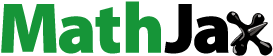
Abstract
The main objective in this study was to evaluate full-fat Stinkbug as an alternative protein source in the diet of Oreochromis mossambicus. Five diets were formulated to partially replace fishmeal at 0, 10, 30, 50, and 70% levels and were denoted as D1, D2, D3, D4, and D5, respectively. The diets were formulated to be isonitrogenous, isocaloric, and isolipidic. Each diet was fed to triplicate groups of fish for 8 weeks. All growth performance indices declined with an increase in Stinkbug meal inclusion. The specific growth rate declined from 2.16% in the control to 1.28% in D5 (P < 0.05). The thermal-unit growth coefficient declined from 1.30 in the control to 0.72 in diet D5 (P < 0.05). The protein efficiency ratio declined from 2.95 in the control to 1.66 in D5 (P < 0.05). However, organosomatic indices did not differ across the fish diets. The aspartate aminotransferase (AST) and alanine aminotransferase (ALT) activities did not differ significantly (P > 0.05) across diets D1 to D4. No histological alterations were evident in the liver and intestine across diets. It was therefore concluded that Stinkbug meal may partially replace fishmeal at 30% replacement level in the diet of O. mossambicus without adverse effects on growth performance and nutrient utilisation indices.
1. Introduction
The use of insect meals in aquafeed has recently become a research priority in the aquaculture sector (Abdel-Tawwab et al., Citation2020; Llagostera et al., Citation2019). This is mainly due to their nutritional composition and high feed conversion efficiency (Bruni et al., Citation2018; Fawole et al., Citation2020). Insects form part of the natural diet of both marine and freshwater fish species (Makkar et al., Citation2014; van Huis, Citation2013) and different orders exhibit different nutritional compositions (Hua, Citation2021). The insect meal protein content ranges between 50 and 82% on a dry matter basis, whilst the fat content ranges from 10 to 30% (Henry et al., Citation2015; Rumpold & Schlüter, Citation2013). The amino acid profile and the fatty acid composition of insect meal are highly dependent on the insect diet, larval stage, and size (Alfiko et al., Citation2022). Insect meals are also rich in minerals and vitamins (Terova et al., Citation2021). Insects that have been used in aquafeed include black soldier fly larvae (Hermetia illucens) (Fischer et al., Citation2022; Guerreiro et al., Citation2020; Mohan et al., Citation2022), mealworm larvae (Tenebrio molitor) (Coutinho et al., Citation2021; Jeong et al., Citation2020; Tran et al., Citation2022), housefly larvae (Musca domestica) (Akinwole et al., Citation2020; Gbai et al., Citation2019; Saleh, Citation2020), mopane worm (Imbrasia belina) (Nyuliwe et al., Citation2022; Rapatsa & Moyo, Citation2017, Citation2019), and superworm (Zophobas morio) (Henry et al., Citation2022; Jabir et al., Citation2012; Prachom et al., Citation2021). These insects have been used in different fish species (marine and freshwater) with varying degrees of success. In a recent review, Rapatsa and Moyo (Citation2022) recommended the use of locally available insects in the replacement of fishmeal in the diets of O. mossambicus.
Some of these insects have been tested on Tilapia sp. such as O. mossambicus and Oreochromis niloticus. Rapatsa and Moyo (Citation2017) showed that substituting fishmeal with I. belina meal increased the growth performance of O. mossambicus with an increase in I. belina meal inclusion. Additionally, studies showed that H. illucens can completely replace fishmeal in the diets of O. niloticus without any adverse effects on growth performance and nutrient utilisation (Kishawy et al., Citation2022; Tippayadara et al., Citation2021). However, Devic et al. (Citation2017) showed that the best growth performance of O. niloticus fed diets with H. illucens was at 30% inclusion level. Furthermore, inclusion levels beyond 30% showed a compromised growth performance and poor nutrient utilisation. The varied results may be due to the processing method of insect meal, the insect larval stage, fish size, and fish species (Tschirner & Simon, Citation2015). The use of insect-based protein sources in aquafeed was prompted by the escalating cost of fishmeal, which is the main protein component in animal feed. Thus, there is a need to investigate the potential use of locally available insects in aquafeed.
In Southern Africa, one of the insects that can be used to replace fishmeal is the Stinkbug (Encosternum delegorguei). It is widely distributed in subtropical, open wood and bushveld of Southern regions of Zimbabwe and Northern Provinces of South Africa (Makore et al., Citation2015). The Stinkbug is mainly sold at the open market in Thohoyandou Town between May and October. Seasonal availability is one of the challenges associated with the replacement of fishmeal with insects (van Huis, Citation2022). Most insects are available during the rainy season. However, the Stinkbug is one of the insects that is harvested during the dry season, when there are no other insects available. The Stinkbug contains a high fat content (Teffo et al., Citation2007). However, its protein content and amino acid profile are poorly understood. Furthermore, the distribution of Stinkbugs coincides with the distribution of the Mozambique tilapia (Oreochromis mossambicus), which is one of the commonly cultured warmwater species in Southern Africa. This makes Stinkbug an ideal candidate for the replacement of fishmeal in Southern Africa.
The Mozambique tilapia (Oreochromis mossambicus) is one of the most widely cultured warm freshwater species in Southern Africa. It is a species of economic importance and plays a role in the gross domestic product of South Africa. However, its production has not yet reached its full potential. Oreochromis mossambicus is a good aquaculture candidate species (El-Sayed, Citation2020). Its capacity to utilize insect meals was recently reviewed and showed that it is pre-adapted to utilise insect-based diets (Rapatsa & Moyo, Citation2022). It is therefore imperative to carry out a detailed study on the effect of dietary full-fat Stinkbug meal as a fishmeal replacement on some aspects of the physiology of O. mossambicus. Therefore, the objective of this study was to determine the effect of dietary full-fat Stinkbug meal on growth performance, blood serum chemistry, liver and intestine histology of juvenile O. mossambicus.
2. Materials and methods
2.1. Proximate composition
Stinkbugs were purchased from Thohoyandou Town open market, in the Vhembe District of Limpopo Province, South Africa. Stinkbugs (dried) were ground using a miller. Stinkbug meal was analysed for proximate and nutrient composition (Table ).
Table 1. Proximate composition, mineral content, and amino acid profile of the Stinkbug meal compared to fishmeal
Dry matter was determined by drying samples using an oven at 105°C until a constant weight was reached. Moisture content was determined by obtaining the difference between the initial weight and the weight lost after samples were dried in the oven at 105°C for 24 h. Crude protein (AOAC #984.13) was determined using the Micro-Kjeldahl method, and the content was estimated by multiplying nitrogen by 6.25 (Foss 2400 Kjeltec analyser unit, Denmark) (Association of Official Analytical Chemists AOAC, Citation2003). Fat content (AOAC #954.02) was determined using solvent extraction with petroleum ether (Soxtec system, Foss Tecator lipid analyser (AOAC, Citation2003). Ash content (AOAC #942.05) was determined by incinerating samples at 550°C for 6 hours in a muffle furnace (Heraeus K1252, Germany) (AOAC, Citation2003). The crude fibre was determined by digesting dried lipid-free residues with 1.25% H2SO4 and 1.25% NaOH. The loss on ignition was recorded as crude fibre (Hlophe-Ginindza et al., Citation2015). Gross energy was analysed using DDS Isothermal CP 500 bomb calorimeter. Neutral detergent fibre (NDF) and Acid detergent fibre (ADF) were determined by boiling sample with a neutral detergent solution and acid detergent solution, respectively (van Soest et al., Citation1991).
