Abstract
Low nutrient availability in sandy soils is a serious threat to crop productivity. The present study evaluated the effectiveness of cotton-sticks biochar, sugarcane press-mud, and vegetable compost on soil chemical properties and chickpea yield in sandy soils. Two chickpea varieties, Bittal-2016 and Noor-2019, were grown in soil amended with cotton-sticks biochar at 10 and 15 Mg ha−1; sugarcane press-mud at 10 and 15 Mg ha−1; vegetable compost at 10 and 15 Mg ha−1; cotton-sticks biochar at 10 and 15 Mg ha−1 plus loaded with Fe/Zn including no amendment. Cotton-sticks biochar at 15 Mg ha−1 loaded with Fe/Zn significantly increased chlorophyll (48.40), N (3.1, 2.5, and 3.9%), P (1.13%, 1.10, and 1.4%), and K (1.44, 2.26, and 1.27%) in root, shoot, and grains of var. Noor-2019. Soil amended with vegetable compost at 10 Mg ha−1 and Fe/Zn-loaded biochar at 15 Mg ha−1 exhibited a marked increase in shoot length (43.2 cm), root length (25.8 cm), root fresh weight (8.0 g), pods per plant (34), 100-grain weight (32.7 g), and grain yield (25.9 g) for var. Noor-2019. Amended soil with Fe/Zn-loaded biochar at 10 Mg ha−1 depicted a remarkable increase in soil EC, pH, OM, and N by 2.12 dS m−1, 8.4, 0.67% and 0.20%, respectively. Conclusively, Fe/Zn-loaded cotton-sticks biochar at 15 Mg ha−1 and vegetable compost at 10 Mg ha−1 effectively improved the soil chemical properties, nutrient uptake, and yield of var. Noor-2019. Hence, cotton-sticks biochar could be used as eco-friendly approach for improving chickpea production in coarse-textured soils.
1. Introduction
Chickpea (Cicer arietinum L.) is an important pulse crop that provides carbohydrates, vitamins, carotene, dietary protein, fiber, and mineral elements (e.g. iron, zinc, calcium, phosphorus, magnesium contents to humans) (Mustafa et al., Citation2022). It is widely grown in arid or semi-arid regions of the world with an annual production of 11–13 million tons. The major chickpea producing countries include Turkey (80%), India (48.5%), and Pakistan (70%) (Bar El Dadon et al., Citation2017; Batool et al., Citation2021). Since chickpea is cultivated around the globe, hence, it is essential to address the challenges related to its low production. The cultivation of chickpea in sandy soils under rain-fed conditions with reduced soil fertility and gradually depleting soil moisture are considered the most critical factors and pose a severe menace to food security for millions of people worldwide (Hajarpoor et al., Citation2014; Zingore et al., Citation2008).
Sandy soils are normally deficient in organic matter (<1%), nutrients availability and having low water holding capacity that pose serious threat to crop production. These soils have high macroporosity, high infiltration rate, high percolation and leaching, excessive drainage, low moisture retention ability, high erodibility, low retention of added nutrients, high evapotranspiration demands, and high surface temperature, making the soils unproductive for normal plant development (Herawati et al., Citation2021; Osman, Citation2018). Plants grown in coarse-textured soils usually experience drought exposure, hence causing severe decline in crop yield due to high temperature and prolonged water scarce conditions (Case et al., Citation2012; Laghari et al., Citation2015; Pradhan et al., Citation2022). The susceptibility to drought stress in sandy soils is due to collapse and slumping of the peds causing soil particles to pack too close, alter pore size distribution, and diminish water availability (Suzuki et al., Citation2007). Cultivation of food crops in sandy soils have also confronted serious limitations for nutrients availability associated with decline in cation exchange capacity (CEC) of soils (Reverchon et al., Citation2014). Poor-textured sandy soils are inherently low in fertility due to rapid oxidation of organic matter because of high soil temperature and aeration. In Pakistan, chickpea is mostly grown on sandy soils that are generally susceptible to macro- and micronutrient deficiency because of high soil pH and quick loss of added fertilizers through leaching (He et al., Citation2021; Osman, Citation2018). Coarse-textured sandy soils are particularly deficient in clay contents that limits the buffering capacity, water accessibility, and humus accumulation, further aggravating the mineral nutrient deficiencies for growing plants. Low crop yield in sandy soils is due to its poor aggregation-induced vulnerability to erosion and having inadequate nutrient-bearing capacity by intensive leaching of supplied nutrients from the top fertile soil layers into deeper soil horizon (Croker et al., Citation2004; Czaban & Siebielec, Citation2013; Zingore et al., Citation2008).
As anticipated, sandy soils also have a wide range of limitations for nutrients availability, in particular, iron (Fe) and zinc (Zn) (Osman, Citation2018; Pal et al., Citation2019). Fe and/or Zn are important micronutrients involved in various metabolic and physiological processes in plants, while the scarcity leads to major yield reduction in food crops. The deficiency of such nutrients is more prevalent in chickpea cultivated soils. In Pakistan, chickpea is largely grown on sandy soils that are susceptible to Fe and Zn deficiency, coupled with drought that chickpea face during its whole growth cycle leading to poor growth and delayed crop maturity (Mahmoud et al., Citation2022; Ullah et al., Citation2020).
Sustainable management of degraded/sandy soils requires potential approaches that improve physico-chemical characteristics and CEC of the soil associated with higher organic carbon content (Croker et al., Citation2004). Use of inorganic fertilizers to fulfill the nutritional demand of plants is restricted in sandy soils due to its reduced availability by excessive leaching. A cost-effective strategy for farmers to improve soil physical and chemical properties of degraded soils to promise its higher nutrient retention capacity may reside in the application of organic amendments (Sukartono, Citation2011). Supplemental use of organic sources (e.g. composts, press mud or manures) has frequently been reported to improve CEC, water retention ability, and fertility status, but have a short life duration in soils because of rapid decomposition of organic matter (Alharby & Fahad, Citation2020; Khan et al., Citation2021).
Soils amended with more stable carbon compounds (e.g. biochar, charged organic material) could be an alternative to ordinary degradable organic sources. Biochar could be used as adsorbent for carbon sequestration and have potential to improve the nutrient holding capacity of soils via reducing the leaching of nutrients (Fatima et al., Citation2022; Selvarajoo et al., Citation2022). In addition, biochar is also effective to remove the organic pollutants from wastewater (Babar et al., Citation2022; François et al., Citation2023). It is evident that biochar has a key role in improving soil physical and chemical conditions, organic matter, water and nutrient availability in sandy soils (Lusiba et al., Citation2018; Pradhan et al., Citation2022). As a carbon-rich material, biochar has a porous structure with high specific surface area which improves the soil structure and aeration, nutrient dynamics and water holding capacity (Bashir et al., Citation2020; Shaaban et al., Citation2018). Biochar has a greater cation anion adsorption capacity per unit of carbon because of containing higher charge density and negative surface charge (Fahad et al., Citation2016), and thus reduces the leaching of nutrient ions and improving the CEC of soils. Soils amended with biochar has a potential to increase the availability of nutrients (e.g. N, C, and P) to growing plants by means of increasing microbial activities and arbuscular mycorrhizal fungal colonization in sandy soils (Ogola et al., Citation2021; Sg et al., Citation2021; Solaiman et al., Citation2020). Addition of biochar to sandy soils has also been described to improve soil physical, biological and chemical characteristics by reducing N2O emission and influencing soil hydraulic conductivity, thus enhancing the agricultural productivity (Paymaneh et al., Citation2018; Singh et al., Citation2010). Hence, use of organic amendments (e.g. sugarcane press-mud and vegetable compost) and biochar as a source of continuous delivery of nutrients to chickpea in sandy soils is of interest.
Although biochar is an effective and sustainable approach to improve the physio-chemical characteristics, nutrient status of soils and crop growth, still information on potential of biochar produced from cotton-sticks loaded with Fe/Zn in enhancing chickpea production in sandy soils is rather elusive. It was hypothesized that Fe/Zn-loaded cotton-sticks biochar and organic amendments may enhance the chickpea production by improving the soil quality and nutritional profile of coarse-textured sandy soils. Specifically, the current study aimed whether organic amendments and Fe/Zn-loaded biochar produced from cotton-sticks improved the ionic uptake, chlorophyll content and soil chemical properties to increase the chickpea growth and production in sandy soils.
2. Materials and methods
2.1. Biochar production
Cotton sticks were collected from the Research Area, MNS University of Agriculture, Multan and cut into small pieces of 2–5 inch. The cut material was then pyrolyzed at 500 ºC in a specialized biochar kiln to produce biochar (Nawaz et al., Citation2023). The biochar was then cooled by simple water spray, air-dried, and ground to make it suitable for further use. Further, the raw image of cotton sticks and biochar is presented in Supplementary Figure S1.
2.2. Biochar and organic amendments characterization
Cotton-sticks biochar, sugarcane press-mud, and vegetable compost were analyzed for chemical and nutritional properties using the following methods.
The electrical conductivity (EC) and pH was estimated by mixing it in distilled water using 1:10 (w/v) suspension ratio.
For measuring the cation exchange capacity (CEC) of the cotton-sticks biochar, sugarcane press-mud and vegetable compost, modified NH4-acetate compulsory displacement method was used (Gaskin et al., Citation2008).
