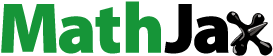
Abstract
Anticancer potential and a prebiotic effect of fructan mixtures from Agave salmiana (branched) and chicory inulin (linear) were evaluated to different mass fractions 1/0, 0/1, 0.5/0.5, 0.75/0.25, 0.25/0.75, respectively, in three concentrations: 2, 3.5, and 5 mg/mL. The viability, proliferation, and apoptosis of human colon cell lines, CRL1831 (healthy), HT29 (cancerous grade 1–2), and SW480 (cancerous grade 3–4), were evaluated by absorbance assays. Lactobacillus acidophilus and Bifidobacterium longum subsp. infantis growth was determined by spectrophotometry at 600 nm. The lactic acid and short-chain fatty acids (SCFAs) production was determined by the HPLC method. Fructan mixtures (inulin/agave, 0.25/0.75) showed a synergic effect on viability, proliferation, and decreased apoptosis in healthy colon cells (CRL1831) at 5 mg/mL. An increase (p < 0.05) of apoptosis in HT29 and SW480 was observed with inulin/agave (0/1) at 3.5 mg/mL and inulin/agave (0.5/0.5), respectively. The proliferation of L. acidophilus and B. longum subsp. infantis was better when the agave fructans were in a higher proportion than inulin. Lactic acid was the highest metabolite produced by L. acidophilus and acetic acid by B. longum subsp. infantis. Fructan mixtures induced SW480 and HT29 cells to apoptosis without toxic effects on healthy cells and improved bacteria proliferation and metabolite production.
Graphical Abstract
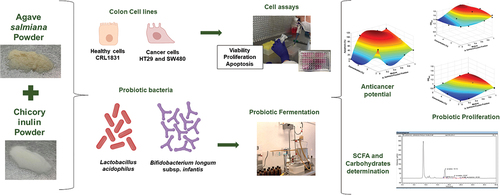
1. Introduction
The gut microbiota plays a vital role in the health and well-being of the host. Furthermore, the health-promoting effects attributed to gut microbiota are mainly related to the production and absorption of nutrients, metabolic homeostasis, and the maintenance of the immune system (Yang et al., Citation2021). However, the imbalance of gut microbiota by reducing diversity, the growth of a group of bacteria, or the increase of proteolytic bacteria leads to dysbiosis (Weiss & Hennet, Citation2017). Many factors, such as medications, surgery, infections, poor nutrition, aging, and lifestyle can cause intestinal dysbiosis. Also, dysbiosis has been connected to developing chronic diseases, including colon cancer (Ambalam et al., Citation2016). Colorectal cancer occupies the second place in causes of death (935, 000) and third place in new cases (1.93 million) found in 2020 (World Health Organization cancer, Citation2021). Colorectal cancer development has been associated with lifestyle and diet (Pandurangan et al., Citation2018). One of the most used strategies to improve host wellness is using prebiotic ingredients to maintain gut microbiota homeostasis. A prebiotic is a substrate selectively utilized by host microorganisms to confer a health benefit (Gibson et al., Citation2017). Several studies have demonstrated that linear (β (2→1)) and branched (β (2→1) and β (2→6)) fructans from chicory and agave plants, respectively, have prebiotic effects (Castillo Andrade et al., Citation2018, Citation2019; Moreno Vilet et al., Citation2014). In vivo, these fructans increase the proliferation of beneficial gut microbiota bacteria, producing short-chain fatty acids (SCFAs). These latter metabolites help to improve the barrier function of the intestinal epithelium and decrease luminal pH, which inhibits pathogenic bacteria, protonates ammonia to ammonium ion, limiting its diffusion to the blood and increasing its fecal excretion and inhibit the transformation of primary bile salts into secondary, which are considered a risk factor for the development of colon cancer (Peredo Lovillo et al., Citation2020). Studies, however, of the synergic effect through the combination of different sources of prebiotics are scarce. It is then interesting to identify ingredients that improve gut microbiota health, reducing colon cancer risks. Therefore, this study aimed to evaluate the synergic effect in vitro of linear and branched fructan mixtures to improve probiotic proliferation, SFCA production, and anticancer potential in human colon cells.
2. Material and methods
The attainment and characterization of Agave salmiana fructans were previously published by Citation2018), whereas chicory inulin-type fructan (Orafti®HIS, 88% inulin) was donated by Beneo (Belgium). Both linear and branched fructans had a PD of 10 to 30 to eliminate this variable from this study.
2.1. Preparation of fructan mixtures
A completely randomized D-optimal experimental design with two mixture variables (linear and branched fructan mixtures) and two process variables for cell assays (fructan concentration and cell type) and probiotic proliferation (fructan concentration and bacteria type) were attained using MODDE 7.0 software (Umetric Inc.). A total of 38 and 30 experiments were performed for cell assays and probiotic proliferation, respectively (Tables ). The preparation of fructan mixtures was performed as follows: solutions were first prepared in concentrations of 2, 3.5 y 5 mg/mL with the mass fractions corresponding to inulin (linear fructans) and A. salmiana fructans (branched fructans): 1/0, 0/1, 0.5/0.5, 0.75/0.25, 0.25/0.75, respectively. The fraction sum must then be equal to 1. For cell-based assays, mixtures were dissolved in a medium supplemented with RPMI-1640 with 10% fetal bovine serum (FBS) and 1% antibiotics (100 U/mL penicillin, 100 µg/mL streptomycin and 0.25 µg/mL amphotericin). Regarding probiotic fermentation, mixtures were dissolved in distilled water at 40% (w/v) and sterilized (121°C/15 min).