2.2. Mineral composition
Selected minerals (Iron, potassium, and sodium) were analysed in the Stinkbug meal. Samples were hydrolysed with 65% concentrated nitric acid and 37% hydrochloric acid. Hydrogen peroxide was used to remove nitrous vapours. The mineral content was then determined using Inductively coupled plasma-optical emission spectrometry (ICP-OES) (Manditsera et al., Citation2019). The analysis was performed using standard solutions of known concentrations. All chemicals used were analytical reagent grade. The selected mineral content of Stinkbug meal is outlined in Table .
2.3. Amino acid profile
Stinkbug meal was analysed for amino acids at the Central Analytical Facilities (CAF) of the Stellenbosch University (South Africa). Samples were hydrolysed with 6 M HCI at 110°C for 22 h in sealed tubes (Ishida et al., Citation1981). Separation was performed on a gas chromatograph (6890N, Agilent Technologies Network) coupled to an Agilent technologies inert XL EI/CI Mass Selective Detector (MSD) (5975, Agilent Technologies Inc., Palo Alto, CA). Amino acid profile of the Stinkbug meal is outlined in Table . The amino acid profile of experimental diets was calculated based on the amino acid profile of the feed ingredients used in experimental diets formulation (Table ).
2.4. Fatty acid profile
The fatty acid methyl esters (FAMEs) for Stinkbug meal were also analysed at the Central Analytical Facilities (CAF) of the Stellenbosch University (South Africa). This was done by adding 2 ml of 2:1 chloroform: methanol solution to 100 mg of the sample (Folch et al., Citation1957). The sample was vortexed and sonicated at room temperature for 30 min. The sample was then centrifuged at 3000 rpm for 1 min. Chloroform (200 µl) was dried completely with a gentle stream of nitrogen and reconstituted and vortexed with 170 µl of methyl tert-butyl ether (MTBE) and 30 µl of trimethylsulfonium hydroxide (TMSH). One µl of the derivitised sample was injected in a 5:1 split ratio onto the GC-FID. Separation was performed on a gas chromatograph (6890N, Agilent Technologies) coupled to flame ionization detector (FID). Separation of the FAMEs was performed on a polar RT-2560 (100 m, 0.25 mm ID, 0.20 µm film thickness) (Restek, USA) capillary column. Hydrogen was used as the carrier gas at a flow rate of 1.2 ml/min. The injector temperature was maintained at 240°C. One µl of the sample was injected in a 5:1 split ratio. The oven temperature was programmed as follows: 100°C for 4 min, ramped to 240°C at a rate of 3 °C/min for 10 min. The fatty acid profile of the Stinkbug meal is outlined in Table . The fatty acid profile of the experimental diets (D1, D2, D3, D4 & D5) is outlined in Table .
2.5. Feed formulation
Five diets were formulated to partially substitute fishmeal at 0, 10, 30, 50, and 70% (Table ). The 0% diet contained 30% fishmeal and served as a control, with no Stinkbug meal. The diets were named D1, D2, D3, D4, and D5, denoting 0, 10, 30, 50, and 70% replacement levels, respectively (Table ). The diets were formulated to be isonitrogenous (30% crude protein), isocaloric (15 MJ/Kg), and isolipidic (12% fat). The Winfeed 3, EFG (Natal) program was used to formulate diets. The Stinkbugs (dried) were purchased from Thohoyandou Town open market. The yellow maize, wheat bran, sunflower meal, and sunflower oil were purchased from a local market. Vitamin/Mineral premix, fishmeal, and binder were purchased from Irvines Africa (Pty) Ltd. Chromic oxide was purchased from Sigma Aldrich. Several studies that used insect meals as fishmeal substitutes have added methionine and lysine in the diets (Cho et al., Citation2022; Djissou et al., Citation2016; Gai et al., Citation2023). To make this study comparable, methionine and lysine were also added in the diets. Moreover, the proximate composition of the Stinkbug meal recorded extremely low levels of methionine (1.76 g/kg) and lysine (6.85 g/kg) that do not meet the requirement for O. mossambicus (methionine: 9.9 g/kg and lysine: 37.8 g/kg). Thus, experimental diets were supplemented with crystalline methionine and lysine which were purchased from Nutroteq Pty Ltd. Chromic oxide (Cr2O3) was used as an inert marker in each diet at 10 g/kg. The ingredients were ground and screened through a 60-mesh size sieve and mixed thoroughly using a planetary mixer (Hobart, Troy, OH, USA) until they were well mixed. During mixing, water was added (10–20% v/w) occasionally until the desired dough thickness was reached. The dough was pelleted through a meat grinder (SLP-45 Shang Hai Taiyi, China) and pushed through to produce extruded strands (2 mm diameter). Pelleted strands were sun-dried and then stored in polyethylene buckets with lids separately at 4°C. The proximate composition of experimental diets is outlined in Table .
Table 2. Ingredients (g/kg, DM) used to formulate experimental diets where Stinkbug meal replaced fishmeal at different replacement levels and the proximate composition of experimental diets
2.6. Experimental design
Feeding trials were conducted at the Aquaculture Research Unit (ARU), University of Limpopo, South Africa. Mozambique tilapia (Oreochromis mossambicus) juveniles were bred at the Aquaculture Research Unit and kept in the hatchery tanks. Before the experiment commenced, fish were collected from the hatchery tanks and placed in a 100 L container with water that was mixed with 2 phenoxyethanol (1 ml/L) as an anaesthetic. Fifteen fish were randomly stocked into 500 L capacity fiberglass experimental tanks in a recirculating system (Filled to 400 L mark). The tanks were connected to a sump and pump that supplied the tanks with water at 10 L/min. The tanks were individually heated with a submersible aquarium heater (ViaAqua Glass heater, 200 W) and aerated with airstones. Fish were left to acclimatise in experimental tanks for 2 weeks while feeding on commercial pellets (Aqua-plus, Avi Products (Pty) Ltd). After the acclimatisation period, fish were anesthetised, and the initial body weight was recorded for each tank. Fish were then randomly allocated to tanks in triplicates and allocated their diets. A total of 15 tanks were used to stock the fish at 15 fish per tank (Stocking density). Fish were fed experimental diets to apparent satiation for 8 weeks twice a day (09:00 h and 15:00 h). Fish were considered satiated when two pellets were left uneaten for 5 min in a tank. Feed intake for each tank was recorded on daily basis. Fish tanks were fitted with the Guelph system, with mild modifications (Cho et al., Citation1982). Faecal matter samples were collected two weeks after the commencement of the experiment from the collection tube. The faecal matter samples were used for protein apparent digestibility coefficient analysis. Water temperature was kept between 27 and 29°C, dissolved oxygen (6.5–8.0 mg/l), ammonia (<1 mg/l), and pH (6.8–8.0). These parameters were monitored on weekly basis using a handheld multiparameter meter (Professional plus YSI 605,000). The experiment was conducted under natural photoperiod. All ethical protocols were observed and approved by the University of Limpopo Animal Research Ethical Committee (AREC/09/2022:PG).
