Abstract
Food additives change the appearance and taste of foods, prevent their deterioration and keep them longer; are very important chemical compounds in terms of food technology. In this study, the cytotoxicity of the monosodium glutamate, monopotassium glutamate and magnesium di-glutamate salts of L-Glutamic acid are frequently used in foods as food additives and their effects on expression of cancer related (CYP1A1 and BCL-2) genes were investigated in MCF-7 and MCF-12A cell lines. For this purpose, different concentrations of MSG (437.5–27.34 mM), MPG (218.75–13.67 mM) and MDG (109.3–6.83 mM) treated with cells for 24 hours. According to the results, IC50 values of MSG, MPG and MDG were measured as 227.17, 84.64 and 80,27 mM for MCF-7 and 156.53, 197.24 and 14,05 mM for MCF-12A respectively. The most effective concentrations were determined as a result of MTT assay and expression analysis of CYP1A1 and BCL-2 genes were performed in both cell lines by qRT-PCR. BCL-2 gene; it was expressed more than its normal function against the substances in MCF-7 cells and caused unplanned cell proliferation in these cells, while it was detected that it was expressed less than its normal function in MCF-12A cells. Similarly, the CYP1A1 gene was found to be less express than its normal function in MCF-7 cells, while it was overexpressed in MCF-12A cells to against the toxicity from these agents. As a result of this study, the data showed that these food additives may cause both cytotoxicity and genotoxicity.
1. Introduction
Various chemical compounds are added to foods in order to change the appearance and taste of foods according to the wishes of the consumer and to prevent them from spoiling and to be stored longer. These substances that are consciously added to foods before being offered for consumption are called food additives (Basoglu, Citation2016; Yoruk & Danyer, Citation2016).
Food additives can be harmful to public health when used in amounts randomly. Even if they are used in doses that do not harm health, it is predicted that these substances may accumulate in the body after a while and reach amounts that may threaten human health, and then cause tissue damage by cytotoxic and genotoxic. For this reason, researches on this subject are increasing and restrictions are imposed on the use of these substances (Altug, Citation2009; Avuloglu-Yilmaz et al., Citation2020; Yuzbasioglu et al., Citation2014).
Food additives used as flavor enhancers are defined by the International Codex Alimentarius Commission (CAC) as “substances that increase taste and/or odor in food”. It is stated to be used in a wide variety of foods such as confectionery, soft drinks, cereals etc. However, the presence of health concerns about the use of these substances has made their use controversial (Appaiah, Citation2010; Maluly et al., Citation2017). Food and Drug Administration (FDA) defines flavor enhancers as safe but there is no consensus on the health effects of these substances. Such conflicting information also casts doubt on the safe use of these food additives (Uslu & Tosun, Citation2013).
Glutamic acids and glutamate are among the most important food additives used as flavor enhancers in many food products. Monosodium Glutamate (MSG; E621-C5H8NNaO4·xH2O) is the most known and used flavor enhancer in the world and is the sodium salt of L-Glutamic acid. By increasing saliva secretion, MSG strengthens the flavor properties of the food and makes it want to eat more often and faster. And also Monopotassium Glutamate (MPG; E622-C5H8KNO4·H2O) is potassium salt of L-Glutamic acid; another one is Magnesium Diglutamate (MDG; E625-C10H16MgN2O8·4 H2O) is also a magnesium salt. Like other salts of glutamic acid, MPG and MDG are frequently used in foods (Weltin et al., Citation2017).
The some methods or assays used to determine the genotoxic effect such as comet and micronucleus assay, generally detect potential DNA damage. Also, gene expression analysis can be used to understand the mechanism that causes possible genotoxic effect of chemicals and identify early molecular biomarkers associated with carcinogenicity. Therefore, real-time reverse transcription (RT) polymerase chain reaction (PCR) becoming the method of choice for high-throughput and accurate expression profiling of selected genes (Bolukbasi, Citation2021; Hwang & Lee, Citation2012).
Changes in the expression of cancer-related genes directly indicate that that substance may be genotoxic on these genes. Therefore, it is useful to evaluate the genotoxicity profile of these chemicals in terms of these genes. A positive result in genotoxicity tests indicates that the compound or its metabolites interact directly (covalent binding) or indirectly (microtubular protein) with DNA. The result may be a permanent change in DNA base sequence and/or chromosome structure or a complete loss of part of the cellular genome. Such changes are often linked to increased tumor incidence if they affect specific gene/gene domains and if some other conditions in the multi-step process of cancer development are combined (Cartus & Schrenk, Citation2017).