The cotton-sticks biochar and organic amendments (sugarcane press-mud and vegetable compost) samples (0.1 g) were digested in concentrated H2SO4 (5 mL) for the determination of N, P, and K (Wolf, Citation1982). After overnight incubation, H2O2 (0.5 mL, 35%) was poured in digestion tube and heated at 330 °C in a digestion block for 30 min until become colorless. The volume of the mixture was adjusted to 50 mL, filtered and further used for estimating the N, P, and K contents using ICP-OES, Optima 2100-DV Perkin-Elmer, USA). The chemical and nutrient characteristics of cotton-sticks biochar, sugarcane press-mud, and vegetable compost are described in Table .
Table 1. Chemical characteristics and nutrient analysis of cotton-sticks biochar (CB), sugarcane press-mud (SPM) and vegetable compost (VC)
Furthermore, cotton-sticks biochar was analyzed for external surface area (8.02 m2 g−1), total pore volume (0.02 cm3 g−1), and average pore diameter (7.90 nm) using the Brunauer-Emmett-Teller (BET) technique at relative pressure P/P0. Nitrogen adsorption-desorption isotherms data were recorded with Autosorb-6iSA, Quanta chrome Instruments, USA (Figure ). The surface morphology of cotton-sticks biochar was determined through SEM. The SEM image of cotton-sticks biochar at 2.00 K X magnification to examine surface morphology is shown in Figure . The surface composition was determined with energy dispersive spectroscopy (EDS) analysis (GeminiSEM 500, Carl Zeiss, Germany) (Figure ).
2.3. Seed planting and experimental design
Uniform and healthy seeds of two chickpea varieties were collected from Ayub Agricultural Research Institute, Faisalabad. The seeds were disinfected with NaOCl (sodium hypochlorite, 10%) solution for 5 min, rinsed thoroughly and air-dried to attain the original moisture. The air-dried, well-mixed, and sieved sandy soil was filled in each pot (10 kg). The soil used in the experiment was collected from Chenab river side District Multan. Subsamples of the soil were analyzed for physical and chemical characteristics (Table ).
Table 2. Physio-chemical characteristics of the experimental soil
Seeds of both chickpea varieties (5/pot) were sown at the top soil layer of 5 cm in earthen pots having 25 cm height and 20 cm diameter. Nitrogen (N), phosphorus (P) and potassium (K) nutrient demands were confronted by applying urea at 32 kg ha−1, DAP at 84 kg ha−1 and sulfate of potash at 30 kg ha−1 fertilizers, corresponding to (160 mg), (420 mg), and (150 mg), respectively, for each pot. All respective fertilizer rates were thoroughly mixed to top soil layer (0–15 cm) during filling of each pot.
Before potting, cotton-sticks biochar, sugarcane press-mud, and vegetable compost were thoroughly mixed in the soil at 10 and 15 Mg ha−1 corresponding to 50 and 75 g/pot, respectively, along with the control (no addition). Cotton-sticks biochar at 10 and 15 Mg ha−1 also loaded with iron (Fe) and zinc (Zn) by dipping in the solution (0.03%) until the biochar was completely soaked. All pots were arranged under natural light and temperature conditions in a wire house (Research Area, MNS University of Agriculture, Multan) and protected from rain during the whole growth period. The soil moisture was kept at about 100% FC (field capacity) level by frequent irrigation on daily basis to fulfil the water requirement of plants. After 1 week of germination, one healthy plant was adjusted to each pot by rogue out the less vigorous seedlings. The study comprised 18 treatments (two varieties × nine cotton-sticks biochar/sugarcane press-mud/vegetable compost treatments) and repeated thrice in CRD factorial design. Weeds were removed manually in each pot when found necessary. The experiment was ended after 145 days of sowing for estimating the growth, nutrient contents, and yield-related traits.
2.4. Determination of NPK contents
Well-dried ground sample of each root, shoot, and grains (0.1 g) were acid digested (H2SO4, 5 mL) to determine the N, P, and K contents (Wolf, Citation1982). The digestion tubes containing plant sample and 35% H2O2 (0.5 mL) were heated at 330 °C for 30 min until becoming colorless. The mixture was then adjusted to 50 mL to determine the nutrient contents. The N, P, and K contents were measured using Kjeldahl appratus, spectrophotometer, and flame photometer, respectively (Bremner, Citation1965; Jones et al., Citation1991; Malavolta et al., Citation1997).
2.5. Estimation of chlorophyll content
At flowering stage, the chlorophyll content was determined by chlorophyll meter SPAD-502Plus (Konica Minolta Inc. Japan).
2.6. Measurement of growth and yield attributes
One plant in each pot was harvested manually and determined for shoot and root lengths with a meter rod. The cut plant was separated into root and shoot portions and measured for fresh weight. The root and shoot segments were then oven-dried separately (POL-EKO APARATURA, Model SLN 32, Poland) for 72 h at 65 °C to record the average dry weights. The number of branches and pods in each treatment was counted manually. 100-grains were selected randomly, counted, and then weight was measured using electric balance. The seeds collected from each plant were weighted to estimate the grain yield.
2.7. Post-harvest analysis of soil
After harvesting of chickpea plants, subsamples of the soil from each treatment were characterized for electrical conductivity, pH, and inorganic nutrients following the method of Jackson (Citation1962), whereas organic matter content was determined using the procedure of Moodie and McGreery (Citation1959).
2.8. Statistical analysis
Fisher’s analysis of variance (ANOVA) technique for each trait was employed using statistical program (STATISTIX 8.1) to test the significance of varieties, soil amendments, and their interaction treatments. Least significant difference (LSD) test at 5% probability level was used to compare the differences among treatments. A correlation analysis was done using R package to assess the association among different traits. Biplot between PC1 and PC2 was drawn using fviz_pca_biplot function.
3. Results
3.1. Ionic contents
Cotton-stick biochar and organic amendments supply in sandy soil caused a variability in ionic uptake in root, shoot and grain portions of two chickpea varieties as illustrated in Supplementary Table S1. Application of cotton-sticks biochar at 15 Mg ha−1 loaded with Fe/Zn significantly increased the root and shoot nitrogen contents (2.5 and 3.1%), respectively, in var. Noor-2019 than var. Bittal-2016. Soil amended with sugarcane press-mud at 10 and 15 Mg ha−1 provided poor response in increasing root and shoot nitrogen contents in both varieties, i.e. Noor-2019 than var. Bittal-2016 as illustrated in Figure . The highest increase in grain nitrogen content (3.9 and 4.0%) was recorded in var. Noor-2019 and var. Bittal-2016, respectively, for cotton-sticks biochar at 15 Mg ha−1 loaded with Fe/Zn and vegetable compost at 15 Mg ha−1 amendments. In contrast, sugarcane press-mud at 10 Mg ha−1 provided minimum grain nitrogen content (3.1 and 3.0%) for var. Noor-2019 and var. Bittal-2016, respectively, as compared to no amendment (2.7%) as illustrated in Figure .
Figure 3. Effect of cotton-stick biochar and organic amendments on root N (a), shoot N (b), grain N (c), root P (d), shoot P (e), grain P (f), root K (g), shoot K (h), and grain K (i) content of two chickpea varieties. The error bars are mean ± S.E. CK, control; CSB, cotton-sticks biochar; SPM, sugarcane press-mud; VC, vegetable compost.
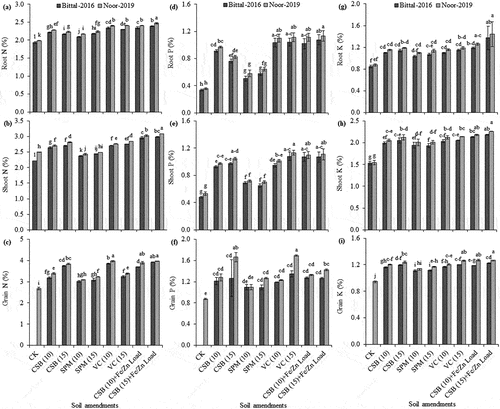
Soil amended with cotton-sticks biochar at 15 Mg ha−1 loaded with Fe/Zn exhibited a maximum increase root phosphorus content (1.08 and 1.13%) for var. Bittal-2016 and var. Noor-2019, respectively, than control (no amendment) as illustrated in Figure . Likewise, a notable increase in shoot phosphorus (1.10%) was recorded for both varieties (i.e. Noor-2019 and Bittal-2016) with cotton-sticks biochar at 10 and 15 Mg ha−1 loaded with Fe/Zn, and vegetable compost at 15 Mg ha−1 amendments in contrast to control (0.5%). The minimum increase in shoot phosphorus content (0.7%) for Noor-2019 and Bittal-2016 varieties was revealed with sugarcane press-mud at 10 and 15 Mg ha−1 as presented in Figure . Soil mixed with cotton-sticks biochar and vegetable compost at 15 Mg ha−1 significantly improved the grain phosphorus content by 1.3 and 1.7% for var. Bittal-2016 and var. Noor-2019, respectively, than no amendment addition as described in Figure .
The potassium contents in root, shoot, and grain (1.37, 2.19 and 1.22% for var. Bittal-2016 vs 1.44, 2.26 and 1.27% for var. Noor-2019), respectively, were increased remarkably in plants subjected to cotton-sticks biochar at 15 Mg ha−1 loaded with Fe/Zn nutrients than control (no amendment). Soil mixed with sugarcane press-mud depicted a minimum increase in root, shoot, and grain potassium contents (1.04, 1.94 and 1.11% for var. Bittal-2016 vs 1.10, 2.01 and 1.14% for var. Noor-2019), respectively, as compared to control as illustrated in Figure .