2.7. Growth performance indices
Fish were weighed at the commencement of the trial, after every two weeks, and at the end of the feeding trial. At the end of the experiment, fish were starved for 24 h before growth performance indices were measured. Three fish from each replica (9 fish per treatment) were randomly selected for measurement of growth parameter indices.
For growth performance, the following parameters were calculated:
Specific growth rate (SGR)
Where In, Wt, W0, and t are the natural logarithm, the final body weight (g), initial body weight (g), and time feeding period (days), respectively.
Thermal-unit growth coefficient :
Feed conversion ratio (FCR)= feed consumed (g)/weight gained (g)
Protein efficiency ratio (PER)= increase in body mass (g)/protein consumed (g)
Weight gain (g) = final weight (g)-initial weight(g)
Feed intake (g/fish/day) = weight of eaten feed (g)/number of fish/feeding period (day).
Fish survival rate (%) = (final number of fish/initial number of fish) × 100
Apparent protein digestibility coefficient (APDC) was determined according to Cho et al. (Citation1982)
Chromic oxide in diets and faeces was determined by adding concentrated nitric acid (HNO3) into the samples and then 70% perchloric acid (HCIO4) was added to oxidize chromic oxide until the colour orange appear. The samples were then read for absorbance at 350 nm, using ultrapure water as a blank. Chromic oxide was then calculated as follows:
Weight of chromic oxide in sample = Absorbance − 0.0032/0.2089
Chromic oxide (%) = (weight of chromic oxide/sample weight (mg)) ×100
APDC (%) =
2.8. Organosomatic indices
Fish were collected from experimental tanks and sedated with 2-phenoxyethanol (1 ml/L). Three fish from each dietary replica (9 fish/diet) were used for organosomatic indices. The condition factor, hepato-somatic index, and viscero-somatic index were calculated.
CF= final body weight(g)/fish length(cm)3 ×100
Hepato-somatic index (HSI)= liver weight(g)/fish weight(g) × 100
Viscero-somatic index (VSI)= viscera weight(g)/fish weight(g) × 100
2.9. Blood chemistry
At the end of the feeding trial, fish were fasted for 24 h. Three fish samples from each dietary replica were randomly selected and sedated with 2-phenoxyethanol (1 ml/L). Blood samples (~3 ml) were drawn from the caudal vasculature into vials using heparinised syringes and centrifuged for 15 min at 3000 rpm (Kishawy et al., Citation2022). Blood samples from each replica were pooled. The serum was used for the analysis of triglyceride, cholesterol, aspartate aminotransferase (AST), and alanine aminotransferase (ALT). The analyses were conducted using a commercial automatic biochemical kit and according to the manufacturers protocol (Sigma-Aldrich, USA).
2.10. Liver and intestinal histological analysis
In each dietary replicate, three fish were randomly selected, anaesthetised, weighed, and sacrificed. Fish liver and intestines (distal) were removed and separately fixed in 10% formalin solution until analysis. During analysis, liver samples were cut into 1 cm3 blocks, whilst intestine samples were cut into 1 cm length. All samples were washed for 2 h in distilled water. Samples were then dehydrated in ethanol solution of 70, 80, 96, and 100% levels concentrations. Liver and intestine samples were made transparent using xylene. Samples were infiltrated using Tissue-Tek® III wax in an oven at 60°C. Samples were further embedded in Tissue-Tek® III wax blocks, sectioned at 5 µm thickness (Leica: RM2125 RTS). The sectioned samples were placed in microscope slides and left to dry. After drying, slides were stained with haematoxylin and eosin (H&E) and covered with coverslips as described by van Dyk and Pieterse (Citation2008).
2.11. Liver and intestinal histological assessment
Liver and intestine slides were assessed using a light microscope at different magnifications (Leica Microsystems model DM750, Leica, Bannockburn, IL, USA). The intestine histology was quantified using a modified scoring system from (Baeza-Arińo et al., Citation2014). For villi length and villi thickness, six measurements per slide were taken to establish mean values. Three slides were assessed per treatment. Goblet cells were quantified by counting the number present in each villus. Six villi per slide were quantified to establish mean values. Liver histology was quantified by analysing the liver hepatocyte condition according to McFadzen et al. (Citation1997). The grading was from 1–3, where 1 describes a healthy liver, 2 as intermediate, and 3 as a degraded liver. Dietary replicates were allocated an average grade value based on the dominant histological alterations observed. Three slides were assessed for each replicate.
3. Statistical analysis
Shapiro-Wilk and Barlett’s tests were used to test data for normality of distribution and homogeneity of variance, respectively. One-way analysis of variance (ANOVA) was used to test significant differences in growth parameter indices, organosomatic indices, blood serum chemistry, and histology. The data was significantly different when P < 0.05. Tukey’s HSD post hoc test was used to determine mean differences. The data was analysed using SPSS version 28.0 software package (Statistical Package and Service Solutions, IBM, Chicago, IL, USA).
4. Results
4.1. Proximate composition
Stinkbug meal contained 29.53% protein and 48.59% fat content (Table ). The protein content of the Stinkbug meal was relatively lower than fishmeal (65%). The ash content of the Stinkbug meal was 3.14%, whilst the ash content of fishmeal was 18%. The highest mineral recorded in the Stinkbug meal was sodium (69.2 mg/l).
The essential amino acids (EAA) composition of the Stinkbug meal showed that lysine (6.85 g/kg), leucine (4.77 g/kg), and valine (4.07 g/kg), were the most abundant (Table ). On the other hand, cystine (15.31 g/kg), glutamic acid (10.19 g/kg), and aspartic acid (6.70 g/kg) showed the highest concentration of non-essential amino acids, respectively (Table ). Fishmeal recorded relatively higher amino acid profile than the Stinkbug meal (Table ). Experimental diets contained nine essential amino acids (Table ).
Table 3. Calculated amino acid profile of experimental diets (% of dry matter)
The Stinkbug meal comprised high levels (7.46 mg/g) of saturated fatty acids (SFA) and monounsaturated fatty acid (MUFA) (7.45 mg/g), respectively (Table ). Palmitic fatty acid dominated the SFA, whilst oleic fatty acid dominated the MUFA. Furthermore, Stinkbug meal contained PUFA, linoleic acid which is one of the most essential omega-6 fatty acids. The experimental diets showed that the fatty acid profile was dominated by C18:2 cis (linoleic acid), C18:1 cis (oleic acid), C16 (palmitic acid), and C16:1 (palmitoleic acid) (Table ). Palmitic acid, oleic acid and palmitoleic acid were the fatty acids that increased in experimental diets with an increase in Stinkbug meal.