The human CYP1A1 (cytochrome P1450) gene is an important member of the CYP1A family that encodes an enzyme involved in the activation of pro-carcinogens (polycyclic aromatic hydrocarbons and aromatic amines) into reactive metabolites. Cytochrome P450 enzymes are involved in the oxidative metabolism of endogenous compounds such as steroids, fatty acids, leukotrienes, and prostaglandins, and the metabolism of chemicals such as drugs from exogenous compounds, carcinogens and other environmental pollutants (Jones & Nebert, Citation1989; Masson et al., Citation2005). The BCL-2 (B-Cell Lymphoma 2) gene encodes the BCL-2 family of proteins that controls cell death primarily by direct binding interactions. They regulate mitochondrial outer membrane permeabilization (MOMP) leading to the irreversible release of intermembrane space proteins, subsequent caspase activation and apoptosis. These proteins are the regulators of apoptosis, but also have other functions. They regulate and mediate the process by which mitochondria contribute to cell death known as the intrinsic apoptosis pathway (Knight et al., Citation2019; Korsmeyer, Citation1999; Petersen et al., Citation1991; Warren et al., Citation2019).
In the current study, the possible cytotoxic effects of MSG, MPG and MDG substances, which are frequently used in foods, on MCF-7 and MCF-12A human cell lines were determined by MTT (Metiltiazol difenil tetrazolyum) analysis. And then gene expression analysis were performed for the most effective concentrations in MTT assay. Expressions of the CYP1A1 and BCL-2 genes were evaluated in cultured MCF-7 (human breast adenocarcinoma) and MCF 12-A (human mammary epithelial cell) cell lines treated with MSG, MPG and MDG for 24 hours. Expressions of these genes were provided more information about their genotoxic mechanism.
2. Material and methods
2.1. Cell culture
In this study, MCF-7 (ATCC® HTB22™-breast cancer) and MCF-12A (ATCC® CRL-10782-normal breast epithelium) cell lines were cultivated using RPMI-1640 (Roswell Park Memorial Institute) or DMEM (Dulbecco′s Modified Eagle′s-Medium) containing 0.2% sodium bicarbonate, 10% fetal bovine serum (FBS) and 1% penicillin/streptomycin. Cells were grown in 25 cm2 or 75 cm2 flasks at 5% CO2 and 37°C. Cells were grown until they reached 80% fullness and then washed with phosphate buffered saline (PBS). 1X Trypsin-EDTA was used for passage of cells.
2.2. MTT method
The cells counted in a 96-well plate (5×103/well) and 100 μl of RPMI (for MCF-7) or DMEM (for MCF-12A) medium were prepared and inoculated into the wells. The microplate was incubated at 37°C and 5% CO2 for 24 hours, and the cells were adhered to the surface. Different concentrations of MSG (27.34–437.5 mM), MPG (13.67–218.75 mM) and MDG (6.83–109,3 mM) treated with cells for 24 hours. After incubation, MTT (5 mg/mL) solution was added to the wells and exposed for 3 hours, and then the reaction was terminated by adding DMSO (dimethylsulfoxide) to the wells. Incubated cells were measured with a microplate reader (Thermo-Multiskan® GO) spectrophotometer at an absorbance value of 570 nm in triplicate. Cell viability was measured using Microsoft Excel software with the following formula: Viability (%) = mean experimental (optical density) OD value/mean control OD value) ×100 %. One-way ANOVA (Tukey) was performed for the MTT assay test results using SPSS 25.0 for Windows (IBM SPSS, Inc., Chicago, IL).
2.3. RNA extraction, complementary DNA (cDNA) synthesis assay
Total RNA extraction of the control and concentration groups were performed according to the RNeasy Plus Kit from Qiagen (Cat. No/ID: 74034) reagent according to suggested procedures by manufacturer. Afterwards, the amount and purity of RNA were determined using the Nanodrop ND-Spectrometer 1000 device (NanoDrop Technologies, Wilmington, DE, USA) and 1.0% agarose gel electrophoresis. Next, cDNA synthesis was performed using the ProtoScript-II First Strand cDNA Synthesis Kit (BioLabs Inc.). Anchored-oligo(dT)18 primer was used because of the long CYP1A1, BCL-2 and β-actin gene regions.
2.4. The qRT-PCR analyses
The primers of β-actin as housekeeping gene and CYP1A1 and BCL-2 genes used in this study specific to these regions were designed using the information of the MCF-7 and MCF-12A cells in the gene bank (NCBI; National Center for Biotechnology Information). Information about these genes and the primers sequences were designed for this study are given in Table .