3.2. Chlorophyll content
Addition of cotton-stick biochar or organic amendments significantly increased the chlorophyll content in plants as illustrated in Supp. Table ; however, a maximum increase (40.17 for var. Bittal-2016 vs 48.43 for var. Noor-2019) was recorded in plants grown in soil treated with cotton-sticks biochar at 15 Mg ha−1 loaded with Fe/Zn nutrients than control (no amendment). Similarly, var. Noor-2019 treated with sugarcane press-mud at 10 Mg ha−1 also exhibited a marked increase in chlorophyll content by 47.10, whereas var. Bittal-2016 had minimum chlorophyll contents (31.40) when supplemented with sugarcane press-mud at 10 Mg ha−1 as illustrated in Figure .
3.3. Growth traits
Organic amendments and cotton-sticks biochar remarkably increased the growth traits of chickpea as illustrated in Supp. Table . Soil amended with vegetable compost at 10 Mg ha−1 followed by cotton-sticks biochar at 15 Mg ha−1 loaded with Fe/Zn nutrients exhibited a highest increase in shoot length by 43.2 cm for var. Noor-2019, whereas addition of sugarcane press-mud at 15 Mg ha−1 to sandy soil caused a maximum increase in shoot length by 38.9 cm for var. Bittal-2016 in contrast to the control (no amendment) as illustrated in Figure . Similarly, plants raised in soil supplemented with vegetable compost at 10 Mg ha−1 depicted a highest increase in root length by 25.8 cm for var. Noor-2019, whereas a marked increase in root length by 22.4 cm was recorded for var. Bittal-2016 with higher rate of 15 Mg ha−1 than no supplement addition. A remarkable decline in root length (10.9 cm for var. Bittal-2016 vs 11.7 cm for var. Noor-2019) was recorded in soil amended with sugarcane press-mud at 10 Mg ha−1 as illustrated in Figure . Plants of var. Bittal-2016 and var. Noor-2019 exhibited a maximum increase in number of branches per plant by 17.7 and 15.7, respectively, when grown in soil treated with sugarcane press-mud at 10 Mg ha−1 as compared to control (no amendment). A notable reduction in number of branches per plant were observed with addition of cotton-sticks biochar at 10 and 15 Mg ha−1 as illustrated in Figure .
Figure 5. Effect of cotton-stick biochar and organic amendments on shoot length (a), root length (b), number of branches (c), root fresh weight (d), root dry weight (e), and shoot dry weight (f) of two chickpea varieties. The error bars are mean ± S.E. CK, control; CSB, cotton-sticks biochar; SPM, sugarcane press-mud; VC, vegetable compost.
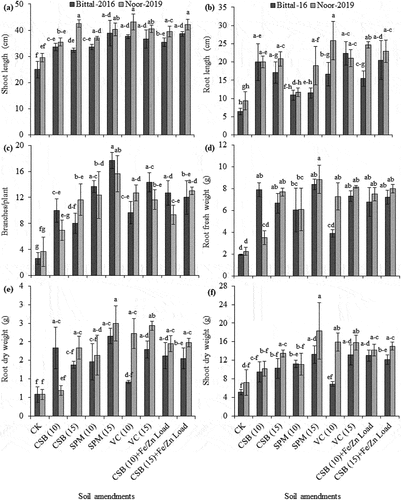
Soil supplemented with sugarcane press-mud at 15 Mg ha−1 followed by vegetable compost at 15 Mg ha−1 and cotton-sticks biochar at 15 Mg ha−1 plus loaded with Fe/Zn presented a highest increase in root fresh weight by 8.8, 8.2 and 8.0 g, respectively, for var. Noor-2019 than control (no amendment). Likewise, a marked increase in root fresh weight by 8.4 g was observed with sugarcane press-mud at 15 Mg ha−1 followed by cotton-sticks biochar at 10 Mg ha−1 for var. Bittal-2016 over no amendment as presented in Figure . A considerable increase in root and shoot dry weights (2.5 and 18.3 g for var. Noor-2019 vs 2.2 and 13.2 g for var. Bittal-2016), respectively, was exhibited with sugarcane press-mud at 15 Mg ha−1 over no amendment as described in Figure .
3.4. Yield and yield components
A diverse variability was exhibited in yield and yield-related traits of two chickpea varieties as illustrated in Supp. Table . The soil treated with vegetable compost at 10 Mg ha−1 followed by alone cotton-sticks biochar at 15 Mg ha−1 and cotton-sticks biochar at 15 Mg ha−1 loaded with Fe/Zn remarkably increased the pods per plant by 34, 29 and 27.3 for var. Noor-2019, respectively, as compared to no amendment. In contrast, addition of cotton-sticks biochar at 15 Mg ha−1 loaded with Fe/Zn markedly increased the pods per plant by 33.7 for var. Bittal-2016. Similar trend was recorded with cotton-sticks biochar at 15 Mg ha−1 loaded with Fe/Zn for number of pods per plant. Plants raised in soil treated without any amendment depicted no pods per plant for both var. Noor-2019 and var. Bittal-2016 as illustrated in Figure . A noticeable increase in 100-grain weight was observed with addition of cotton-sticks biochar and organic amendments; however, maximum increase by 32.7 g was exhibited for var. Noor-2019 with vegetable compost at 10 Mg ha−1 over control (no amendment addition). Contrarily, 100-grain weight was recorded higher by 24.9 and 24.4 g for var. Bittal-2016 with sugarcane press-mud at 15 Mg ha−1 and cotton-sticks biochar at 10 Mg ha−1, respectively, over no amendment as illustrated in Figure . Plants grown in soil mixed with organic amendment of vegetable compost at 10 Mg ha−1 described a remarkable increase in grain yield by 25.9 g for var. Noor-2019 than control (no amendment). A substantial increase in grain yield by 20.2 g was depicted for var. Bittal-2016 with amended soil of cotton-sticks biochar at 15 Mg ha−1 loaded with Fe/Zn as illustrated in Figure .
3.5. Correlation of nutrient and chlorophyll content with growth and yield attributes
Root nitrogen, and root, shoot, and grain potassium contents were positively correlated with chlorophyll content. A positive relationship of ionic uptake (e.g. nitrogen, phosphorus, and potassium) was revealed with growth traits, pods per plant and grain yield of chickpea. Chlorophyll content was positively correlated with shoot length, number of branches per plant, root fresh weight, dry weights for root and shoot, and 100-grain weight. Pods per plant and 100-grain weight had strong and positive correlation with grain yield subjected to cotton-sticks biochar and organic amendments as illustrated in Figure .
Figure 7. Correlation coefficients (r) indicating the association among nutrient contents, chlorophyll, growth and yield attributes of two chickpea varieties following cotton-stick biochar and organic amendments. The change in color represents the significant differences at p < 0.05. SL, shoot length; RL, root length, B/P, number of branches per plant; RFW, root fresh weight; RDW, root dry weight; SDW, shoot dry weight; P/P, pods per plant; 100-GW, 100-grain weight; GY, grain yield.
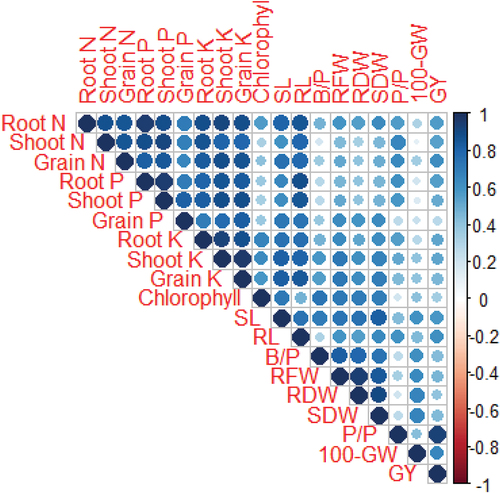
3.6. Principal component analysis of nutrient and chlorophyll content with growth and yield attributes
PCA was conducted among nutrient content, chlorophyll, growth, and yield attributes of two chickpea varieties to visualize the association following cotton-stick biochar and organic amendments. A variability of 56.4% and 15.2% was assessed between Dim1 and Dim2, respectively, that exhibited a close association of GY with root and shoot N, P, K, and chlorophyll content while negative to grain P and P/P as presented in Figure .
Figure 8. Biplot of combined principal component analysis (PCA) of nutrient and chlorophyll content with growth and yield attributes of two chickpea varieties following cotton-stick biochar and organic amendments. SL, shoot length; RL, root length, B/P, number of branches per plant; RFW, root fresh weight; RDW, root dry weight; SDW, shoot dry weight; P/P, pods per plant; 100-GW, 100-grain weight; GY, grain yield. T0, CK; T1, cotton-sticks biochar at 10 Mg ha−1; T2, cotton-sticks biochar at 15 Mg ha−1; T3, sugarcane press-mud at 10 Mg ha−1; T4, sugarcane press-mud at 15 Mg ha−1; T5, vegetable compost at 10 Mg ha−1; T6, vegetable compost at 15 Mg ha−1; T7, cotton-sticks biochar at 10 Mg ha−1 + Fe/Zn Load; T8, cotton-sticks biochar at 15 Mg ha−1 + Fe/Zn Load.