Table 4. Fatty acid profile of the Stinkbug meal
Table 5. The fatty acid profile of experimental diets (g/kg)
4.2. Growth performance parameters
There was a decrease in growth performance with increasing Stinkbug meal inclusion (Table ). The highest WG (167.37 g), SGR (2.16%), TGC (1.30), and PER (2.95) were recorded in fish fed the control diet (D1). The fish fed the control diet (D1) also had the lowest FCR (1.42). The APDC of fish fed the control diet (79.67%) was higher (P < 0.05) than the rest of the diets. However, diets D2 to D5 showed no significant difference in APDC (P > 0.05). Diet D5 recorded the lowest growth performance and nutrient utilisation indices (Table ). Organosomatic indices (CF, VSI, HSI) were not influenced by Stinkbug meal inclusion (P > 0.05).
Table 6. Growth parameters and organosomatic indices (mean ± SD) of Oreochromis mossambicus fed diets with Stinkbug meal as a fishmeal replacement at increasing substitution levels for 8 weeks. n = 9
4.3. Blood serum chemistry
The ALT values of fish fed the control diet (22.66 U/L) did not differ (P > 0.05) from diets D2, D3, and D4 (Table ). The diet with the highest inclusion level (D5) recorded the highest ALT level (32.66 U/L), which differed significantly (P < 0.05) from the rest of the diets. The AST values showed no discernible pattern. However, the highest AST level was recorded in diet D5 (318.33 U/L) and differed (P < 0.05) from other diets. Stinkbug meal did not influence (P > 0.05) the cholesterol and triglyceride levels among the diets.
Table 7. Blood chemistry (mean ± SD) of Oreochromis mossambicus fed diets with Stinkbug meal at different replacement levels, n = 3
4.4. Liver and intestinal histology
There was no significant difference in the intestine villi height across the diets (P > 0.05) (Table ). However, a numerically higher villi height was recorded in diet D1 (201 µm). Villi thickness also did not differ significantly (P > 0.05) across different diets. However, Diet D1 also recorded the thickest villi (64.66 µm). Substituting fishmeal with stinkbug meal had no influence on the number of goblet cell in the intestine (P > 0.05). Furthermore, the micrographs showed normal intestine at different inclusion levels (Figure ). The liver hepatocyte score was 1 across all diets (Table ). This was confirmed by the normal liver micrographs of fish from all diets (Figure ).
Figure 1. Micrographs of O. mossambicus fed Stinkbug meal as fishmeal replacement at different inclusion levels stained with H& E. A: diet D1 (0%), B: diet D2 (10%), C: diet D3 (30%), D: diets D4 (50%), E: diet D5 (70%). V: villi, LP: Lamina propria, GC: goblet cell, EL: epithelial layer, SM: Submucosa, S: Serosa. Scale bar: 20 µm.
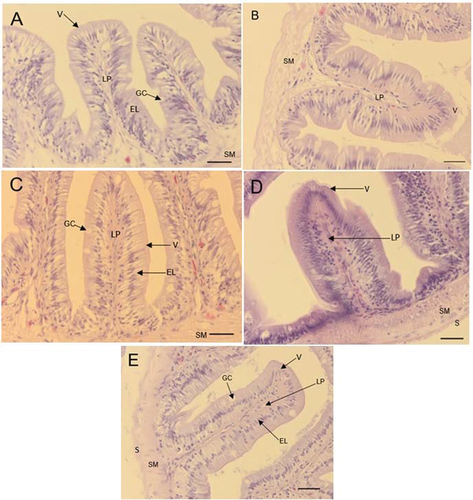
Figure 2. Liver micrographs of O. mossambicus fed diets with Stinkbug meal as a fishmeal replacement. A (control: 0%), B (diet D2; 25%), C (diet D3: 30%), D (diet D4: 50%), and E (diet D5; 75%). V: vacuolisation, N: nucleus, ND: nuclear displacement, HP: Hepatic plate, KC: Kupffer cell. Scale bar: 20 µm.
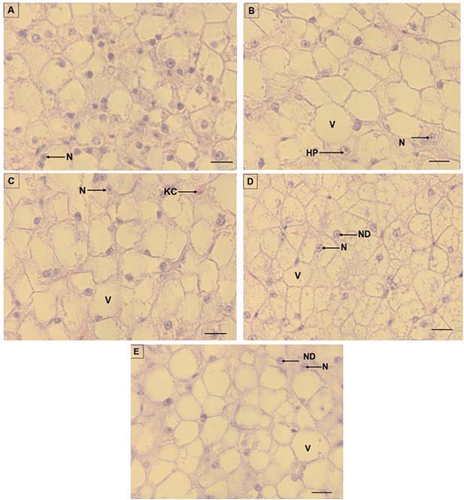
Table 8. The score for the distal intestine of O. mossambicus fed diets with stinkbug meal at different replacement levels. Villi height and thickness are expressed as mean±SD. n = 9
5. Discussion
There was a decrease in the growth performance of O. mossambicus with increasing Stinkbug meal inclusion. The observed decline in growth performance may be linked to an imbalance in the amino acid profile of the Stinkbug meal. Although the Stinkbug meal contained all the essential amino acids, the concentrations recorded did not meet the requirements of O. mossambicus. Methionine and lysine are known to be the most limiting amino acids in fish growth (Inje et al., Citation2018). The study recorded 1.76 g/kg methionine and 6.85 g/kg lysine levels in the Stinkbug meal. These values are relatively lower than the requirement for O. mossambicus. Methionine and lysine requirements for O. mossambicus are 9.9 g/kg and 37.8 g/kg, respectively (Santiago & Lovell, Citation1988). It was thus deemed necessary to supplement experimental diets with crystalline methionine and lysine. The decline in growth performance may also be attributed to an imbalance in the fatty acid profile of the Stinkbug meal. Although Stinkbug meal contains linoleic acid, the study showed that this insect lacks omega-3 fatty acids such as α-linolenic acid, eicosapentaenoic acid (EPA) and docosahexaenoic acid (DHA), which are essential fatty acids for growth. Like most other terrestrial insects, Stinkbug lacks EPA and DHA when compared to aquatic insects (Parmar et al., Citation2022; Twining et al., Citation2016). It is also possible that chitin might have contributed to the decrease in growth performance. Although chitin was not determined, NDF and ADF are taken as surrogate measures of chitin (Finke, Citation2007). Furthermore, chitin interferes with the digestion and absorption of nutrients in fish species, thus compromising fish growth (Gu et al., Citation2022; Tran et al., Citation2022). This phenomenon was also reported in studies where insect meal replaced fishmeal in aquafeed (Anvo et al., Citation2017; Fawole et al., Citation2020; Zarantoniello et al., Citation2021).
There was a noticeable reduction in feed intake with an increase in Stinkbug meal inclusion. This may also have contributed to the reduced growth performance that was observed in fish species. The reduced feed intake may be attributed to trans-2-decenal and trans-2-octenal compounds that the Stinkbug releases as a defence mechanism (Sagun et al., Citation2016). These compounds are known to cause an unpleasant odour that repels predators. The pungent smell was also observed in diets with Stinkbug meal. It is speculated that the Stinkbug odour reduced voluntary feed intake. Although there was a decline in the growth performance, organosomatic indices (CF, HSI, VSI) were not significantly different across all diets. The condition factor (CF) explains the well-being of fish species. In this study, Stinkbug meal did not influence the condition factor. This shows that fish from all experimental diets were in good health. The hepatosomatic index followed a similar trend. This index reveals the health status of the liver. Thus, utilising diets with Stinkbug meal as a fishmeal replacement did not cause any metabolic or liver deficiencies in O. mossambicus. The Viscero-somatic index also did not differ significantly among diets. This confirms that no excessive hepatic fat was accumulated in fish from all diets. Similar findings were observed in fish that have been fed insect meal as a fishmeal substitute (Renna et al., Citation2017; Tippayadara et al., Citation2021; Xiao et al., Citation2018). This suggests that Stinkbug meal had no negative effects on the health status of O. mossambicus.