Table 1. D-optimal experimental design for fructan mixtures on anticancer potential
Table 2. D-optimal experimental design for fructan mixtures on probiotic proliferation and response variables
2.2. Anticancer activity in human colon epithelial cells
The human colon epithelial cell lines CRL1831(ATCC CRL-1831) (healthy), HT29 (ATCC HTB-38) (cancerous, grade 1–2), and SW480 (ATCC CCL-228) (cancerous, grade 3–4) were used to evaluate anticancer activity. Each colon cell line was supplemented with fructan mixtures (200 µL) in 96-well plates (1 × 104 cells/well) and immediately incubated (at 37°C, 5% CO2) for 24, 72, and 120 h. The viability assay was performed using 20 µL of Alamar Blue (resazurin) assay, and the absorbance was measured with a spectrophotometer Thermo Scientific at 570 nm. Finally, the proliferation and apoptosis assay were performed using the ApoLive-Glo™ Multiplex Assay according to the manufacturer’s instructions. The absorbance was measured at 450 and 620 nm, respectively.
2.3. Microscopic analysis
The staining of treated cancer cell lines and examination by fluorescence microscopy are suitable methods for evaluating morphological changes and the expression of cytotoxicity in the cell membrane and chromatin. Because of the experiments’ number, only the cells treated with inulin/agave mixtures (0.5/0.5) at 5 mg/mL were analyzed by immunocytochemistry assay for 0 and 120 h. The DAPI (4,6-diamidino-2-phenylindole) staining method was used to observe visual symptoms of apoptosis in treated cells. Morphological changes from apoptosis were detected by fluorescence microscopy (The Bio-Rad ZOE Fluorescent Cell Imager) after staining with 1 μg/mL DAPI PureBlu DAPI nuclear staining Dye #1351303 (Biorad).
For cell proliferation, BrdU (5’-bromo-2’-deoxyuridine) was incorporated into the DNA of those cells that are in the S phase of the cell cycle. The detection of BrdU was performed through an indirect immunocytochemical technique. For this purpose, cells were incubated with an anti-BrdU mouse Anti-BrdU antibody, clone Bu20a (MCA2483) (Biorad) as the first antibody, and then the images were taken in ZOE Fluorescent Cell Imager (Biorad). The cells were counted under the microscope for both assays, and all experiments were repeated three times.
2.4. Probiotic proliferation
Bacteria proliferation was carried out as described by Martinez Gutierrez et al. (Citation2017) with modifications. Carbohydrate-free MRS medium was sterilized, filled anoxically in an N2–CO2 mixture, and stirred constantly. The medium was distributed in 50 mL amber jars, and then fructan mixtures were added in different concentrations. One mL of 0.5 MacFarland standard bacterial inoculum was used for each Lactobacillus acidophilus strain (DSM 20,079) and Bifidobacterium longum subsp. infantis (DSM 20,088) strain, to then remain in constant agitation (75 rpm, 37°C). Furthermore, 2 mL sample was taken every 3 h for 24 h, with the control being the sample at 0 h. The absorbance was determined at 600 nm using a Thermo Scientific spectrophotometer.
2.5. Short-chain fatty acid determination (SCFAs)
The SCFAs were only determined in the samples with the optimum mass fraction of fructan mixtures (inulin/agave; 0.25/0.75) at 2, 3.5, and 5 mg/mL concentrations for 0 and 21 h (the log phase’s highest point). The methodology described by Citation2012) with some modifications was used in this study. The bacterial samples were centrifuged and stored at −80°C until further use. Moreover, 20 µL were introduced in an HPLC (Thermo Fisher Scientific UltiMate 3000 and a BioRad Aminex HPX-87 H column) at 30°C with a 0.008 N H2SO4 as the mobile phase 100 A, in isocratic mode, at a flow rate of 0.4 mL/min, with an IR detector, and at a run time of 40 min.
3. Statistical analysis
Two polynomial equations (EquationEqs. 1(1)
(1) and Equation2
(2)
(2) ) were used to evaluate the effect of fructan mixtures of inulin and A. salmiana fructans on cell assays and probiotic proliferation, respectively:
Y represents viability, proliferation, and apoptosis using EquationEq. 1(1)
(1) and Bifidobacterium longum subsp. infantis or Lactobacillus acidophilus proliferation using EquationEq. 2
(2)
(2) . Parameters α1-α28 of both equations are the coefficients from the multivariate regression model for the four-way analysis of variance (ANOVA), which includes a cubic model for the mixing variables (A and B) and a quadratic model for the process variables (C and D). The branched fructans are (A), the linear fructans are (B), the concentration is (C), and the cell or bacteria type is (D). A confidence level of 95% (p < 0.05) with MODDE 7.0 statistical software (Umetric AB) was used. EquationEqs. 1
(1)
(1) and Equation2
(2)
(2) were reduced to its corresponding lopped forms after the omission of the statistically nonsignificant coefficient values (p > 0.05), and mathematical models were created, data were sent to MATLAB 13 files to draw the required plots. For SCFAs, a Tukey test was used to analyze whether significant variation existed between the treatments at probability values of p < 0.05. The experiments of cell assays were performed in triplicate and for probiotic proliferation and SCFAs in duplicate.