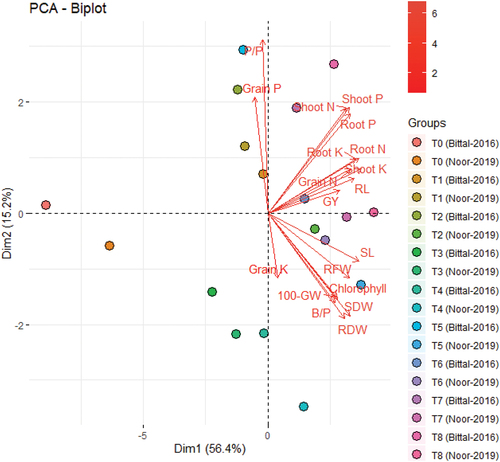
3.7. Soil chemical properties
Soil amended with cotton-sticks biochar at 10 Mg ha−1 loaded with Fe/Zn depicted a highest increase in soil EC by 2.19 and 2.12 dS m−1 for var. Bittal-2016 and var. Noor-2019, respectively, as compared to vegetable compost and sugarcane press-mud amendments. Similarly, cotton-sticks biochar at 10 Mg ha−1 loaded with Fe/Zn showed maximum increase in soil pH by 8.4 for var. Noor-2019; whereas sugarcane press-mud at 15 Mg ha−1 presented a marked increase in soil pH by 8.5 for var. Bittal-2016 as illustrated in Table . Soil organic matter and nitrogen contents was increased considerably by 0.72 and 0.21%, respectively, for var. Bittal-2016 with cotton-sticks biochar at 15 Mg ha−1, whereas cotton-sticks biochar at 10 Mg ha−1 loaded with Fe/Zn exhibited a highest increase in soil organic matter and nitrogen by 0.67 and 0.20%, respectively, for var. Noor-2019 in parallel to control (no amendment addition). Soil mixed with vegetable compost at 15 Mg ha−1 depicted a notable increase in soil phosphorus (10.7 mg kg−1 for var. Noor-2019 vs 10.4 mg kg−1 for var. Bittal-2016) than no amendment addition. A noticeable increase in soil potassium by 204.7 mg kg−1 for var. Bittal-2016 was recorded with vegetable compost at 15 Mg ha−1; however, alone cotton-sticks biochar at 10 Mg ha−1 showed maximum soil potassium content by 199 mg kg−1 for var. Noor-2019 as illustrated in Table .
Table 3. Effect of cotton-stick biochar and organic amendments on chemical properties of sandy soil cultivated with two chickpea varieties
4. Discussion
Sandy soils are poor in fertility, organic matter, and water retention that poses serious threats to crop productivity (Case et al., Citation2012; Laghari et al., Citation2015; Van Asperen et al., Citation2014). Cultivation of food crops, in particular, chickpea in sandy soils have serious limitations of water and nutrient uptake for its optimum development (Reverchon et al., Citation2014). Previously, biochar and organic amendments addition to poor-textured soils have been reported to improve the soil structure and chemical properties (e.g. pH, EC, CEC, organic matter, and nutrient profile), thus aggravating the crop yield and growth (Mawof et al., Citation2021). However, studies related to Fe/Zn-loaded biochar produced from cotton-sticks and organic materials as more stable and sustainable amendments in enhancing the chickpea productivity in coarse textured sandy soils are of interest.
4.1. Nutrient contents
Nutrient acquisition (e.g. N, P, and K) in root, shoot, and grains of chickpea was facilitated by cotton-sticks biochar loaded with Fe/Zn and other organic materials in current results. An increase in nutrient absorption by chickpea plants in sandy soils might be linked with distinct properties of biochar including high specific surface area, cation exchange capacity (CEC), and water holding capacity (de Jesus Duarte et al., Citation2019; Fahad et al., Citation2015). In recent studies, it is well illustrated that biochar amendment has a key function to increase the water holding capacity of sandy soils (Bruun et al., Citation2014; Danish et al., Citation2020; Rogovska et al., Citation2014), thereby ensuring higher availability of nutrients to plants. In fact, Fe/Zn-loaded cotton-sticks biochar addition to sandy soils allows to increase the soil biological as well as chemical characteristics, which in turn enhances the availability of nutrients (Gul & Whalen, Citation2016; Gul et al., Citation2014). Positive effects of Fe/Zn loading on biochar for higher nutrient uptake are more evident in present findings in contrast to previous reports might be due to the fact that Fe could actively adsorb higher content of nutrients at the root surfaces and ultimately increasing the nutrient uptake in plants (Zhang et al., (Citation1998). The beneficial responses of biochar application on root development and morphology for higher nutrient absorption are clearly evident in previous reports of Zhang et al. (Citation2019) & Liu et al. (Citation2021). In present results, an increase in nutrient absorption with Fe/Zn-enriched cotton-sticks biochar might be attributed to the fact that biochar addition in sandy soils may reduce the bulk density of the top soil and improve the soil porosity, consequently increasing the roots ability to access more rhizospheric area for nutrient uptake. Kim et al. (Citation2011) claimed that nutrient availability in soils depends on organic matter content and specific nutrient retention capacity of soils. Soils with such poor chemical characteristics provide less time for nutrient sorption, in particular, Fe and Zn, thereby causing excessive leaching of ions. In support to current results, Fe/Zn-loaded biochar supply is accredited to increase the organic carbon and nutrient sorption time, thus suggesting its efficient role in improving the availability of nutrients (e.g. nitrogen, phosphorus, potassium, iron, and zinc) to plant roots in coarse-textured sandy soils (Amin & Mihoub, Citation2021; Chandrika et al., Citation2022). Furthermore, a notable supremacy of Fe/Zn-enriched cotton-sticks biochar for an increased availability of nutrients to plant roots in sandy soils might be attributed to its key role in mineralization of nutrients and enhanced roots’ vitality for nutrient absorption (Hameeda et al., Citation2019; Nawaz et al., Citation2023). In previous reports, Abbas et al. (Citation2009) and Paramesh et al. (Citation2020) indicated that Zn nutrient in competition with Fe may reduce the Fe adsorption on soil particles, consequently reducing the Fe uptake and translocation from plant roots to shoot. In contrast, Fe/Zn-loaded cotton-sticks biochar increased the accumulation of nutrients in different chickpea plant parts that could presumably due to increased organic matter content, thus resultantly leading to higher nutrient availability (Iratkar et al., Citation2014). In reliability of our results, Fe/Zn-loaded biochar application also provides a safe environment for microbial growth liable for improving soil health and root system for higher nutrient uptake (Ducey et al., Citation2013). It has been well described that microbial taxa also contain nitrogenase genes for promoting nitrogen assimilation in chickpea grains (Batool et al., Citation2021). As true in the present study, pyrolysis of the cotton-sticks biochar caused complete destruction of the uneven surfaces and developed pores on its surfaces due to release of organics and volatiles, ultimately resulting in higher nutrient adsorption (Arif et al., Citation2022; Dawar et al., Citation2021).
4.2. Chlorophyll content
Adequate availability of chlorophyll content in plants is directly linked with crop productivity. The current findings showed the positive effects of Fe/Zn-loaded cotton-sticks biochar on overall productivity of chickpea might be due to increased chlorophyll content. A marked increase in chlorophyll content with cotton-sticks biochar has been corroborated in previous reports of Nadeem et al. (Citation2017) & Nawaz et al. (Citation2023). A substantial increase in chlorophyll content with Fe/Zn-loaded cotton-sticks biochar amendment is implicated to dominant effects of Fe and Zn released from the biochar that involved in the production of δ-damino laveulinic acid and glycine, further stimulating the chlorophyll synthesis (Bashir et al., Citation2020; Miller et al., Citation1982). In recent reports, it is suggested that chlorophyll contents were decreased in Fe or Zn-deficient plants. The possible reasons of reducing chlorophyll pigments in Fe/Zn-deficient plants might be their deficiency effects on production process of chlorophylls. In current results, a prominent increase in chlorophyll content in Fe/Zn-loaded cotton-sticks biochar amended plants further explained the key involvement of Fe in oxidation and reduction processes of cytochrome liable for chlorophyll synthesis (Marschner, Citation2011). Likewise, Zn has key function in enzymatic processes and regulating the electron transport system, while its deficiency can increase the free radicals to damage the LHC proteins and thylakoid membranes, hence aggravating chlorophyll degradation in plants (Roosta et al., Citation2018).
