C., Schmit, J. P., Ramaswamy, S., & Belanger, A. (2000). Effect of various essential oils on Callosobruchus maculatus (F.) (Coleoptera: Bruchidae). Journal of Stored Products Research, 36(4), 355–364. https://doi.org/10.1016/S0022-474X(99)00055-7
- Khan H Azhar, Akram W, Shad S Ali, Lee J and Oliveira P Lagerblad. (2013). Correction: Insecticide Mixtures Could Enhance the Toxicity of Insecticides in a Resistant Dairy Population of Musca domestica L. PLoS ONE, 8(8), 10.1371/annotation/14f1545c-cbe3-4783-b7c6-f6f9e4c4a1f4
- Koul, O. (2008). Photochemical and insect control: An antifeedant approach. Critical Reviews in Plant Sciences, 27(1), 1–24. https://doi.org/10.1080/07352680802053908
- Liu, J., Sun, Z., Zou, Y., Li, W., He, F., Huang, X., Lin, C., Cai, Q., Wisniewski, M., & Wu, X. (2021). Pre-and postharvest measures used to control decay and mycotoxigenic fungi in potato (Solanum tuberosum L.) during storage. Critical Reviews in Food Science and Nutrition, 62(2), 415–428. https://doi.org/10.1080/10408398.2020.1818688
- Martinez-Villar, E., Sáenz-De-Cabezón, F. J., & Moreno-Grijalba, F. (2005). Effects of azadirachtin on the two-spotted spider mite, Tetranychus urticae (Acari: Tetranychidae). Experimental and Applied Acarology, 35(3), 215. https://doi.org/10.1007/s10493-004-5082-6
- Martin, D. E., Latheef, M., & Lo´pez, J. D. (2015). Evaluation of selected acaricides against two spotted spider mite (Acari: Tetranychidae) on greenhouse cotton using multispectral data. Experimental & Applied Acarology, 66(2), 227–245. https://doi.org/10.1007/s10493-015-9903-6
- Mmbone, S., Mulaa, M., Wanjala, F. M., Nyukuri, R. W., & Cheramgoi, E. (2014). Efficacy of Tagetes minuta and Tephrosia vogelii crude leaf extracts on Tetranychus urticae (Acari: Tetranychidae) and Aphis fabae (Homoptera: Aphididae). African Journal of Food Science and Technology, 5(8), 168–173.
- Mourao, S., Zanuncio, J. C., Pallini-Filho, A., Guedes, R. N. C., & Camargos, A. B. (2004). Toxicity of neem extracts (Azadirachta indica) To the Coffee Red Mite, Oligonchus Ilicis. Pesquisa-Agropecuaria Brasilieira, 39(8), 827–830. https://doi.org/10.1590/S0100-204X2004000800015
- Muzemu, S., Mvumi, B. M., Nyirenda, S. P. M., Sileshi, G. W., Sola, P., Chikukura, L., Kamanula, J. F., Belmain, S. R., & Stevenson, P. C. (2011). Pesticidal effects of indigenous plant extracts against rape aphids and tomato red spider mites. African Crop Science Conference Proceedings, 10: 171–173.
- Mwandila, N. J. K., Olivier, J., Visser, D., & Munthali, D. C. (2009). The Control of Red Spider Mites On Tomatoes Using. Neem and Syringa Extracts.African Journal of Agricultural Research, 8(8), 695–700.
- Phoofolo, M. W., Mabaleha, S., & Mekbib, S. B. (2013). Laboratory assessment of insecticidal properties of Tagetes minuta crude extracts against Brevicoryne brassicae on cabbage.Int. Journal of Entomology and Nematology, 1(6), 134–139.
- Potenza, M. R., Gomes, R. C. O., Jocys, T., Takematsu, A. P., & Ramos, A. C. O. (2006). Avaliação de produtos naturais para o controle de ácaro rajado Tetranychus urticae (Koch, 1836) (Acari: Tetranychidae) em casa de vegetação. Arquivos Do Instituto Biológico, 73(4), 455–459. https://doi.org/10.1590/1808-1657v73p4552006
- Premalatha, K., Nelson, S. J., Vishnupriya, R., Balakrishnan, S., & Santhana Krishnan, V. P. (2018). Acaricidal activity of plant extracts on two-spotted spider mite, Tetranychus urticae Koch (Acari: Tetranychidae). Journal of Entomology and Zoology Studies, 6(1), 1622–1625.