4. Results and discussion
4.1. Anticancer activity in human colon epithelial cell
Cell cytotoxic (viability), proliferation, and apoptosis were measured to evaluate the anticancer potential of fructan mixtures.Table shows the data obtained for each experiment performed. The parameters evaluated had a significant effect (P = 2.45E–06 and R2 = 0.99) on the viability of the three cell types at 72 h. Figures show the cell viability results of CRL1831, HT29 and SW480 respectively. The viability of CRL1831 cells was increased with fructan mixture treatments. The highest rate (around 100%) (Figure ) was observed at a 0.25 mass fraction of inulin and 0.75 of A. salmiana fructans with a concentration of 3.5 mg/mL, which was significantly higher than the control and the cancer cells (p < 0.05). These mixtures had no cytotoxic effect on the cellular viability of CRL1831 because all treatments maintained cellular balance. Simultaneously, the cell viability of HT29 (Figure ) and SW480 (Figure ) decreased significantly (p < 0.05), with 0.75 of inulin and 0.25 of A. salmiana fructans being linear fructan, the predominant component with concentrations around 2 mg/mL. SW480 (Figure ) had the lowest viability percentages (around 10%) as compared with HT29 (around 15%) (Figure ). These results agree with other studies where SW480 cells tended to be more sensitive to some treatments (Sun et al., Citation2015). This might be because cancer cells tend to change their structure, making them less resistant than healthy cells (Alibert et al., Citation2017; Letai, Citation2017). In general, the healthy cells (Figure ) showed better viability percentages compared to HT29 (Figure ) and SW480 (Figure ). This indicates that fructan mixtures benefit healthy colon cells and help control colon cancer cells’ viability since no cytotoxicity was found in healthy cells compared with cancer cells, which is a prerequisite for any chemopreventive agent.
The treatments also significantly affected cell proliferation (P = 0.0003 and R2 0.96). Figures show the cell proliferation results of CRL1831, HT29 and SW480, respectively. At 120 h, inulin/agave mixtures (0.5/0.5) provided the highest proliferation percentages (around 100%) for CRL1831 cells at concentrations of 5 mg/mL (Figure ). It was observed that when only inulin was present, the proliferation of healthy cells was low and remained constant for any time and concentration.
At the same time, for HT29 (Figure ), the proliferation percentage was the lowest (p < 0.05) with inulin (1/0) at 2 mg/mL of concentration. In the case of SW480 cells (Figure ), the lowest proliferation percentage (around 0%) was also observed with inulin (1/0) at any concentration. The increase in the antiproliferative effect in HT29 and SW480 cells treated with fructans is directly associated with fructan type, mixture fraction, and dosage (fraction mixtures and fructans’ concentration). The SW480 cells showed less resistance to the antiproliferative effect of fructan mixtures than HT29 cells, consistent with previous results where these same cell lines were used. These authors suggested that the stage, grade, and disease progression are relevant factors affecting their proliferation process because of the differences between their TGF-β pathways’ regulation (Agarwal et al., Citation1999; Nowakowska et al., Citation2014). Similar results were reported by Le et al. (Citation2019); when prebiotics were added to probiotic cultures, a proliferation inhibition of colon cancer cells (HCT116, Caco-2) was observed. However, when agave fructans were present in the mixtures, an opposite effect was observed, indicating a higher proliferation percentage of cancer cells. Figures show the images of immunocytochemical assays of fructan mixtures (0.5/0.5) at 5 mg/mL for the three cell types. In these conditions, these fructans help maintain the proliferation activity of healthy cells (Figure ) and promote cancer cell proliferation (Figure ). Recall that BrdU is incorporated in the synthesis phase, meaning the mixture is an excellent chemopreventive for healthy cells. Perhaps branched fructan allows cells to generate energy to increase their growth and proliferation since it was reported that a higher rate of glucose metabolism would enable cells to produce abundant ATP and the evasion of apoptosis pathways (DeBerardinis et al., Citation2008; Fritz & Fajas, Citation2010; Leal Esteban & Fajas, Citation2020).
Figure 3. Cells proliferation by BrdU incorporation a) CRL1831 cells control, at 0 h, b) CRL1831 cells, with 5 mg/mL of 0.5/0.5 agave/inulin at 120 h, c) HT29 cells control, at 0 h, d) HT29 cells with 5 mg/mL of 0.5/0.5 agave/inulin at 120 h, e) SW480 control cells, at 0 h, f) SW480 cells with 5 mg/mL of 0.5/0.5 agave/inulin at 120 h.