4.3. Growth traits
Biochar and organic amendments are essential to enhance the growth performance of plants in poor-textured sandy soils (Sukartono, Citation2011). In the present study, application of Fe/Zn-loaded cotton-sticks biochar and organic amendments in sandy soils increased the root shoot lengths, fresh and dry weights by means of retaining higher water and nutrient retention ability (Bamagoos et al., Citation2021). A substantial increase in shoot and root growth by Fe/Zn-enriched cotton-sticks biochar could be due to its potential role in modifying the soil conditions favorable for plant growth. Previously, similar findings were also claimed by Zimmerman (Citation2010); Lentz and Ippolito (Citation2012) who viewed that biochar is a rich source of carbon having enough quantities of plant nutrients, however, can make nutrient deficient soils more fertile. In the present study, Fe/Zn-loaded cotton-sticks biochar indicated a significant increase in root and shoot lengths and fresh weight that might be attributed to active role of Fe and Zn in the biosynthesis of chlorophyll and proteins, DNA, photosynthesis and respiration. Previous studies also described that Fe and Zn actively involves in the maintenance of chloroplast structure, translocation of photosynthates, proliferation of roots and gene expression of related hormones (e.g. cytokinins and ABA), thereby increasing nutrient uptake and growth of plants (Semida et al., Citation2021; Shrivastava et al., Citation2018). A marked decline in growth traits of chickpea supplemented without Fe/Zn-enriched cotton-sticks biochar might be related to destruction of thylakoid membrane, quinone, plastoquinone, and electron transport system due to acute deficiency of Fe and Zn (Roosta et al., Citation2018). It is more likely that an improvement in chemical properties of coarse-textured soils, mineralization, and availability of nutrients to plant roots are accompanied by the addition of organic amendments and Fe/Zn-loaded cotton-sticks biochar, thus suggesting the possible reasons of increased plant biomass (Hameeda et al., Citation2019; Irfan et al., Citation2022; Mawof et al., Citation2021). It was also claimed that plants raised in soils amended with biochar improved the water retention capacity of soils and may boost the rhizospheric nutrient cycling, and ultimately plant growth (Bruun et al., Citation2014; Mihoub et al., Citation2022).
4.4. Yield and yield attributes
In the present study, cotton-sticks biochar loaded with Fe/Zn nutrients and compost addition to sandy soils helped to increase the pods per plant, 100-grain weight, and yield of both chickpea varieties (Bittal-2016 and Noor-2019), which could possibly be due to improved soil structure, aeration, nutrient retention, and microbial population (Mihoub et al., Citation2022). An increase in biological activity of microbes for nutrient fixation and solubilization in amended soils of cotton-sticks biochar could explain the greater nutrients availability to plant roots, hence aggravating root growth and grain yield attributes. It has been claimed in previous reports that losses to nitrate leaching could be reduced up to 75% with biochar addition. The improvement in chickpea yield with vegetable compost and Fe/Zn-loaded cotton-sticks biochar might be due to the key role of nutrients (e.g. N, P, K, Fe, Zn) released from biochar and organic amendments in enzymes metabolism, chlorophyll production, and stomatal conductance which further resulted in higher photosynthesis (Wali et al., Citation2020). Recently, Ibad Ullah et al. (Citation2022) also concluded that integrated use of biochar and nutrients significantly increased the grain yield and fresh biomass of soybean. Similar findings were also validated in soybean (Correa et al., Citation2009), green pepper (Yao et al., Citation2015), wheat (Dawar et al., Citation2021), and maize (Irfan et al., Citation2021), suggesting improved biomass and grain yields due to better colonization of beneficial microorganisms within the plant rhizosphere for retaining immobile water and nutrients within microspores by increasing metric potential. In agreement to current findings, positive responses of biochar and organic amendments on increased root shoot ratio, pods per plant, and grain yield with higher water use efficiency and carbon dioxide flow to leaf mesophyll cells have previously been reported in french beans and tomatoes (Akhtar et al., Citation2014; Saxena et al., Citation2013).
A strong correlation of nutrient and chlorophyll contents with growth and yield-related traits in recent results validated that ionic uptake and chlorophyll synthesis improved the chickpea growth in sandy soils. PCA analysis also presented that enhanced chickpea growth and yield in coarse-textured soils is strongly associated with chlorophyll and nutrients contents.
4.5. Soil chemical properties
Amended soil with Fe/Zn-loaded biochar and organic amendments tends to increase soil chemical properties (e.g. soil pH, EC, organic matter, N, P and K contents) than no amendment addition. Biochar amendment has been reported to improve the chemical properties of poor-textured soils (Ahmad et al., Citation2022; Hameeda et al., Citation2019). In the present study, soil pH was higher with cotton-sticks biochar enriched with Fe/Zn nutrients making suitable environment for plants to grow especially in drylands with low organic matter content (Gul et al., Citation2015; Taghizadeh-Toosi et al., Citation2012; Zhu et al., Citation2018). Soil mixing with Fe/Zn-loaded biochar had higher EC than the soil receiving no amendment. It is expected that biochar bearing cations on its surfaces are released into the rhizosphere, therefore increasing the exchangeable cations/anions in the soil mix (Khan et al., Citation2020). Cotton-sticks biochar as an amendment contains char and organic debris that have imposed positive effects on soil health. Increasing soil organic matter with biochar amendment is related to biochar mineralization and is closely concur with the previous findings of Mawof et al. (Citation2021) & Rahi et al. (Citation2022). Biochar enriched with Fe/Zn is considered promising to improve the nutrient profile of sandy soil. In fact, biochar with small particle size characteristics is substantiated to slight increase in CN ratio that crucial for enhanced nutrient retention (de Jesus Duarte et al., Citation2019). The possible reasons for increased nitrogen content in soils with Fe/Zn-loaded cotton-sticks biochar might be due to root-persuaded mineralization of organic nitrogen, and reduced losses of nitrogen caused by leaching, volatilization, and denitrification (Taghizadeh-Toosi et al., Citation2012). It is also evident that root growth promotion is soils amended with organic and biochar amendments facilitate the growth of organic phosphorus hydrolyzing and inorganic phosphorus solubilizing microbes, hence leading to higher inorganic phosphorus content in soils. Indeed, these microorganisms may secrete the acids in soils and solubilize the precipitated phosphorus into available form (Gul & Whalen, Citation2016; Ziadi et al., Citation2014). It is also evident that biochar may contain polycyclic aromatic hydrocarbons (PAHs). Upon amending the soil with such biochar containing PAHs may get accumulate in plants and present severe human health problems. The carcinogenic behavior of PAHs mainly depends on the type of feedstock and the temperature at which pyrolysis occurs (Wali et al., Citation2020). Hence, temperature maintenance of biochar pyrolysis can make the feedstock feasible for agricultural use.
5. Conclusions
Fe/Zn-loaded cotton-sticks biochar had significant effects on nutrient uptake, chlorophyll contents, grain yield of chickpea, and soil chemical properties (e.g. electrical conductivity, soil pH, organic matter, nitrogen, phosphorus and potassium contents) of sandy soils. The nutrient accumulation, chlorophyll content, and yield traits of chickpea were improved with Fe/Zn-loaded cotton-sticks biochar at 15 Mg ha−1 and vegetable compost. Further, Fe/Zn-loaded cotton-sticks biochar improved the soil chemical properties of coarse-textured sandy soils that resulted in enhanced ionic uptake and grain yield of chickpea. Hence, Fe/Zn-loaded biochar produced from cotton sticks is an environmentally safe material and could be exploited as a resource for improving the chickpea production in sandy soils. Further studies are recommended to evaluate the long-term effects of cotton-sticks biochar loaded with Fe/Zn to improve the soil quality and economic yields of food crops in areas of poor-textured properties.
Author contributions
AM: Conceptualization and methodology; KSA: validation; SU: formal analysis and investigation; JW: data curation; MAS: writing-original draft preparation; MA & MI: writing-review and editing; HAS: supervision; TUH: project administration; IMM & HOE: funding acquisition. All authors have read and agreed to the published version of the manuscript.
Supplemental Material
Download Zip (621.6 KB)Acknowledgments
The authors would like to extend the sincere appreciation to the Researchers Supporting Project Number (RSPD2023R741), King Saud University, Riyadh, Saudi Arabia. Further, we highly acknowledge the contribution of Dr. Balal Yousaf, Associate Professor at University of Science and Technology of China, Hefei, Anhui, China for supporting us in the characterization of biochar.
Disclosure statement
No potential conflict of interest was reported by the author(s).