- Rabea, E. I. (2009). Comparative toxicity of five pesticides against Tetranychus urticae (Koch), Myzus persicae (Sulzer) and Aphis nerii (Boyer de Fonscolombe). Alexandria Science Exchange Journal, 30(3), 412–418. https://doi.org/10.21608/ASEJAIQJSAE.2009.3253
- Roy, S., Mukhopadhyay, A., & Guruswami Gurusubramanian, G. (2012). Chemical-based integrated approaches for the management of tea red spider mite, Oligonychus coffeae Nietner (Acari: Tetranychidae) in tea plantations of sub-Himalayan North Bengal, India. International Journal of Acarology, 38(1), 74–78. https://doi.org/10.1080/01647954.2011.590154
- Ruth Buba, B., Yao Eziah, V., Afreh-Nuamah, K., & Kyerematen, R. (2015). Susceptibility of two spotted spider mite Tetranychus urticae Koch (Acari; Tetranychidae) to some selected insecticide in the greater Accra region of Ghana. [Masters of Philosophy Degree in Entomology], Hons Crop Protection University of Maiduguri 1–119 (Accessed May, 2019).
- Saminathan, V. R., Senguttuvan, T., & Gajendran, G. (2011). Combined efficacy of neem and insecticides against brinjal mealy bug, Coccidohystrix insolita (Green). Madras Agricultural Journal, 97(7/9), 273–274.
- Sanguanpong, U & Schmutterer, H. (1992). Laboratory trials on the effects of neem oil and neem-seed based extracts against the TSSM. Tetranychus urticae Agris, 99(6), 637–646.
- SAS (Statistical Analysis System) Institute, Inc. (2002). SAS (R) Proprietary Software Version 9.00 (Ts M0). SAS Institute, Inc.
- Shirin, F., Hossain, M., Kabir, M. F., Roy, M., & Sarker, S. R. (2007). Callus induction and plant regeneration from internodal and leaf explants of four potatoes (Solanum tuberosum L.) cultivars. World Journal of Agricultural Sciences, 3(1), 01–06.
- SPSS (Statistical Package for the Social Sciences). (2007). Registered trade mark software package.
- Stavrinides, M. C., & Mills, N. J. (2009). Demographic effects of pesticides on biological control of Pacific spider mite (Tetranychus pacificus) by the western predatory mite (Galendromus occidentalis). Biological Control, 48(3), 267–273. https://doi.org/10.1016/j.biocontrol.2008.10.017
- Stavrinides, M. C., Van Nieuwenhuyse, P., Van Leeuwen, T., & Mill, N. J. (2010). Development of acaricide resistance in Pacific spider mite (Tetranychus pacificus) from California vineyards. Experimental and Applied Acarology, 50(3), 243–254. https://doi.org/10.1007/s10493-009-9310-y
- Tiruneh, W. G., Chindi, A., & Woldegiorgis, G. (2017). Technical efficiency determinants of potato production: A study of rain-fed and irrigated smallholder farmers in Welmera district, Oromia, Ethiopia. Journal of Development and Agricultural Economics, 9(8), 217–223. https://doi.org/10.5897/JDAE2016.0794
- Tsegaw, T. (2011). Registration of Bubu, a medium maturing improved potato (Solanum tuberosum L.) variety for the Eastern Highlands of Ethiopia. East African Journal of Sciences, 5(1), 69–71.
- Tsolakis, H., Ragusa, E., & Ragusa, S. (2002). Effects of neem oil (Azadirachta indica) on Tetranychus urticae Koch (Acariformes, Tetranchidae) in laboratory tests. Acarid Phylogeny and Evolution: Adaptation in Mites and Ticks, 351–362. https://doi.org/10.1007/978-94-017-0611-7_36
- USEPA (United State Environmental Protection Agency). (2012). Biopesticides registration action document-tagetes oil. Document, 4–22. http://www.epa.gov/pesticides/chem_search/reg_actions/registration/decision
- Venzon, M., Rosado, M. C., Molina-Rugama, A. J., Duarte, V. S., Dias, R., & Pallini, A. (2008). Acaricidal efficacy of neem against Polyphago tarsonemuslatus (Banks) (Acari Tarsonemidae). Crop Protection, 27(3–5), 869–872. https://doi.org/10.1016/j.cropro.2007.10.001
- Veronez, B., Sato, M. E., & Nicastro, R. L. (2012). Toxicidade de compostos sintéticos e naturais sobre Tetranychus urticae e o predador Phytoseiulus macropilis. Pesquisa https://doi.org/10.1590/S0100-204X2012000400006.
- Wondimu Belay, D., Asfaw, Z., Lulekal, E., & Kassa, B. (2021). Farmers’ management of potato (Solanum tuberosum L.) late blight (Phytophtorainfestans (Mont.) de Bary) and sprouting in Shashemene and West Shewa districts, Ethiopia. Cogent Food & Agriculture, 7(1), 1925432. https://doi.org/10.1080/23311932.2021.1925432