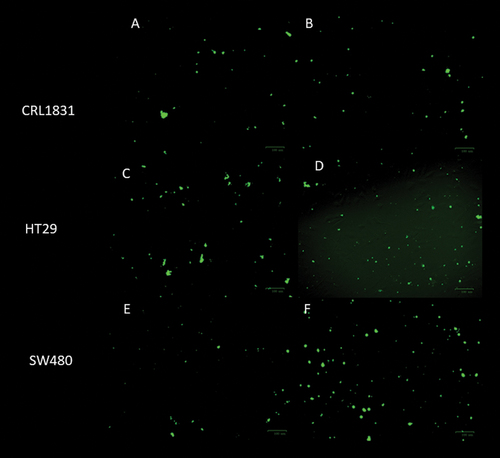
Concerning apoptosis has been defined as a tightly controlled programmed cell death that helps to contribute to the elimination of unwanted cells to retain the balance between the survival and death of cells (Arun et al., Citation2019). When cell death is stopped, malignant cells are generated, which are more resistant to apoptotic pathways. In this sense, the search for new strategies or ingredients that induce apoptosis is necessary. In this study, apoptosis was significantly affected (P = 6.32E–06 R2 = 0.98) by the parameters evaluated at 120 h. Figures show the results at 120 h for the three types of line cells used. CRL1831 cells (Figure ) had the lowest percentages (around 0%) of apoptotic cells in comparison to cancer cells, and inulin/agave (0.25/0.75) mixtures gave the best results, where the predominant component was branched fructan at 2 mg/mL of concentration. Fructans were able to increase the apoptotic cells both in the HT29 and SW480 cell lines. Specifically for HT29 cells (Figure ), apoptotic cells significantly increased (p < 0.05) with branched fructan (0/1) as the predominant component at a concentration of around 3.5 mg/mL. Conversely, for SW480 cells (Figure ), the percentages of apoptotic cells were significantly high (p < 0.05) with inulin/agave mixtures (0.5/0.5) at a concentration of 5 mg/mL. In general, SW480 cells showed a stronger tendency towards apoptosis than HT29; this agrees with other studies (Agarwal et al., Citation1999), where apoptosis resistance was higher in HT29 cells. The same was also observed for DAPI staining at 120 h. DAPI (4′, 6-diamidino-2-phenylindole) is a blue fluorescent DNA stain that exhibits~20-fold enhancement of fluorescence upon binding to dsDNA. It is excited by the violet (405 nm) laser line and is commonly used as chromosome staining (Figure ).
Figure 5. Detection of apoptotic cells by DAPI staining, a) CRL1831 cells control, at 0 h, b) CRL1831 cells, with 5 mg/mL of 0.5/0.5 agave/inulin at 120 h, c) HT29 cells control, at 0 h, d) HT29 cells with 5 mg/mL of 0.5/0.5 agave/inulin at 120 h, e) SW480 control cells, at 0 h, f) SW480 cells with 5 mg/mL of 0.5/0.5 agave/inulin at 120 h.
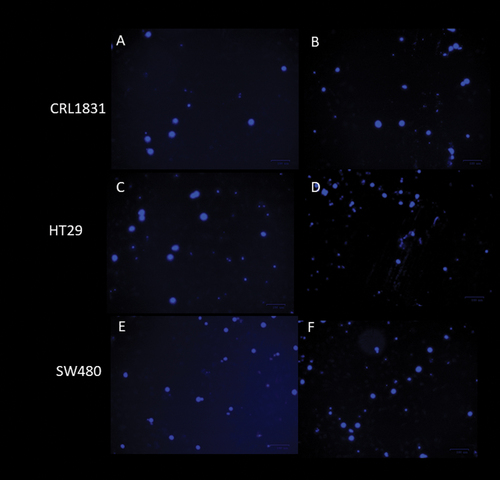
The literature also states that apoptosis increases with the histologic grade of cancer (Wang et al., Citation2014). The results from this study agree with this statement since SW480 is a grade 3–4 type of cancer, while HT29 is a grade 1–2 (Leibovltz et al., Citation1976). These results are interesting because the combination of linear and branched fructans can prevent the apoptosis of healthy colon cells and induce the apoptosis of colon cancer cells. These fructans could act as nutrients preventing the formation of reactive oxygen in healthy cells and as suppressors or inhibitors of cancer cells because of the high content of β-(2→1 and 2→6) O-glycosidic bonds as well as its molecular structure (Malyarenko et al., Citation2017). To our knowledge, this is the first study that has used crude fructan mixtures from inulin and A. salmiana to evaluate the anticancer potential in vitro because most studies (Arun et al., Citation2019; Borowicki et al., Citation2010) have added prebiotics to probiotic bacteria culture, and the fermented supernatant then was used to evaluate the anticancer effect. These studies showed that butyrate is the primary SCFA that can induce apoptosis and inhibit cell proliferation by arresting the cell cycle in the G0/G1 phase (Borowicki et al., Citation2010) and induce apoptosis of HT29 cells through DNA condensation and fragmentation, as well as through reactive oxygen species (ROS) production (Arun et al., Citation2019). Therefore, we cannot say that butyrate is responsible for inducing apoptosis because we used crude fructans. Our results agree with those reported by Citation2018), who employed crude polysaccharides from mushrooms C. cibarius to evaluate anticancer properties. Their results showed anticancer and anti-inflammatory potential in the human colon adenocarcinoma cell line LS180 through an MTT assay and by inhibiting the activity of two isoforms of cyclooxygenase COX-1 and COX-2, respectively. These fructans probably led to an inhibitory effect on these enzymes, associated with cancer progression by inhibiting apoptosis. Further studies are necessary to corroborate these enzymatic activities.
4.2. Probiotic proliferation
According to bacterial kinetics obtained with the different treatments, the highest OD600 values were obtained at 21 h for Lactobacillus acidophilus and Bifidobacterium longum subsp. infantis and were statistically significant (P = 7.08E–09, R2 0.99). Lactobacillus acidophilus (Figure ) had the best proliferation results, with the lowest and highest optical densities of 1.41 to 1.82, respectively, compared with Bifidobacterium longum subsp. infantis (Figure ) with densities of 0.78 to 1.12, respectively. For Lactobacillus acidophilus (Figure ), the highest OD was obtained with the inulin/agave fraction (0.25/0.75), where the branched fructan was the predominant component with a concentration of around 5 mg/mL. For Bifidobacterium longum subsp. infantis, however, the highest bacteria number was obtained with inulin/agave (0.25/0.75) and (0/1) with a concentration of around 3.5 mg/mL. This indicates that by applying the same conditions (fructan degree of polymerization, temperature, time, fructans’ proportions, and mixture concentrations), fructan mixtures inulin/agave (0.25/0.75) have a synergic effect on probiotic proliferation in both bacteria. In a previous in vitro study (Martinez Gutierrez et al., Citation2017), it was reported that different individual prebiotic sources stimulated Bifidobacterium and Lactobacillus counts. However, the OD of our study was higher than this previous study, which indicates that using fructan mixtures improves the growth of both bacteria. However, they preferred branched fructans, which agrees with the results reported by Castillo Andrade et al. (Citation2019).