The Stinkbug meal had no influence on the ALT and AST levels. The ALT and AST are liver enzymes that often leak into the bloodstream when there is hepatic damage or increased stress level (Adeoye et al., Citation2020). Belghit et al. (Citation2018) indicated that feeding an Atlantic salmon (Salmo salar) a diet with H. illucens as a fishmeal substitute did not affect the ALT and AST levels. Salmo salar is one of the fish species that feed on aquatic insects in nature, and it is reportedly pre-adapted to utilising insect-based diets (Henry et al., Citation2015). In the wild, O. mossambicus also feeds on insects (El-Sayed, Citation2020). It is probably also pre-adapted to utilising insect-based diets. The Stinkbug meal had no effect on the cholesterol and triglyceride levels across diets. Cholesterol levels in fish fed insect-based diets are known to be reduced due to chitin level (Belghit et al., Citation2018). However, in this study the levels of cholesterol may have not been affected by the chitin level. Similar observations were recorded when Argyrosomus japonicus was fed diets with H. illucens as a fishmeal replacement (Madibana et al., Citation2020).
Substituting fishmeal with Stinkbug meal did not affect the liver and intestine histology of O. mossambicus from all experimental diets. The liver is an organ involved in nutrient metabolism (Rašković et al., Citation2011). It is also a good indicator of the nutritional status of the fish. Changes in its morphological structure is paramount in determining the capacity of a species to digest and effectively utilise a diet. Lack of liver histological alterations shows that O. mossambicus can utilise an insect-based diet. The intestine villi and thickness across diets did not differ significantly. The goblet cell prevalence was not influenced by the Stinkbug meal in all experimental diets. Goblet cells are responsible for secreting mucus as a protective compound (Kim & Ho, Citation2010). This shows that the Stinkbug meal did not have detrimental effects on the intestine histology of O. mossambicus. The intestine is an organ where feed digestion and nutrient absorption take place (Elia et al., Citation2018). This further shows that O. mossambicus utilised an insect-based diet without adverse effects. This is probably because this species has requisites digestive enzymes to handle an insect-based diet. This agrees with a study where O. mossambicus successfully utilised a diet where mopane worm replaced fishmeal (Rapatsa & Moyo, Citation2017). The study shows that the Stinkbug meal may partially replace fishmeal at 30% inclusion level. This is because at inclusion levels beyond 30%, the growth performance and nutrient utilisation declined significantly. Moreover, at 70% inclusion level, the AST and ALT levels increased significantly. High levels of these liver enzymes may have moderate metabolic effects on juvenile O. mossambicus. Furthermore, the FCR recorded at 30% inclusion level (1.72) showed that fish fed this diet were able to convert feed intake into body weight gain. To the best of the authors knowledge, there is no study that evaluated using Stinkbug meal as fishmeal replacement in aqua feeds. Thus, there are no comparable results for this study. The limitation associated with feeding O. mossambicus diets with Stinkbug meal is its poor growth performance at higher inclusion levels. Another limitation of using Stinkbug meal is its limited availability and seasonal variation. Stinkbug meal also contain high fat content which may cause oxidation in the diets.
6. Conclusion
This study evaluated the effects of dietary full-fat Stinkbug meal on the growth performance, blood serum chemistry, and liver and intestinal histology of juvenile O. mossambicus. The study showed that growth performance declined with an increase in Stinkbug meal. However, the organosomatic indices were not influenced by the inclusion of Stinkbug meal in O. mossambicus diets. The AST and ALT values were also not influenced by the Stinkbug meal inclusion. However, at the highest inclusion level, these enzymes were elevated. The liver and intestine were also not influenced by the Stinkbug meal inclusion. The main limitation with using Stinkbug meal as a fishmeal replacement is its high fat content and an imbalance in fatty acid profile. A cost benefit analysis to determine the economic viability of using Stinkbug meal as a fishmeal replacement is recommended.
Authors’ contributions
Moyo N.A.G. and Rapatsa M.M. conceptualized the study. Nephale L.E. conducted experimental trials, analysed and interpreted the data. The manuscript was drafted by Nephale L.E. and all authors read and revised the manuscript. All authors approved the final manuscript.
Acknowledgments
The National Research Foundation (NRF) and the University of Limpopo, Aquaculture Research Unit are recognised for financial support. Mr. Gavin Geldenhuys is appreciated for his technical assistance.
Disclosure statement
No potential conflict of interest was reported by the author(s).
Data availability statement
Data is available upon request from the corresponding author(s).
Additional information
Funding
Notes on contributors
Livhuwani E. Nephale
Livhuwani Eva Nephale is a PhD candidate at the University of Limpopo, Aquaculture Research Unit. She holds an MSc in Aquaculture from the University of Limpopo. Her current research interest is fish nutrition.
Ngonidzashe A.G. Moyo
Prof N. A. G Moyo is an established researcher with more than 30 years’ experience in Aquatic ecosystems including Aquaculture. He holds a PhD from University of Waterloo in Canada and has supervised more than 10 PhD students.
Mmaditshaba M. Rapatsa
Prof M. M Rapatsa is an upcoming researcher. She holds a PhD in aquaculture from the University of Limpopo and has published more than 15 papers in Aquaculture.
References
- Abdel-Tawwab, M., Khalil, R. H., Metwally, A. A., Shakweer, M. S., Khallaf, M. A., & Abdel-Latif, H. M. R. (2020). Effects of black soldier fly (Hermetia illucens L.) larvae meal on growth performance, organs-somatic indices, body composition, and hematobiochemical variables of European sea bass, Dicentrarchus labrax. Aquaculture 522, 735136. Article 735136. https://doi.org/10.1016/j.aquaculture.2020.735136
- Adeoye, A. A., Akegbejo-Samsons, Y., Fawole, F. J., & Davies, S. J. (2020). Preliminary assessment of black soldier fly (Hermetia illucens) larval meal in the diet of African catfish (Clarias gariepinus): Impact on growth, body index, and hematological parameters. Journal of the World Aquaculture Society, 51(4), 1024–17. https://doi.org/10.1111/jwas.12691
- Akinwole, A. O., Dauda, A. B., & Ogunkunle, V. O. (2020). Growth performance, nutrient utilization, survival and body indices of African catfish (Clarias gariepinus, Burchell 1822) reared on maggot meal based diet. FUDMA Journal of Sciences, 4(2), 53–59. https://doi.org/10.33003/fjs-2020-0402-109
- Alfiko, Y., Xie, D., Astuti, R. T., Wong, J., & Wang, L. (2022). Insects as a feed ingredient for fish culture: Status and trends. Aquaculture and Fisheries, 7(2), 166–178. https://doi.org/10.1016/j.aaf.2021.10.004
- Anvo, M. P. M., Aboua, B. R. D., Compaoré, I., Siaaao, R., Zoungrana-Kaboré, C. Y., Kouamelan, E. P., & Toguyéni, A. (2017). Fish meal replacement by Cirina butyrospermi caterpillar’s meal in practical diets for Clarias gariepinus fingerlings. Aquaculture Research, 48(10), 5243–5250. https://doi.org/10.1111/are.13337
- AOAC International. (2003). Official methods of analysis of AOAC International (17th ed.). Gaithersburg, MD, USA, Association of Analytical Communities.