Data availability statement
All data is available within the manuscript and its sup-porting information
Supplementary data
Supplemental data for this article can be accessed online at https://doi.org/10.1080/23311932.2023.2265110
Additional information
Funding
References
- Abbas, G., Khan, M. Q., Jamil, M., Tahir, M., & Hussain, F. (2009). Nutrient uptake, growth and yield of wheat (Triticum aestivum) as affected by zinc application rates. International Journal of Agriculture & Biology, 11, 389–21. http://www.fspublishers.org/
- Ahmad, W., Khan, A., Zeeshan, M., Ahmad, I., Adnan, M., Fahad, S., Solaiman, Z., & Solaiman, Z. (2022). Relative efficiency of biochar particles of different sizes for immobilising heavy metals and improving soil properties. Crop and Pasture Science, 74(2), 112–120. https://doi.org/10.1071/CP20453
- Akhtar, S. S., Li, G., Andersen, M. N., & Liu, F. (2014). Biochar enhances yield and quality of tomato under reduced irrigation. Agricultural Water Management, 138, 37–44. https://doi.org/10.1016/j.agwat.2014.02.016
- Alharby, H. F., & Fahad, S. (2020). Melatonin application enhances biochar efficiency for drought tolerance in maize varieties: Modifications in physio‐biochemical machinery. Agronomy Journal, 112(4), 2826–2847. https://doi.org/10.1002/agj2.20263
- Amin, A. E., & Mihoub, A. (2021). Effect of sulfur-enriched biochar in combination with sulfur-oxidizing bacterium (Thiobacillus Spp.) on release and distribution of phosphorus in high calcareous p-fixing soils. Journal of Soil Science and Plant Nutrition, 21(3), 2041–2047. https://doi.org/10.1007/s42729-021-00500-5
- Arif, M., Liu, G., Ur Rehman, M. Z., Yousaf, B., Ahmed, R., Mian, M. M., Ashraf, A., Munir, M. A. M., Rashid, M. S., & Naeem, A. (2022). Carbon dioxide activated biochar-clay mineral composite efficiently removes ciprofloxacin from contaminated water-reveals an incubation study. Journal of Cleaner Production, 332, 130079. https://doi.org/10.1016/j.jclepro.2021.130079
- Babar, M., Munir, H. M. S., Nawaz, A., Ramzan, N., Azhar, U., Sagir, M., Tahir, M. S., Ikhlaq, A., Mubashir, M., Khoo, K. S., & Chew, K. W. (2022). Comparative study of ozonation and ozonation catalyzed by Fe-loaded biochar as catalyst to remove methylene blue from aqueous solution. Chemosphere, 307, 135738. https://doi.org/10.1016/j.chemosphere.2022.135738
- Bamagoos, A., Alharby, H., & Fahad, S. (2021). Biochar coupling with phosphorus fertilization modifies antioxidant activity, osmolyte accumulation and reactive oxygen species synthesis in the leaves and xylem sap of rice cultivars under high-temperature stress. Physiology & Molecular Biology of Plants, 27(9), 2083–2100. https://doi.org/10.1016/j.sjbs.2021.09.035
- Bar El Dadon, S., Abbo, S., & Reifen, R. (2017). Leveraging traditional crops for better nutrition and health—the case of chickpea. Trends in Food Science & Technology, 64, 39–47. https://doi.org/10.1016/j.tifs.2017.04.002
- Bashir, A., Rizwan, M., Ali, S., Adrees, M., Rehman, M. Z., & Qayyum, M. F. (2020). Effect of composted organic amendments and zinc oxide nanoparticles on growth and cadmium accumulation by wheat; a life cycle study. Environmental Science and Pollution Research, 27(19), 23926–23936. https://doi.org/10.1007/s11356-020-08739-8
- Batool, S., Asghar, H. N., Shehzad, M. A., Yasin, S., Sohaib, M., Nawaz, F., Akhtar, G., Mubeen, K., Zahir, Z. A., & Uzair, M. (2021). Zinc-solubilizing bacteria-mediated enzymatic and physiological regulations confer zinc biofortification in chickpea (Cicer arietinum L.). Journal of Soil Science and Plant Nutrition, 21(3), 2456–2471. https://doi.org/10.1007/s42729-021-00537-6
- Bremner, J. M. (1965). Total nitrogen. Methods of soil analysis: Part 2 chemical and microbiological properties. 9, 1149–1178. https://doi.org/10.2134/agronmonogr9.2.c32
- Bruun, E. W., Petersen, C. T., Hansen, E., Holm, J. K., & Hauggaard‐Nielsen, H. (2014). Biochar amendment to coarse sandy subsoil improves root growth and increases water retention. Soil Use and Management, 30(1), 109–118. https://doi.org/10.1111/sum.12102
- Case, S. D., McNamara, N. P., Reay, D. S., & Whitaker, J. (2012). The effect of biochar addition on N2O and CO2 emissions from a sandy loam soil–the role of soil aeration. Soil Biology and Biochemistry, 51, 125–134. https://doi.org/10.1016/j.soilbio.2012.03.017
- Chandrika, K. P., Qureshi, A. A., Singh, A., Sarada, C., & Gopalan, B. (2022). Fe and Zn metal nanocitrates as plant nutrients through soil application. ACS Omega, 7(49), 45481–45492. https://doi.org/10.1021/acsomega.2c06096
- Correa, O. S., Montecchia, M. S., Berti, M. F., Ferrari, M. C. F., Pucheu, N. L., Kerber, N. L., & García, A. F. (2009). Bacillus amyloliquefaciens BNM122, a potential microbial biocontrol agent applied on soybean seeds, causes a minor impact on rhizosphere and soil microbial communities. Applied Soil Ecology, 41(2), 185–194. https://doi.org/10.1016/j.apsoil.2008.10.007
- Croker, J., Poss, R., Hartmann, C., & Bhuthorndharaj, S. (2004). Effects of recycled bentonite addition on soil properties, plant growth and nutrient uptake in a tropical sandy soil. Plant and Soil, 267(1–2), 155–163. https://doi.org/10.1007/s11104-005-4641-x
- Czaban, J., & Siebielec, G. (2013). Effects of bentonite on sandy soil chemistry in a long-term plot experiment (II); effect on pH, CEC, and macro-and micronutrients. Polish Journal of Environmental Studies, 22 (6), 1669–1676.
- Danish, S., Zafar-Ul-Hye, M., Fahad, S., Saud, S., Brtnicky, M., Hammerschmiedt, T., & Datta, R. (2020). Drought stress alleviation by ACC deaminase producing Achromobacter xylosoxidans and Enterobacter cloacae, with and without timber waste biochar in maize. Sustainability, 12(15), 6286. https://doi.org/10.3390/su12156286
- Dawar, K., Fahad, S., Alam, S. S., Khan, S. A., Younis, A., Dawar, U., Datta, S., Danish, R., & Dick, R. P. (2021). Influence of variable biochar concentration on yield-scaled nitrous oxide emissions, wheat yield and nitrogen use efficiency. Scientific Reports, 11(1), 16774. https://doi.org/10.1038/s41598-021-96309-4
- Dawar, K., Fahad, S., Jahangir, M. M. R., Munir, I., Alam, S. S., Khan, S. A., Mian, I. A., Datta, R., Saud, S., Banout, J., Adnan, M., Ahmad, M. N., Khan, A., Dewil, R., Habib-Ur-Rahman, M., Ansari, M. J., & Danish, S. (2021). Biochar and urease inhibitor mitigate NH3 and N2O emissions and improve wheat yield in a urea fertilized alkaline soil. Scientific Reports, 11(1), 17413. https://doi.org/10.1038/s41598-021-96771-0
- de Jesus Duarte, S., Glaser, B., & Pellegrino Cerri, C. E. (2019). Effect of biochar particle size on physical, hydrological and chemical properties of loamy and sandy tropical soils. Agronomy, 9(4), 165. https://doi.org/10.3390/agronomy9040165
- Ducey, T. F., Ippolito, J. A., Cantrell, K. B., Novak, J. M., & Lentz, R. D. (2013). Addition of activated switchgrass biochar to an aridic subsoil increases microbial nitrogen cycling gene abundances. Applied Soil Ecology, 65, 65–72. https://doi.org/10.1016/j.apsoil.2013.01.006
- Fahad, S., Hussain, S., Saud, S., Hassan, S., Tanveer, M., Ihsan, M. Z., Shah, A. N., Ullah, A., Khan, F., Ullah, S., & Alharby, H. (2016). A combined application of biochar and phosphorus alleviates heat-induced adversities on physiological, agronomical and quality attributes of rice. Plant Physiology and Biochemistry, 103, 191–198. https://doi.org/10.1016/j.plaphy.2016.03.001
- Fahad, S., Hussain, S., Saud, S., Tanveer, M., Bajwa, A. A., Hassan, S., Shah, A. N., Ullah, A., Wu, C., Khan, F. A., Shah, F., Ullah, S., Chen, Y., & Huang, J. (2015). A biochar application protects rice pollen from high-temperature stress. Plant Physiology and Biochemistry, 96, 281–287. https://doi.org/10.1016/j.plaphy.2015.08.009
- Fatima, B., Bibi, F., Ali, M. I., Woods, J., Ahmad, M., Mubashir, M., Khan, M. S., Bokhari, A., & Khoo, K. S. (2022). Accompanying effects of sewage sludge and pine needle biochar with selected organic additives on the soil and plant variables. Waste Management, 153, 197–208. https://doi.org/10.1016/j.wasman.2022.08.016
- François, M., Lin, K. S., Rachmadona, N., & Khoo, K. S. (2023). Advancement of biochar-aided with iron chloride for contaminants removal from wastewater and biogas production: A review. Science of the Total Environment, 874, 162437. https://doi.org/10.1016/j.scitotenv.2023.162437
- Gaskin, J. W., Steiner, C., Harris, K., Das, K. C., & Bibens, B. (2008). Effect of low-temperature pyrolysis conditions on biochar for agricultural use. Transactions of the ASABE, 51(6), 2061–2069. https://doi.org/10.13031/2013.25409
- Gul, S., & Whalen, J. K. (2016). Biochemical cycling of nitrogen and phosphorus cycling in biochar-amended soils. Soil Biology and Biochemistry, 103, 1–15. https://doi.org/10.1016/j.soilbio.2016.08.001
- Gul, S., Whalen, J. K., Thomas, B. W., Sachdeva, V., & Deng, H. (2015). Physico-chemical properties and microbial responses in biochar-amended soils: Mechanisms and future directions. Agriculture, Ecosystems & Environment, 206, 46–59. https://doi.org/10.1016/j.agee.2015.03.015
- Gul, S., Winans, K. S., Leila, M., & Whalen, J. K. (2014). Sustaining soil carbon in bioenergy cropping systems of northern temperate regions. CABI Reviews, 9, 1–23. https://doi.org/10.1079/PAVSNNR20149026
- Hajarpoor, A., Soltani, A., Zeinali, E., & Sayyedi, F. (2014). Simulating climate change impacts on production of chickpea under water-limited conditions. Agriculture Science Developments, 3 (6), 209–217.