Figure 6. Optical density (600 nm) of a) Lactobacillus acidophilus and b) Bifidobacterium longum subsp. infantis at 21 h fermentation.
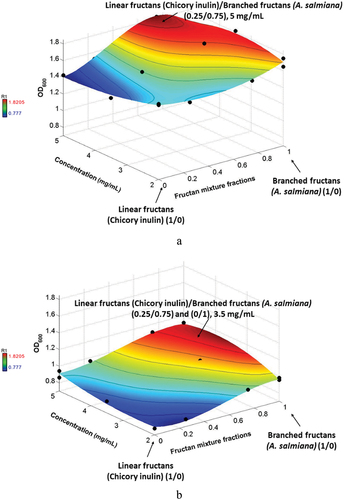
Lactobacillus spp and Bifidobacterium spp are the most commonly known probiotics because of their capacity to regulate proper intestinal function and be effective in the prevention or treatment of several gastrointestinal disorders: (diarrhea, necrotizing enterocolitis, antibiotic-associated diarrhea, inflammatory bowel disease, cancer, and surgical infections, among others) (Gupta & Garg, Citation2009; Linares et al., Citation2017). That is why it is important to improve the proliferation of these bacteria, and fructan mixtures could provide a beneficial effect on the host.
4.3. Short-fatty acid production (SCFAs)
Table shows the results of SCFAs and lactic acid production by the fermentation of Lactobacillus acidophilus and Bifidobacterium longum subsp. infantis and the best production was obtained using inulin/agave mixtures (0.25/0.75). For Lactobacillus acidophilus, the total content of SCFAs increased significantly (p < 0.05) regarding the control in the three concentrations evaluated. The SCFAs’ concentration ranged from 46.4 (0 h) to 54.48 mM (21 h), approximately 1.2 times higher than the control group. No significant differences (p > 0.05) between the three concentrations evaluated were observed. Similar results were obtained by Bifidobacterium longum subsp. infantis fermentation (Table ), and the total content of SCFAs increased significantly (p < 0.05) in the three concentrations evaluated: from 43.97 to 64.0. SFCA values of this study were higher than the results reported by Citation2017) using A. salmiana fructans, chicory inulin, and glucose as individual prebiotics in the growth of different strains of Lactobacillus and Bifidobacterium. Lactic acid production by L. acidophilus was the highest (p < 0.05) with 3.5 mg/mL of substrate concentration (Table ), and this was higher than those produced by B. longum subsp. infantis, which corroborates that lactic acid is a specific metabolite of Lactobacillus spp. In general, the lactic acid production of both bacteria was directly proportional (p < 0.05) to the substrate concentration. Similar behavior was observed by Citation2017) for the Lactobacillus and Bifidobacterium species; conversely, the values of this study were higher than those reported by these authors. The differences observed could be attributed to the substrate concentration that was used. It was reported by Vulevic et al. (Citation2004) that the prebiotic effect is associated with lactic acid by producing bacteria like Lactobacillus and Bifidobacterium species. Therefore, the ratio of lactic acid production over the total SCFA production can be calculated to provide a qualitative and quantitative assessment of each substrate tested. For L. acidophilus, the ratio of acid lactic production was 0.98, which was similar in the three evaluated concentrations. For B. longum subsp. infantis, however, the highest ratio of 0.87 was produced in 5% of the substrate, which is a higher ratio, that suggests that these substrates better supported the growth of lactic acid-producing bacteria. These values were higher than those reported by Vulevic et al. (Citation2004), and the differences observed could be attributed to concentration and substrate type as well as the strain used. Acetic and propionic acid were produced in higher (p < 0.05) concentrations by B. longum subsp. infantis than by L. acidophilus, which is in agreement with the results reported by Martinez Gutierrez et al. (Citation2017). These values, however, were higher than those reported by these authors. Propionic acid was produced in smaller amounts than lactic and acetic acid by both bacteria, but its concentration was enhanced using fructan mixtures, with regard to previous studies reported (Martinez Gutierrez et al., Citation2017; Vulevic et al., Citation2004). In addition, this study confirms that the metabolites’ production was dependent on the bacteria genus used, as previously reported by these last authors, but this finding supports the ability of fructan mixtures to enhance the production of lactic, acetic, and propionic acid.
Table 3. Concentration of SCFA from fermentation of inulina and A. salmiana fructans mixtures (mM)
These organic acids can lower luminal pH, inhibit pathogen growth, improve the maintenance of epithelial barrier function, increase calcium solubility, facilitate the passive uptake and induce select tight junction proteins and increase the growth of beneficial bacteria that can inhibit the conversion of pro-carcinogens into carcinogens: nitroreductase, β-glucuronidase, and β-glucosidase inactivation (Koh et al., Citation2016; Peredo Lovillo et al., Citation2020; Ranjbar et al., Citation2021; Sanders et al., Citation2019).