- Baeza-Arińo, R., Martĩnez-Llorens, S., Nogales-Merida, S., Jover-Cerda, M., & Tomas-Vidal, A. (2014). Study of liver and gut alterations in sea bream, Sparus aurata L., fed a mixture of vegetable protein concentrate. Aquaculture Research, 47(2), 460–471. https://doi.org/10.1111/are.12507
- Belghit, I., Liland, N. S., Gjesdal, P., Biancarosa, I., Menchetti, E., Li, Y., Waagobo, R., & Krogdahl, E. L. (2018). Black soldier fly larvae meal can replace fish meal in diets of sea-water phase Atlantic salmon (Salmo salar). Aquaculture, 503, 609–619. https://doi.org/10.1016/j.aquaculture.2018.12.032
- Bruni, L., Pastorelli, R., Viti, C., Gasco, L., & Parisi, G. (2018). Characterisation of the intestinal microbial communities of rainbow trout (Oncorhynchus mykiss) fed with Hermetia illucens (black soldier fly) partially defatted larva meal as partial dietary protein source. Aquaculture, 487, 56–63. https://doi.org/10.1016/j.aquaculture.2018.01.006
- Cho, J., Bae, J., & Hwang, I. (2022). Effects of black soldier fly (Hermetia illucens) pre-pupae meal on the growth, stress, and immune responses of juvenile rainbow trout (Oncorhynchus mykiss) reared at different stocking densities. Aquaculture Reports, 25, Article ID 101202.
- Cho, C. Y., Slinger, S. J., & Bayley, H. S. (1982). Bioenergetics of salmonid fishes energy intake, expenditure and productivity. Comparative Biochemistry and Physiology, 73(1), 25–41. https://doi.org/10.1016/0305-0491(82)90198-5
- Coutinho, F., Castro, C., Guerreiro, I., Rangel, F., Couto, A., Serra, C. R., Peres, H., Pousão-Ferreira, P., Rawski, M., Oliva-Teles, A., & Enes, P. (2021). Mealworm larvae meal in diets for meagre juveniles: Growth, nutrient digestibility and digestive enzymes activity. Aquaculture 535, 736362. Article 736362. https://doi.org/10.1016/j.aquaculture.2021.736362
- Devic, E., Leschen, W., Murray, F., & Little, D. C. (2017). Growth performance, feed utilization and body composition of advanced nursing Nile tilapia (Oreochromis niloticus) fed diets containing black soldier fly (Hermetia illucens) larvae meal. Aquaculture Nutrition, 24(1), 416–423. https://doi.org/10.1111/anu.12573
- Djissou, A. S. M., Adjahouinou, D. C., Koshio, S., & Fiogbe, E. D. (2016). Complete replacement of fish meal by other animal protein sources on growth performance of Clarias gariepinus fingerlings. International Aquatic Research, 8(4), 333–341. https://doi.org/10.1007/s40071-016-0146-x
- Elia, A. C., Capucchio, M. T., Caldaroni, B., Magara, G., Dörr, A. J. M., Biasato, I., Biasibetti, E., Righetti, M., Pastorino, P., Prearo, M., Gai, F., Schiavone, A., & Gasco, L. (2018). Influence of Hermetia illucens meal dietary inclusion on the histological traits, gut mucin composition and the oxidative stress biomarkers in rainbow trout (Oncorhynchus mykiss). Aquaculture, 496, 50–57. https://doi.org/10.1016/j.aquaculture.2018.07.009
- El-Sayed, A. F. M. (2020). Tilapia culture (2nd ed.). Academic Press.
- Fawole, F. J., Adeoye, A. A., Tiamiyu, L. O., Ajala, K. I., Obadara, S. O., & Ganiyu, I. O. (2020). Substituting fishmeal with Hermetia illucens in the diets of African catfish (Clarias gariepinus): Effects on growth, nutrient utilization, haemato-physiological response, and oxidative stress biomarker. Aquaculture 518, 734849. Article 734849. https://doi.org/10.1016/j.aquaculture.2019.734849
- Finke, M. D. (2007). Estimate of chitin in raw whole insects. Zoo Biology, 26(2), 105–115. https://doi.org/10.1002/zoo.20123
- Fischer, H., Romano, N., Renukdas, N., Kumar, V., & Sinha, A. K. (2022). Comparing black soldier fly (Hermetia illucens) larvae versus prepupae in the diets of largemouth bass, Micropterus salmoides: Effects on their growth, biochemical composition, histopathology, and gene expression. Aquaculture 546, 737323. Article 737323. https://doi.org/10.1016/j.aquaculture.2021.737323
- Folch, J., Lees, M., & Stanley, G. H. S. (1957). A simple method for the isolation and purification of total lipids from animal tissues. Journal of Biological Chemistry, 226(1), 497–509. https://doi.org/10.1016/S0021-9258(18)64849-5
- Gai, F., Cusimano, G. M., Maricchiolo, G., Caccamo, L., Caimi, C., Macchi, E., Meola, M., Perdichizzi, A., Tartarisco, G., & Gasco, L. (2023). Defatted black soldier fly meal in diet for grow-out gilthead seabream (Sparus aurata L. 1758): Effects on growth performance, Gill Cortisol level, digestive enzyme activities, and intestinal histological structure. Aquaculture Research, ID, 3465335. https://doi.org/10.1155/2023/3465335
- Gbai, M., Ouattara, N., Bamba, Y., Ouattara, M., Ouattara, A., & Yao, K. (2019). Substitution of the fishmeal by maggot meal in the feed of Nile tilapia Oreochromis niloticus at different stages of growth. International Journal of Fisheries and Aquaculture Research, 5(4), 25–39. . www.eajournals.org
- Guerreiro, I., Serra, C. R., Coutinho, F., Couto, A., Castro, C., Rangel, F., Pres, H., Pousão-Ferreira, P., Matos, E., Gasco, L., Gai, F., Olivia-Teles, A., & Enes, P. (2020). Digestive enzyme activity and nutrient digestibility in meagre (Argyrosomus regius) fed increasing levels of black soldier fly meal (Hermetia illucens). Aquaculture Nutrition, 27(1), 142–152. https://doi.org/10.1111/anu.13172
- Gu, J., Liang, H., Ge, X., Xia, D., Pan, L., Mi, H., & Ren, M. (2022). A study of the potential effect of yellow mealworm (Tenebrio molitor) substitution for fish meal on growth, immune and antioxidant capacity in juvenile largemouth bass (Micropterus salmoides). Fish and Shellfish Immunology, 120, 214–221. https://doi.org/10.1016/j.fsi.2021.11.024
- Henry, M., Gasco, L., Piccolo, G., & Fountoulaki, E. (2015). Review on the use of insects in the diet of farmed fish: Past and future. Animal Feed Science and Technology, 203, 1–22. https://doi.org/10.1016/j.anifeedsci.2015.03.001
- Henry, M. A., Golomazou, E., Asimaki, A., Psofakis, P., Fountoulaki, E., Mente, E., Rumbos, C. I., Athanassiou, C. G., & Karapanagiotidis, I. T. (2022). Partial dietary fishmeal replacement with full-fat or defatted superworm (Zophobas morio) larvae meals modulates the innate immune system of gilthead seabream, Sparus aurata. Aquaculture Reports, 27, Article 101347.