Table 1. Gene information in the NCBI database, sequences and melting temperatures of primers designed for use in qRT-PCR
Following cDNA synthesis, Real-Time PCR applications were performed using SYBR Green I Master dye via Pico Real Time (Thermo). The mRNA levels of CYP1A1 and BCL-2 were determined by qPCR method in order to determine the effect of the most effective concentrations of food additives on the expression of genes related to cancer. PCR conditions consisted initial denaturation 10 min at 95°C, (40 cycles) 95°C for 15 s, 60°C for 25 s, 72°C for 30 s, and a melting analysis of 52°C to 95°C with an increasing temperature 0.5°C min−1. The qRT-PCR analysis contained three biological replicates, consisting of three technical replicates using the obtained optimal conditions.
2.5. Normalization and statistical analysis of qPCR results
Gene expression results determined as Ct value were normalized by considering beta-actin and control conditions used in the study as housekeeping gene (Livak & Schmittgen, Citation2001). Real-Time PCR reaction of CYP1A1 and BCL-2 genes were monitored simultaneously for the most effective concentration in MTT analysis and their peak profiles were recorded. The Ct values of each sample were determined by these peak profiles. The mRNA levels of the synthesized gene products were determined quantitatively by these obtained Ct values and Melting Curve Analysis (Kubista et al., Citation2006). The obtained data were normalized according to the 2−ΔΔCt method of Livak and Schmittgen (Livak & Schmittgen, Citation2001). The mean, standard deviation, standard error and statistical significance of these data were calculated with the statistical program SPSS 25.0 for Windows (IBM SPSS, Inc., Chicago, IL).
3. Results and discussions
While the developments and recent innovations in the food industry have eliminated some of the problems encountered in the past, they have also brought some new problems. As a result of the production techniques brought by the developing technology and the diversity of consumer taste, the use of food additives in the food industry is increasing day by day. While people continue to access durable and abundant food sources in the industrialized world, their concerns about food safety are steadily increasing (Amin et al., Citation2013; Erden Calişir & Caliskan, Citation2003; Fleming et al., Citation2006). For this reason, in order to ensure the best possible protection of human health, it is necessary to consider the food production chain as a system, to constantly monitor food safety, to consider scientific evidence and all kinds of risk assessments.
There are a significant number of studies on the genotoxic and cytotoxic effects of food additives (Adeyemo & Farinmade, Citation2013; Akin & Sumer, Citation1991; Ataseven et al., Citation2016; Canitezer & Topaktas, Citation2012). However, although it is seen that only MSG, one of the substances used in our study, has been studied extensively in this regard, the number of studies with human cells is very few and the results obtained are contradictory. Studies for the other two food additives are very limited and therefore there is a significant gap in the literature. Potential cytotoxicity and genotoxicity of all three flavor enhancers were tried to be determined, molecular mechanisms were determined in MCF-7 and MCF-12A cell lines and preliminary data for more detailed studies were revealed.
In the literature review, no study was found that examined the cytotoxicity of the food additives (MSG, MPG and MDG) examined in this study in human cancer cell lines. Although most of the studies have focused on the genotoxic effects of these substances (Khatab & Elhaddad, Citation2015; Prasath & Surya, Citation2013; Renjana et al., Citation2013; Turkoglu, Citation2015) effects on the activity of cancer-related genes have not been reported yet.
Many chemical agents cause cytotoxicity by damaging cells at different levels. In order to determine the biological activity of these agents, it is necessary to determine whether they are toxic or not. For this purpose, cytotoxicity tests are performed in in vitro cell culture. These tests allow the activity of many agents to be examined in a short time and provide basic data for future evaluations. The 3-(4,5-dimethylthiazol-2-yl)-2,5-diphenyl tetrazolium bromide (MTT) test, which is used to evaluate cytotoxicity or cell viability, is one of the most preferred colorimetric tests (Ghasemi et al., Citation2021). The test is fast, reliable and easy to apply and is preferred in many laboratories worldwide (Tolosa et al., Citation2015).
In the current study, it was aimed to determine the cytotoxicity of MSG, MPG and MDG, which are frequently preferred flavor enhancers, in human cell cultures and to determine the expression of the most effective concentrations in terms of cancer-related genes.