Acetate is produced from pyruvate via acetyl-CoA and the Wood-Ljungdahl pathway and is used as an energy source by the cells (Koh et al., Citation2016). Propionate can be formed from phosphoenolpyruvate through the succinate or acrylate pathways, in which lactate is reduced to propionate. Lactate and acetate are starting units used by other colon bacteria through cross-feeding for butyrate, which promotes colon motility, reduces inflammation, induces apoptosis of cancerous colonocytes, and inhibits cell progression as well as propionate production. They can bind and eliminate carcinogens from the intestinal system. Propionate reduces lipogenesis and cholesterol synthesis, controls intestinal inflammation (histone deacetylase), and exerts crucial physiological effects on several organs. Like acetate, this metabolite reduces diabetes by boosting colonic regulatory T (Treg) cells’ function. Lactate improves gut healthy and immune defense and increases the absorption area in the intestinal barrier (Allsopp et al., Citation2013; Koh et al., Citation2016; Peredo Lovillo et al., Citation2020; Ranjbar et al., Citation2021; Sanders et al., Citation2019; Silva et al., Citation2020). Thus, producing SCFAs by microorganisms is vital for gastrointestinal health in general. This study proves that the mixture of linear and branched prebiotics improves in vitro the production of SCFA.
5. Conclusions
The results of the present study provide evidence for the in vitro anticancer potential of fructan mixtures from chicory inulin and Agave salmiana through decreased proliferation and increased apoptosis induction in colon cancer cells HT29 and SW480. Fructans mixtures benefitted healthy colon CRL1831 cells, finding no cytotoxicity in healthy cells compared with cancer cells, which is a prerequisite for any chemopreventive agent. These fructan mixtures also enhanced the potential prebiotics properties favoring the growth of Lactobacillus acidophilus compared to Bifidobacterium longum subsp. infantis. The metabolism activity of these bacteria was also improved by the increase of lactic acid and short-chain fatty acids’ production. Therefore, mixtures of linear and branched fructans could be an effective strategy for cancer colon prevention and prebiotic potential. Further studies are necessary to conduct other assays that allow elucidating the mechanisms of action of fructan mixtures on colon cancer cells.
Author contributions
P.A.G. completed the analysis, data acquisition, interpretation and drafted the manuscript. L.E.A.Q. made human colon cells analysis and interpretation. M.A.R.C. drafted the manuscript, providing revision. F.M.G. performed microorganism analysis and interpretation. R.G.G. participed with experimental design and statistic analysis. A.G.L. developed the concept and interpretation of the data and drafted the manuscript and intellectual content. All authors approved the final version of the manuscript.
Acknowledgments
This work was supported by Fondo de Apoyo a la Investigación de la Universidad Autónoma de San Luis Potosí, México (C16-FAI-09-09.09). Paola Alvarez-García received a scholarship from CONACYT, MEXICO.
Disclosure statement
No potential conflict of interest was reported by the authors.
Data availability statement
All generated data during this study are included in this published article.
Additional information
Funding
Notes on contributors
Luz Eugenia Alcántara Quintana
Dr. Luz Eugenia Alcántara Quintana She is a CONACyT Chair attached to the Coordination for Innovation and Application of Science and Technology. She is currently working on discovering the molecular patterns that can cause cancer due to exposure to pollutants. As well as in the biochemical and molecular analysis of anticancer substances. Also in novel treatments for the transdermal administration of drugs for chronic and acute pain in pediatric and adult cancer patients.
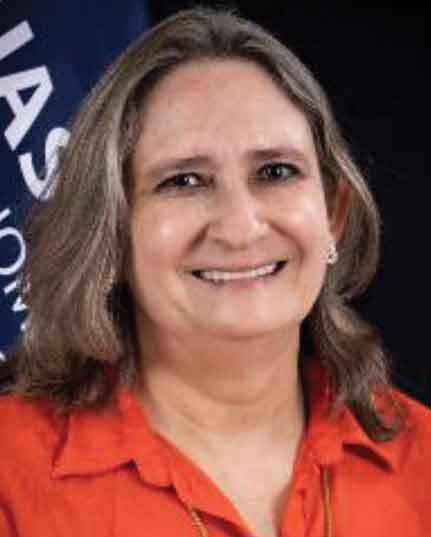
Alicia Grajales Lagunes
Alicia Grajales-Lagunes She is a Researcher Professor at the Faculty of Chemical Science of the Autonomous University of San Luis Potosi, Mexico. She is currently working with prebiotic effect of agave fructans on intestinal health, to use these fructans in the development of functional food that improve the consumers’ health. This study is related to another study where these fructan mixtures will be used to develop functional meat products. Additionally, we have an interdisciplinary group where each collaborator works with different fields like: Food Engineering, thermophysical properties of foods, Process Modeling and Simulation, Design and Analysis of Statistical Experiments, biochemical and molecular analysis of anticancer substances, probiotic microbiology.
References
- Agarwal, B., Bhendwal, S., Halmos, B., Moss, S. F., Ramey, W. G., & Holt, P. R. (1999). Lovastatin augments apoptosis induced by chemotherapeutic agents in colon cancer cells. Clinical Cancer Research, 5(8), 2223–19.