- Hameeda, G., Manzoor, G., Bano, M., Chandio, T. A., & Awan, A. A. (2019). Biochar and manure influences tomato fruit yield, heavy metal accumulation and concentration of soil nutrients under wastewater irrigation in arid climatic conditions. Cogent Food & Agriculture, 5(1), 1576406. https://doi.org/10.1080/23311932.2019.1576406
- Herawati, A., Syamsiyah, J., Baldan, S. K., & Arifin, I. (2021). Application of soil amendments as a strategy for water holding capacity in sandy soils. In IOP Conference Series: Earth and Environmental Science, 724, 012014. IOP Publishing. https://doi.org/10.1088/1755-1315/724/1/012014
- He, H., Wu, M., Su, R., Zhang, Z., Chang, C., Peng, Q., Dong, Z., Pang, J., & Lambers, H. (2021). Strong phosphorus (P)-zinc (Zn) interactions in a calcareous soil-alfalfa system suggest that rational P fertilization should be considered for Zn biofortification on Zn-deficient soils and phytoremediation of Zn-contaminated soils. Plant and Soil, 461(1–2), 119–134. https://doi.org/10.1007/s11104-020-04793-w
- Iratkar, A. G., Giri, J. D., Kadam, M. M., Giri, J. N., & Dabhade, M. B. (2014). Distribution of DTPA extractable micronutrients and their relationship with soil properties in soil of parsori watershed of Nagpur district of Maharashtra. Asian Journal of Soil Science, 9, 297–299. http://www.researchjournal.co.in/onli
- Irfan, M., Dawar, K., Fahad, S., Mehmood, I., Alamri, S., Siddiqui, M. H., Saud, S., Khattak, J. Z. K., Ali, S., Hassan, S., & Nawaz, T. (2022). Exploring the potential effect of achnatherum splendens L.–derived biochar treated with phosphoric acid on bioavailability of cadmium and wheat growth in contaminated soil. Environmental Science and Pollution Research, 29(25), 37676–37684. https://doi.org/10.1007/s11356-021-17950-0
- Irfan, M., Mudassir, M., Khan, M. J., Dawar, K. M., Muhammad, D., Mian, I. A., Ali, W., Fahad, S., Saud, S., Hayat, Z., Nawaz, T., Khan, S. A., Alam, S., Ali, B., Banout, J., Ahmed, S., Mubeen, S., Danish, S. … Elgorban, A. M. (2021). Heavy metals immobilization and improvement in maize (Zea mays L.) growth amended with biochar and compost. Scientific Reports, 11(1), 18416. https://doi.org/10.1038/s41598-021-97525-8
- Jackson, M. L. (1962). Soil chemical analysis, constable and co. Ltd. London. ( 497).
- Jones, J. B., Jr., Wolf, B., & Mills, H. A. (1991). Plant analysis handbook: A practical sampling, preparation, analysis, and interpretation guide. Micro-Macro Inc.
- Khan, M. I., Afzal, M. J., Bashir, S., Naveed, M., Anum, S., Cheema, S. A., Wakeel, A., Sanaullah, M., Ali, M. H., & Chen, Z. (2021). Improving nutrient uptake, growth, yield and protein content in chickpea by the co-addition of phosphorus fertilizers, organic manures, and bacillus sp. Mn-54. Agronomy, 11(3), 436. https://doi.org/10.3390/agronomy11030436
- Khan, S., Shah, Z., Mian, I. A., Dawar, K., Tariq, M., Khan, B., Mussarat, M., Amin, H., Ismail, M., Ali, S., & Shah, T. (2020). Soil fertility, N2 fixation and yield of chickpea as influenced by long-term biochar application under mung–chickpea cropping system. Sustainability, 12(21), 9008. https://doi.org/10.3390/su12219008
- Kim, H. Y., Lim, S. S., Kwak, J. H., Lee, S., Lee, D. S., Hao, X., Yoon, K. S., & Choi, W. J. (2011). Soil and compost type affect phosphorus leaching from inceptisol, ultisol, and andisol in a column experiment. Communication in Soil Science and Plant Analysis, 42(18), 2188–2199. https://doi.org/10.1080/00103624.2011.602450
- Laghari, M., Mirjat, M. S., Hu, Z., Fazal, S., Xiao, B., Hu, M., Chen, Z., & Guo, D. (2015). Effects of biochar application rate on sandy desert soil properties and sorghum growth. Catena, 135, 313–320. https://doi.org/10.1016/j.catena.2015.08.013
- Lentz, R. D., & Ippolito, J. A. (2012). Biochar and manure affect calcareous soil and corn silage nutrient concentrations and uptake. Journal of Environmental Quality, 41(4), 1033–1043. https://doi.org/10.2134/jeq2011.0126
- Liu, B., Li, H., Li, H., Zhang, A., & Rengel, Z. (2021). Long‐term biochar application promotes rice productivity by regulating root dynamic development and reducing nitrogen leaching. Global Change Biology Bioenergy, 13, 257–268. https://doi.org/10.1111/gcbb.12766
- Lusiba, S., Odhiambo, J., & Ogola, J. (2018). Growth, yield and water use efficiency of chickpea (Cicer Arietinum): Response to biochar and phosphorus fertilizer application. Archives of Agronomy and Soil Science, 64(6), 819–833. https://doi.org/10.1080/03650340.2017.1407027
- Mahmoud, A. W. M., Ayad, A. A., Abdel-Aziz, H. S., Williams, L. L., El-Shazoly, R. M., Abdel-Wahab, A., & Abdeldaym, E. A. (2022). Foliar application of different iron sources improves morpho-physiological traits and nutritional quality of broad bean grown in sandy soil. Plants, 11(19), 2599. https://doi.org/10.3390/plants11192599
- Malavolta, E., Vitti, G. C., & Oliveira, S. A. (1997). Assessment of the nutritional status of plants: Principles and applications (2nd ed.).ABPPF.
- Marschner, H. (2011). Marschner’s mineral nutrition of higher plants. Academic Press.
- Mawof, A., Prasher, S., Bayen, S., & Nzediegwu, C. (2021). Effects of biochar and biochar-compost mix as soil amendments on soil quality and yield of potatoes irrigated with wastewater. Journal of Soil Science and Plant Nutrition, 21(4), 2600–2612. https://doi.org/10.1007/s42729-021-00549-2
- Mihoub, A., Amin, A. E., Motaghian, H. R., Saeed, M. F., & Naeem, A. (2022). Citric Acid (CA)–Modified biochar improved available phosphorus concentration and its half-life in a P-Fertilized calcareous Sandy Soil. Journal of Soil Science and Plant Nutrition, 22(1), 465–474. https://doi.org/10.1007/s42729-021-00662-2
- Miller, G. W., Denney, A., Pushnik, J., & Ming-Ho, Y. (1982). The formation of delta-aminolevulinate a precursor of chlorophyll in barley and the role of iron. Journal of Plant Nutrition, 5(4–7), 289–300. https://doi.org/10.1080/01904168209362958
- Moodie, C. D., & McGreery, R. A. (1959). Laboratory manual for soil fertility development in corn (Zea mays L.) and subsequent grain yield. Crop Science, 11, 368–372.