- Hlophe-Ginindza, S. N., Moyo, N. A. G., & Ngambi, J. W. (2015). The effect of exogenous enzyme supplementation on growth and digestive enzyme activities in Oreochromis mossambicus fed kikuyu-based diets. Aquaculture Research, 47(12), 3777–3787. https://doi.org/10.1111/are.12828
- Hua, K. (2021). A meta-analysis of the effects of replacing fish meals with insect meals on growth performance of fish. Aquaculture 530, 735732. Article 735732. https://doi.org/10.1016/j.aquaculture.2020.735732
- Hu, Y., Huang, Y., Tang, T., Zhong, L., Chu, W., Dai, Z., Chen, Hu, Y., & Chen, K. (2020). Effect of partial black soldier fly (Hermetia illucens L.) larvae meal replacement of fish meal in practical diets on the growth, digestive enzyme and related gene expression for rice field eel (Monopterus albus). Aquaculture Reports 17, 100345. Article 100345. https://doi.org/10.1016/j.aqrep.2020.100345
- Inje, O. F., Olufunmilayo, A. H., Audu, J. A., Ndaman, S. A., & Chidi, E. E. (2018). Protein quality of four indigenous edible insect species in Nigeria. Food Science and Human Wellness, 7(2), 175–183. https://doi.org/10.1016/j.fshw.2018.05.003
- Ishida, Y., Fujita, T., & Asai, K. (1981). New detection and separation method for amino acids by high-performance liquid chromatography. Journal of Chromatography A, 204, 143–148. https://doi.org/10.1016/S0021-9673(00)81650-7
- Jabir, A. R., Razak, S. A., & Vikineswary, S. (2012). Nutritive potential and utilization of super worm (Zophobas morio) meal in the diet of Nile tilapia (Oreochromis niloticus) juvenile. African Journal of Biotechnology, 11(24), 6592–6598. https://doi.org/10.5897/AJB11.1084
- Jeong, S. M., Khosravi, S., Mauliasari, I. R., & Lee, S. M. (2020). Dietary inclusion of mealworm (Tenebrio molitor) meal as an alternative protein source in practical diets for rainbow trout (Oncorhynchus mykiss) fry. Fisheries and Aquatic Sciences, 23(1), 12. https://doi.org/10.1186/s41240-020-00158-7
- Kim, Y. S., & Ho, S. B. (2010). Intestinal goblet cells and mucins in health and disease: Recent insights and progress. Current Gastroenterology Reports, 12(5), 319–330. https://doi.org/10.1007/s11894-010-0131-2
- Kishawy, A. T. Y., Mohammed, H. A., Zaglool, A. W., Attia, M. S., Hassan, F. A. M., Roushdy, E. M., Ismail, T. A., & Ibrahim, D. (2022). Partial defatted black solider larvae meal as a promising strategy to replace fish meal protein in diet for Nile tilapia (Oreochromis niloticus): Performance, expression of protein and fat transporters, and cytokines related genes and economic efficiency. Aquaculture 555, 738195. Article 738195. https://doi.org/10.1016/j.aquaculture.2022.738195
- Llagostera, P. F., Kallas, Z., Reig, L., & Amores de Gea, D. (2019). The use of insect meal as a sustainable feeding alternative in aquaculture: Current situation, Spanish consumers’ perceptions and willingness to pay. Journal of Cleaner Production, 229, 10–21. https://doi.org/10.1016/j.jclepro.2019.05.012
- Madibana, M. J., Mwanza, M., Lewis, B. R., Fouché, C. H., Toefy, R., & Mlambo, V. (2020). Black soldier fly larvae meal as a fishmeal substitute in juvenile dusky kob diets: Effect on feed utilization, growth performance, and Blood parameters. Sustainability, 12(22), 9460. https://doi.org/10.3390/su12229460
- Makkar, H. P. S., Tran, G., Heuze, V., & Ankers, P. (2014). State-of-the-art on use of insects as animal feed. Animal Feed Science and Technology, 197, 1–33. https://doi.org/10.1016/j.anifeedsci.2014.07.008
- Makore, T. A., Garamumhango, P., Chirikure, T., & Chikambi, S. D. (2015). Determination of nutritional composition of Encosternum delegorguei caught in Nerumedzo community of Bikita, Zimbabwe. International Journal of Biology, 7(4), 4. https://doi.org/10.5539/ijb.v7n4p13
- Manditsera, F. A., Luning, P. A., Fogliano, V., & Lakemond, C. M. M. (2019). The contribution of wild harvested edible insects (Eulepida mashona and Henicus whellani) to nutrition security in Zimbabwe. Journal of Food Composition and Analysis, 75, 17–25. https://doi.org/10.1016/j.jfca.2018.09.013
- McFadzen, I. R. B., Coombs, S. H., & Halliday, N. C. (1997). Histological indices of the nutritional condition of sardine, Sardina pilchardus (walbaum) larvae of the north coast of Spain. Journal of Experimental Marine Biology and Ecology, 212(2), 239–258. https://doi.org/10.1016/S0022-0981(96)02755-4
- Mohan, K., Rajan, D. K., Muralisankar, T., Ganesan, A. R., Sathishkumar, P., & Revathi, N. (2022). Use of black soldier fly (Hermetia illucens L.) larvae meal in aquafeeds for a sustainable aquaculture industry: A review of past and future needs. Aquaculture 553, 738095. Article 738095. https://doi.org/10.1016/j.aquaculture.2022.738095
- Muin, H., & Taufek, N. M. (2022). Evaluation of growth performance, feed efficiency and nutrient digestibility of red hybrid tilapia fed dietary inclusion of black soldier fly larvae (Hermetia illucens). Aquaculture and Fisheries. https://doi.org/10.1016/j.aaf.2022.09.006
- Nyuliwe, T. C., Mlambo, V., Madibana, M. J., Mwanza, M., & Wokadala, O. C. (2022). Partial substitution of fishmeal with mopane worm meal in dusky kob fingerling (Argyrosomus japonicus) diets: Feed utilization, digestive enzyme activity, Blood parameters, and growth performance. Aquaculture Journal, 2(2), 59–71. https://doi.org/10.3390/aquacj2020006
- Parmar, T. P., Kindinger, A. L., Mathieu-Resuge, M., Twining, C. W., Shipley, J. R., Kainz, M. J., & Martin-Creuzburg, D. (2022). Fatty acid composition differs between emergent aquatic and terrestrial insects-A detailed single system approach. Frontiers in Ecology and Evolution, 10. Article 952292. https://doi.org/10.3389/fevo.2022.952292
- Prachom, N., Boonyoung, S., Hassaan, M. S., El-Haroun, E., & Davies, S. J. (2021). Preliminary evaluation of superworm (Zophobas morio) larval meal as a partial protein source in experimental diets for juvenile Asian sea bass, lates calcarifer. Aquaculture Nutrition, 27(5), 1-1304–1314. https://doi.org/10.1111/anu.13269