- Alibert, C., Goud, B., & Manneville, J. B. (2017). Are cancer cells really softer than normal cells? Biology of the Cell, 109(5), 167–189. https://doi.org/10.1111/boc.201600078
- Allsopp, P., Possemiers, S., Campbell, D., Saldaña Oyarzábal, I., Gill, C., & Rowland, I. (2013). An exploratory study into the putative prebiotic activity of fructans isolated from Agave angustifolia and the associated anticancer activity. Anaerobe, 22, 38–44. https://doi.org/10.1016/j.anaerobe.2013.05.006
- Ambalam, P., Raman, M., Purama, R. K., & Doble, M. (2016). Probiotics, prebiotics and colorectal cancer prevention. Best Practice & Research Clinical Gastroenterology, 30(1), 119–131. https://doi.org/10.1016/j.bpg.2016.02.009
- Arun, K. B., Madhavan, A., Reshmitha, T. R., Thomas, S., Nisha, P., & Nie, D. (2019). Short chain fatty acids enriched fermentation metabolites of soluble dietary fibre from Musa paradisiaca drives HT29 colon cancer cells to apoptosis. Plos One, 14(5), 1–20. https://doi.org/10.1371/journal.pone.0216604
- Borowicki, A., Stein, K., Scharlau, D., Scheu, K., Brenner Weiss, G., Obst, U., Hollmann, J., Lindhauer, M., Wachter, N., & Glei, M. (2010). Fermented wheat aleurone inhibits growth and induces apoptosis in human HT29 colon adenocarcinoma cells. The British Journal of Nutrition, 103(3), 360–369. https://doi.org/10.1017/S0007114509991899
- Castillo Andrade, A. I., Rivera Bautista, C., Godínez Hernandez, C., Ruiz Cabrera, M. A., Fuentes Ahumada, C., García Chavez, E., & Grajales Lagunes, A. (2018). Physiometabolic effects of Agave salmiana fructans evaluated in Wistar rats. International Journal of Biological Macromolecules, 108, 1300–1309. https://doi.org/10.1016/j.ijbiomac.2017.11.043
- Castillo Andrade, A. I., Rivera Bautista, C., Ruiz Cabrera, M. A., Soria Guerra, R. E., Fuentes Ahumada, C., García Chavez, E., & Grajales Lagunes, A. (2019). Agave salmiana fructans as gut health promoters: Prebiotic activity and inflammatory response in Wistar healthy rats. International Journal of Biological Macromolecules, 136, 785–795. https://doi.org/10.1016/j.ijbiomac.2019.06.045
- DeBerardinis, R. J., Lum, J. J., Hatzivassiliou, G., & Thompson, C. B. (2008). The biology of cancer: Metabolic reprogramming fuels cell growth and proliferation. Cell Metabolism, 7(1), 11–20. https://doi.org/10.1016/j.cmet.2007.10.002
- Fritz, V., & Fajas, L. (2010). Metabolism and proliferation share common regulatory pathways in cancer cells. Oncogene, 29(31), 4369–4377. https://doi.org/10.1038/onc.2010.182
- Gibson, G. R., Hutkins, R., Sanders, M. E., Prescott, S. L., Reimer, R. A., Salminen, S. J., Scott, K., Stanton, C., Swanson, K. S., Cani, P. D., Verbeke, K., & Reid, G. (2017). The International Scientific Association for Probiotics and Prebiotics (ISAPP) consensus statement on the definition and scope of prebiotics. Nature Reviews Gastroenterology & Hepatology, 14(8), 491–502. https://doi.org/10.1038/nrgastro.2017.75
- Gupta, V., & Garg, R. (2009). Probiotics. Indian Journal of Medical Microbiology, 27(3), 202–209. https://doi.org/10.4103/0255-0857.53201
- Hernandez Hernandez, O., Muthaiyan, A., Moreno, F. J., Montilla, A., Sanz, M. L., & Ricke, S. C. (2012). Effect of prebiotic carbohydrates on the growth and tolerance of Lactobacillus. Food Microbiology, 30(2), 355–361. https://doi.org/10.1016/j.fm.2011.12.022
- Koh, A., De Vadder, F., Kovatcheva Datchary, P., & Bäckhed, F. (2016). From dietary fiber to host physiology: Short-chain fatty acids as key bacterial metabolites. Cell, 165(6), 1332–1345. https://doi.org/10.1016/j.cell.2016.05.041
- Leal Esteban, L. C., & Fajas, L. (2020). Cell cycle regulators in cancer cell metabolism. Biochimica Et Biophysica Acta Molecular Basis of Disease, 1866(5), 165715. https://doi.org/10.1016/j.bbadis.2020.165715
- Leibovltz, A., Stlnson, J. C., McCombs, W. B., McCoy, C. E., Mazur, K. C., & Mabry, N. D. (1976). Classification of human colorectal adenocarcinoma Cell Lines. Cancer Research, 36(12), 4562–4569.