- Mustafa, G., Shehzad, M. A., Tahir, M. H. N., Nawaz, F., Akhtar, G., Bashir, M. A., & Ghaffar, A. (2022). Pretreatment with chitosan arbitrates physiological processes and antioxidant defense system to increase drought tolerance in alfalfa (Medicago sativa L.). Journal of Soil Science and Plant Nutrition, 22(2), 2169–2186. https://doi.org/10.1007/s42729-022-00801-3
- Nadeem, S. M., Imran, M., Naveed, M., Khan, M. Y., Ahmad, M., Zahir, Z. A., & Crowley, D. E. (2017). Synergistic use of biochar, compost and plant growth‐promoting rhizobacteria for enhancing cucumber growth under water deficit conditions. Journal of the Science of Food and Agriculture, 97(15), 5139–5145. https://doi.org/10.1002/jsfa.8393
- Nawaz, F., Rafeeq, R., Majeed, S., Ismail, M. S., Ahsan, M., Ahmad, K. S., Akram, A., & Haider, G. (2023). Biochar amendment in combination with endophytic bacteria stimulates photosynthetic activity and antioxidant enzymes to improve soybean yield under drought stress. Journal of Soil Science and Plant Nutrition, 23(1), 746–760. https://doi.org/10.1007/s42729-022-01079-1
- Ogola, J. B. O., Macil, P. J., & Odhiambo, J. J. O. (2021). Biochar application and rhizobium inoculation increased intercepted radiation and yield of chickpea in contrasting soil types. International Journal of Plant Production, 15(2), 219–229. https://doi.org/10.1007/s42106-021-00141-9
- Osman, K. T. (2018). Sandy Soils. In Management of soil problems (pp. 37–65). Springer International Publishing. https://doi.org/10.1007/978-3-319-75527-4_3
- Pal, V., Singh, G., & Dhaliwal, S. S. (2019). Agronomic biofortification of chickpea with zinc and iron through application of zinc and urea. Communications in Soil Science and Plant Analysis, 50(15), 1864–1877. https://doi.org/10.1080/00103624.2019.1648490
- Paramesh, V., Dhar, S., Dass, A., Kumar, B., Kumar, A., El-Ansary, D. O., & Elansary, H. O. (2020). Role of integrated nutrient management and agronomic fortification of zinc on yield, nutrient uptake and quality of wheat. Sustainability, 12(9), 3513. https://doi.org/10.3390/su12093513
- Paymaneh, Z., Gryndler, M., Konvalinková, T., Benada, O., Borovička, J., Bukovská, P., Püschel, D., Řezáčová, V., Sarcheshmehpour, M., & Jansa, J. (2018). Soil matrix determines the outcome of interaction between mycorrhizal symbiosis and biochar for Andropogon gerardii growth and nutrition. Frontiers in Microbiology, 9, 2862. https://doi.org/10.3389/fmicb.2018.02862
- Pradhan, S., Mackey, H. R., Al-Ansari, T. A., & McKay, G. (2022). Biochar from food waste: A sustainable amendment to reduce water stress and improve the growth of chickpea plants. Biomass Conversion and Biorefinery, 12(10), 4549–4562. https://doi.org/10.1007/s13399-022-02575-1
- Rahi, A. A., Younis, U., Ahmed, N., Ali, M. A., Fahad, S., Sultan, H., Zarei, T., Danish, S., Taban, S., El Enshasy, H. A., & Tamunaidu, P. (2022). Toxicity of cadmium and nickel in the context of applied activated carbon biochar for improvement in soil fertility. Saudi Journal of Biological Sciences, 29(2), 743–750. https://doi.org/10.1016/j.sjbs.2021.09.035
- Reverchon, F., Flicker, R. C., Yang, H., Yan, G., Xu, Z., Chen, C., Hosseini Bai, S., & Zhang, D. (2014). Changes in δ 15 N in a soil–plant system under different biochar feedstocks and application rates. Biology and Fertility of Soils, 50(2), 275–283. https://doi.org/10.1007/s00374-013-0850-2
- Rogovska, N., Laird, D. A., Rathke, S. J., & Karlen, D. L. (2014). Biochar impact on Midwestern mollisols and maize nutrient availability. Geoderma, 230, 340–347. https://doi.org/10.1016/j.geoderma.2014.04.009
- Roosta, H. R., Estaji, A., & Niknam, F. (2018). Effect of iron, zinc and manganese shortage-induced change on photosynthetic pigments, some osmoregulators and chlorophyll fluorescence parameters in lettuce. Photosynthetica, 56(2), 606–615. https://doi.org/10.1007/s11099-017-0696-1
- Saxena, J., Rana, G., & Pandey, M. (2013). Impact of addition of biochar along with Bacillus sp. on growth and yield of French beans. Scientia Horticulturae, 162, 351–356. https://doi.org/10.1016/j.scienta.2013.08.002
- Selvarajoo, A., Wong, Y. L., Khoo, K. S., Chen, W. H., & Show, P. L. (2022). Biochar production via pyrolysis of citrus peel fruit waste as a potential usage as solid biofuel. Chemosphere, 294, 133671. https://doi.org/10.1016/j.chemosphere.2022.133671
- Semida, W. M., Abdelkhalik, A., Mohamed, G. F., Abd El-Mageed, T. A., Abd El-Mageed, S. A., Rady, M. M., & Ali, E. F. (2021). Foliar application of zinc oxide nanoparticles promotes drought stress tolerance in eggplant (Solanum melongena L.). Plants, 10(2), 421. https://doi.org/10.3390/plants10020421
- Sg, L., Jjo, O., Adeleke, R., & Rhizosphere, M. S. (2021). The potential of biochar to enhance concentration and utilization of selected macro and micro nutrients for chickpea (Cicer arietinum) grown in three contrasting soils. Rhizosphere, 17, 100289. https://doi.org/10.1016/j.rhisph.2020.100289
- Shaaban, M., Van Zwieten, L., Bashir, S., Younas, A., Núñez-Delgado, A., Chhajro, M. A., Kubar, K. A., Ali, U., Rana, M. S., Mehmood, M. A., & Hu, R. (2018). A concise review of biochar application to agricultural soils to improve soil conditions and fight pollution. Journal of Environmental Management, 228, 429–440. https://doi.org/10.1016/j.jenvman.2018.09.006
- Shrivastava, S., Jain, A. K., & Arya, V. (2018). Response of organic manure, zinc and iron on soil properties, yield and nutrient uptake by pearl millet crop grown in inceptisoi. International Journal of Pure & Applied Bioscience, 6(1), 426–435. https://doi.org/10.18782/2320-7051.4026
- Singh, B. P., Hatton, B. J., Singh, B., Cowie, A. L., & Kathuria, A. (2010). Influence of biochars on nitrous oxide emission and nitrogen leaching from two contrasting soils. Journal of Environmental Quality, 39(4), 1224–1235. https://doi.org/10.2134/jeq2009.0138
- Solaiman, Z. M., Shafi, M. I., Beamont, E., & Anawar, H. M. (2020). Poultry litter biochar increases mycorrhizal colonisation, soil fertility and cucumber yield in a fertigation system on sandy soil. Agriculture, 10(10), 480. https://doi.org/10.3390/agriculture10100480
- Sukartono, S. (2011). Soil fertility status, nutrient uptake, and maize (Zea mays L.) yield following biochar and cattle manure application on sandy soils of Lombok, Indonesia. Journal of Tropical Agriculture, 49, 47–52.
- Suzuki, S., Noble, A. D., Ruaysoongnern, S., & Chinabut, N. (2007). Improvement in water-holding capacity and structural stability of a sandy soil in Northeast Thailand. Arid Land Research and Management, 21(1), 37–49. https://doi.org/10.1080/15324980601087430
- Taghizadeh-Toosi, A., Clough, T. J., Sherlock, R. R., & Condron, L. M. (2012). A wood based low-temperature biochar captures NH3-N generated from ruminant urine-N, retaining its bioavailability. Plant and Soil, 353(1–2), 73–84. https://doi.org/10.1007/s11104-011-1010-9
- Ullah, A., Farooq, M., Rehman, A., Hussain, M., & Siddique, K. H. (2020). Zinc nutrition in chickpea (Cicer arietinum): A review. Crop & pasture science, 71, 199–218. https://doi.org/10.1071/CP19357
- Ullah, I., Muhammad, D., Mussarat, M., Khan, S., Adnan, M., Fahad, S., Ismail, M., Mian, I. A., Ali, A., Saleem, M. H., Saeed, M., Gul, F., Ibrahim, M., Raza, M. A. S., Hammad, H. M., Nasim, W., Saud, S., Khattak, J. Z. K. … Khan, S. M. (2022). Comparative effects of biochar and NPK on wheat crops under different management systems. Crop and Pasture Science, 74(2), 31–40. https://doi.org/10.1071/CP21146
- Van Asperen, H. L., Bor, A. M. C., Sonneveld, M. P. W., Bruins, H. J., & Lazarovitch, N. (2014). Properties of anthropogenic soils in ancient run-off capturing agricultural terraces in the central Negev desert (Israel) and related effects of biochar and ash on crop growth. Plant and Soil, 374(1–2), 779–792. https://doi.org/10.1007/s11104-013-1901-z
- Wali, F., Naveed, M., Bashir, M. A., Asif, M., Ahmad, Z., Alkahtani, J., Alwahibi, M. S., & Elshikh, M. S. (2020). Formulation of biochar-based phosphorus fertilizer and its impact on both soil properties and chickpea growth performance. Sustainability, 12(22), 9528. https://doi.org/10.3390/su12229528
- Wolf, B. (1982). A comprehensive system of leaf analysis and its use for diagnosing crop nutrient status. Communication in Soil Science and Plant Analysis, 13(12), 1035–1059. https://doi.org/10.1080/00103628209367332
- Yao, Y., Gao, B., Wu, F., Zhang, C., & Yang, L. (2015). Engineered biochar from biofuel residue: Characterization and its silver removal potential. ACS Applied Materials & Interfaces, 7(19), 10634–10640. https://doi.org/10.1021/acsami.5b03131
- Zhang, D., Wang, Y., Tang, X., Zhang, A., Li, H., & Rengel, Z. (2019). Early priority effects of occupying a nutrient patch do not influence final maize growth in intensive cropping systems. Plant and Soil, 442(1–2), 285–298. https://doi.org/10.1007/s11104-019-04155-1
- Zhang, X., Zhang, F., & Mao, D. (1998). Effect of iron plaque outside roots on nutrient uptake by rice (oryza sativa L.). Zinc uptake by Fe-deficient rice. Plant and Soil, 202(1), 33–39. https://doi.org/10.1023/A:1004322130940
- Zhu, Q., Liu, X., Hao, T., Zeng, M., Shen, J., Zhang, F., & Vries, W. D. (2018). Modeling soil acidification in typical Chinese cropping systems. Science of the Total Environment, 613-614, 1339–1348. https://doi.org/10.1016/j.scitotenv.2017.06.257
- Ziadi, N., Whalen, J. K., Messiga, A. J., & Morel, C. (2014). Assessment and modeling of soil available phosphorus in sustainable cropping systems. Advances in Agronomy, 122, 85–126. https://doi.org/10.1016/B978-0-12-417187-9.00002-4
- Zimmerman, A. R. (2010). Abiotic and microbial oxidation of laboratory produced black carbon (biochar). Environmental Science & Technology, 44(4), 1295–1301. https://doi.org/10.1021/es903140c
- Zingore, S., Delve, R. J., Nyamangara, J., & Giller, K. E. (2008). Multiple benefits of manure: The key to maintenance of soil fertility and restoration of depleted sandy soils on African smallholder farms. Nutrient Cycling in Agroecosystems, 80(3), 267–282. https://doi.org/10.1007/s10705-007-9142-2