- Rapatsa, M. M., & Moyo, N. A. G. (2017). Evaluation of Imbrasia belina meal as a fishmeal substitute in Oreochromis mossambicus diets: Growth performance, histological analysis and enzyme activity. Aquaculture Reports, 5, 18–26. https://doi.org/10.1016/j.aqrep.2016.11.004
- Rapatsa, M. M., & Moyo, N. A. G. (2019). Enzyme activity and histological analysis of Clarias gariepinus fed on Imbrasia belina meal used for partial replacement of fishmeal. Fish Physiology and Biochemistry, 45(4), 1309–1320. https://doi.org/10.1007/s10695-019-00652-3
- Rapatsa, M. M., Moyo, N. A. G. (2022). A review and meta-analysis of the effects of Replacing fishmeal with insect meals on growth of tilapias and sharptooth catfish. Aquaculture Nutrition 2022, 1–10. Article 9367587. https://doi.org/10.1155/2022/9367587
- Rašković, B. S., Stanković, M. B., Marković, Z. Z., & Poleksić, V. D. (2011). Histological methods in the assessment of different feed effects on liver and intestine of fish. Journal of Agricultural Sciences, 56(1), 87–100. https://doi.org/10.2298/JAS1101087R
- Renna, M., Schiavone, A., Gai, F., Dabbou, S., Lussiana, C., Malfatto, V., Prearo, M., Capucchio, M. T., Biasato, I., Biasibetti, E., De Marco, M., Brugiapaglia, A., Zoccarato, I., & Gasco, L. (2017). Evaluation of the suitability of a partially defatted black soldier fly (Hermetia illucens L.) larvae meal as ingredient for rainbow trout (Oncorhynchus mykiss walbaum) diets. Journal of Animal Science and Biotechnology, 8(1). Article 57. https://doi.org/10.1186/s40104-017-0191-3
- Rumpold, B. A., & Schlüter, O. K. (2013). Potential and challenges of insects as an innovative source for food and feed production. Innovative Food Science and Emerging Technologies, 17, 1–11. https://doi.org/10.1016/j.ifset.2012.11.005
- Sagun, S., Collins, E., Martin, C., Nolan, E. J., & Horzempa, J. (2016). Alarm odor compounds of the brown marmorated stink bug exhibit antibacterial activity. Journal of Pharmacognosy and Natural Products, 2(3), 119. https://doi.org/10.4172/2472-0992.1000119
- Saleh, H. H. E. (2020). Effect of feeding on fresh (wet) housefly maggots (Musca domestica) with or without Artificial diet on water Quality and growth Rates of African catfish (Clarias gariepinus Burchell, 1822) fry under laboratory conditions. Journal of Zoological Research, 2(2). https://doi.org/10.30564/jzr.v2i2.2053
- Santiago, C. B., & Lovell, R. T. (1988). Amino acid requirements for growth of Nile tilapia. Journal of Nutrition, 188(12), 1540–1546. https://doi.org/10.1093/jn/118.12.1540
- Teffo, L. S., Toms, R. B., & Eloff, J. N. (2007). Preliminary data on the nutritional composition of the composition of the edible stink-bug, ncosternum delegorguei Spinola, consumed in Limpopo province, South Africa. South African Journal of Science, 103, 434–436. https://hdl.handle.net/10520/EJC96630
- Terova, G., Gini, E., Gasco, L., Moroni, F., Antonini, M., & Rimoldi, S. (2021). Effects of full replacement of dietary fishmeal with insect meal from Tenebrio molitor on rainbow trout gut and skin microbiota. Journal of Animal Science and Biotechnology, 12(1). Article 30. https://doi.org/10.1186/s40104-021-00551-9
- Tippayadara, N., Dawood, M. A. O., Krutmuang, P., Hoseinifar, S. H., Doan, H. V., & Paolucci, M. (2021). Replacement of fish meal by black soldier fly (Hermetia illucens) larvae meal: Effects on growth, haematology, and skin mucus immunity of Nile tilapia, Oreochromis niloticus. Animals, 11(1), 193. https://doi.org/10.3390/ani11010193
- Tran, H. Q., Prokešová, M., Zare, M., Matoušek, J., Ferrocino, I., Gasco, L., & Stejskal, V. (2022). Production performance, nutrient digestibility, serum biochemistry, fillet composition, intestinal microbiota and environmental impacts of European perch (Perca fluviatilis) fed defatted mealworm (Tenebrio molitor). Aquaculture 547, 737499. Article 737499. https://doi.org/10.1016/j.aquaculture.2021.737499
- Tschirner, M., & Simon, A. (2015). Influence of different growing substrates and processing on the nutrient composition of black soldier fly larvae destined for animal feed. Journal of Insects as Food and Feed, 1(4), 249–259. https://doi.org/10.3920/JIFF2014.0008
- Twining, C. W., Brenna, J. T., Lawrence, P., Shipley, J. R., Tollefson, T. N., & Winkler, D. W. (2016). Omega-3 long-chain polyunsaturated fatty acids support aerial insectivore performance more than food quantity. Proceedings of the National Academy of Sciences, 113(39), 10920–10925. https://doi.org/10.1073/pnas.1603998113
- van Dyk, J. C., & Pieterse, G. M. (2008). A histo-morphological study of the testis of the sharptooth catfish (Clarias gariepinus) as reference for future toxicological assessments. Journal of Applied Ichthyology, 24(4), 415–422. https://doi.org/10.1111/j.1439-0426.2008.01127.x
- van Huis, A. (2013). Potential of insects as food and feed in assuring food security. Annual Review of Entomology, 58(1), 563–583. https://doi.org/10.1146/annurev-ento-120811-153704
- van Huis, A. (2022). Edible insects: Challenges and prospects. Entomological Research, 52(4), 161–177. https://doi.org/10.1111/1748-5967.12582
- van Soest, P. J., Robertson, J. B., & Lewis, B. A. (1991). Methods for dietary fiber, neutral detergent fiber and nonstarch polysaccharides in relation to animal nutrition. Journal of Dairy Science, 74(10), 3583–3597. https://doi.org/10.3168/jds.S0022-0302(91)78551-2
- Xiao, X., Jin, P., Zheng, L., Cai, M., Yu, Z., Yu, J., & Zhang, J. (2018). Effects of black soldier fly (Hermetia illucens) larvae meal protein as a fishmeal replacement on the growth and immune index of yellow catfish (Pelteobagrus fulvidraco). Aquaculture Research, 49(4), 1–9. https://doi.org/10.1111/are.13611
- Zarantoniello, M., Randazzo, B., Secci, G., Notarstefano, V., Giorgini, E., Lock, E. J., Parisi, G., & Olivotto, I. (2021). Application of laboratory methods for understanding fish responses to black soldier fly (Hermetia illucens) based diets. Journal of Insects as Food and Feed, 8(11), 1173–1195. https://doi.org/10.3920/JIFF2020.0135