- Le, B., Ngoc, A. P. T., & Yang, S. H. (2019). Synbiotic fermented soymilk with Weissella cibaria FB069 and xylooligosaccharides prevents proliferation in human colon cancer cells. Journal Applied Microbiology, 128(5), 1486–1496. https://doi.org/10.1111/jam.14551
- Letai, A. (2017). Apoptosis and cancer. Annual Review of Cancer Biology, 1(1), 275–294. https://doi.org/10.1146/annurev-cancerbio-050216-121933
- Linares, D. M., Gómez, C., Renes, E., Fresno, J. M., Tornadijo, M. E., Ross, R. P., & Stanton, C. (2017). Lactic acid bacteria and bifidobacteria with potential to design natural biofunctional health-promoting dairy foods. Frontiers in Microbiology, 8, 846. https://doi.org/10.3389/fmicb.2017.00846
- Malyarenko, O. S., Usoltseva, R. V., Shevchenko, N. M., Isakov, V. V., Zvyagintseva, T. N., & Ermakova, S. P. (2017). In vitro anticancer activity of the laminarans from Far Eastern brown seaweeds and their sulfated derivatives. Journal of Applied Phycology, 29(1), 543–553. https://doi.org/10.1007/s10811-016-0915-3
- Martinez Gutierrez, F., Ratering, S., Juárez Flores, B., Godinez Hernandez, C., Geissler Plaum, R., Prell, F., Zorn, H., Czermak, P., & Schnell, S. (2017). Potential use of Agave salmiana as a prebiotic that stimulates the growth of probiotic bacteria. LWT Food Science and Technology, 87, 151–159. https://doi.org/10.1016/j.lwt.2017.05.044
- Moreno Vilet, L., Garcia Hernandez, M. H., Delgado Portales, R. E., Corral Fernández, N. E., Cortez Espinosa, N., Ruiz Cabrera, M. A., & Portales Perez, D. P. (2014). In vitro assessment of agave fructans (Agave salmiana) as prebiotics and immune system activators. International Journal of Biological Macromolecules, 63, 181–187. https://doi.org/10.1016/j.ijbiomac.2013.10.039
- Nowacka-Jechalkea, N., Nowaka, R., Judab, M., Malmb, A., Lemieszekc, M., Rzeskic, W., & Kaczyński, Z. (2018). New biological activity of the polysaccharide fraction from cantharellus cibarius and its structural characterization. Food Chemistry, 268, 355–361. https://doi.org/10.1016/j.foodchem.2018.06.106
- Nowakowska, M., Pospiech, K., Lewandowska, U., Piastowska Ciesielska, A. W., & Bednarek, A. K. (2014). Diverse effect of WWOX overexpression in HT29 and SW480 colon cancer cell lines. Tumor Biology, 35(9), 9291–9301. https://doi.org/10.1007/s13277-014-2196-2
- Pandurangan, A. K., Divya, T., Kumar, K., Dineshbabu, V., Bakthavatchalam, V., & Sudhandiran, G. (2018). Colorectal carcinogenesis: Insights into the cell death and signal transduction pathways: A review. World Journal of Gastrointestinal Oncology, 10(9), 244–259. https://doi.org/10.4251/wjgo.v10.i9.244
- Peredo Lovillo, A., Romero Luna, H. E., & Jiménez Fernández, M. (2020). Health promoting microbial metabolites produced by gut microbiota after prebiotics metabolism. Food Research International, 136, 109473. https://doi.org/10.1016/j.foodres.2020.109473
- Ranjbar, R., Vahdati, S. N., Tavakoli, S., Khodaie, R., & Behboudi, H. (2021). Immunomodulatory roles of microbiota-derived short-chain fatty acids in bacterial infections. Biomedicine & Pharmacotherapy, 141, 111817. https://doi.org/10.1016/j.biopha.2021.111817
- Sanders, M. E., Merenstein, D. J., Reid, G., Gibson, G. R., & Rastall, R. A. (2019). Probiotics and prebiotics in intestinal health and disease: From biology to the clinic. Nature Reviews Gastroenterology & Hepatology, 10(10), 605–616. https://doi.org/10.1038/s41575-019-0173-3
- Silva, Y. P., Bernardi, A., & Frozza, R. L. (2020). The role of short-chain fatty acids from gut microbiota in gut-brain communication. Frontiers in Endocrinology, 11, 1–14. https://doi.org/10.3389/fendo.2020.00025
- Sun, Y., Liu, Z., Zou, X., Lan, Y., Sun, X., Wang, X., Zhao, S., Jiang, C., & Liu, H. (2015). Mechanisms underlying 3-bromopyruvate-induced cell death in colon cancer. Journal of Bioenergetic and Biomembranes, 47(4), 319–329. https://doi.org/10.1007/s10863-015-9612-1
- Vulevic, J., Rastall, R. A., & Gibson, G. R. (2004). Developing quantitative approach for determining the in vitro prebiotic potential of dietary oligosaccharides. FEMS Microbiology Letters, 236(1), 153–159. https://doi.org/10.1111/j.1574-6968.2004.tb09641.x
- Wang, R. A., Li, Z. S., Yan, Q. G., Bian, X. W., Ding, Y. Q., Du, X., Sun, B. C., Sun, Y. T., & Zhang, X. H. (2014). Resistance to apoptosis should not be taken as a hallmark of cancer. Chinese Journal of Cancer, 33(2), 47–50. https://doi.org/10.5732/cjc.013.10131
- Weiss, G. A., & Hennet, T. (2017). Mechanisms and consequences of intestinal dysbiosis. Cellular and Molecules Life Sciences, 74(16), 2959–2977. https://doi.org/10.1007/s00018-017-2509-x
- World Health Organization cancer. (2021). https://www.who.int/news-room/fact-sheets/detail/cancer.
- Yang, W., Liu, Y., Yang, G., Meng, B., Yi, Z., Yang, G., Chen, M., Hou, P., Wang, H., & Xu, X. (2021). Moderate-intensity physical exercise affects the exercise performance and gut microbiota of mice. Frontiers in Cellular and Infection Microbiology, 11, 712381. https://doi.org/10.3389/fcimb.2021.712381