The 50% inhibitory concentration (IC50) value was calculated by determining the MTT results and the applied dose and % cell viability curve with the Microsoft Excel (Cytotoxicity = test absorbance value/control absorbance value average x100). The experiments were repeated three times for each chemical concentration and the results are shown in Table (Avuloglu-Yilmaz et al., Citation2020). According to the results, IC50 values of MSG, MPG and MDG were measured as 227.17, 84.64 and 80.27 mM for MCF-7 and 156.53, 197.24 and 14.05 mM for MCF-12A, respectively. The results showed that all three food additives have the ability to induce cytotoxicity in MCF-7 and MCF-12A cell lines (Table-2).
Table 2. MTT results of MSG, MPG and MDG treatment on MCF-7 and MCF-12A cell lines
Considering the data obtained from the MTT analysis and the IC50 values, Real Time-PCR assays were studied by applying concentrations of 437.5 mM, 218.7 mM and 109.3 mM for MSG, MPG and MDG, respectively, in both cell lines. Therefore, molecular biological analysis was only tested for these concentrations. Because these concentrations are the most effective doses in common in both cell lines.
In this current study, the genotoxicity of MSG, MPG and MDG which are one of the flavor enhancers frequently used in foods; and the effects of these substances on the expression of CYP1A1 and BCL-2 genes that are cancer related were investigated in MCF-7 (breast cancer) and MCF-12A (normal breast epithelium) cells. The changes in the expression levels of the targeted genes in the study were determined by Real-Time PCR method. The qPCR method is a very effective method for detecting genes, determining their functions, testing and determining their relationship with various factors (Bolukbasi, Citation2022; Buyuk et al., Citation2016; Kubista et al., Citation2006).
Along with these results, the most effective concentrations were determined as a result of MTT assay and gene expression analyzes of the transcript profiles of CYP1A1 and BCL-2 genes were performed in MCF-7 and MCF-12A cells to which three different substances were applied. Transcript profiles of CYP1A1 and BCL-2 genes and control profiles of MCF-7 and MCF-12A cells were compared with Beta-actin gene selected as a housekeeping gene. The obtained data were normalized according to the 2−ΔΔCt method of Livak and Schmittgen (Livak & Schmittgen, Citation2001).
Normalized Real-Time PCR results of MCF-7 and MCF-12A cells and likewise, the mean, standard error and standard deviation of the gene expression data obtained as a result of normalization were calculated and the results are given in Table .
Table 3. Mean, standard deviation and standard error of CYP1A1 and BCL-2 gene expression data obtained as a result of normalization of MCF-7 and MCF-12A cells
The normalized gene expression data were averaged and the changes in the expression level of the CYP1A1 and BCL-2 genes at the determined (IC50) concentration depending on the obtained results were shown on the figures for each food additive, separately.
In MCF-7 (breast cancer) cells; the BCL-2 gene expression level reached the highest level with an approximately 2.3-fold increase in the determined concentration of MSG compared to the control group (P < 0.05). This increase was determined as 1.18 times in MPG. In MDG treatment, it increased approximately 1.2 times (P < 0.05). On the contrary, a decrease in gene expression levels was observed in all treatment substances at CYP1A1 gene expression level compared to the control group. While the decrease in the determined concentration of MSG was 0.54 times compared to the control group (P < 0.05), it reached the lowest level with 0.42 times in MPG. Similarly, a 0.76-fold change was observed in the MDG treatment Table and Figures ).
Figure 1. Concentration-related changes in the expression levels of CYP1A1 and BCL-2 genes determined in MSG-treated MCF-7 (breast cancer) and MCF-12A (normal breast epithelium) cell lines.
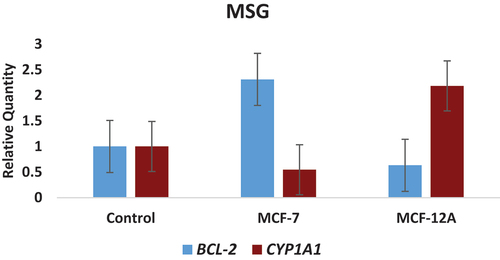
Figure 2. Concentration-related in the expression levels of CYP1A1 and BCL-2 genes determined in MPG-treated MCF-7 (breast cancer) and MCF-12A (normal breast epithelium) cell lines.
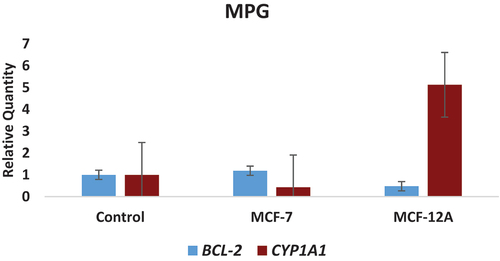
Figure 3. Concentration-related changes in the expression levels of CYP1A1 and BCL-2 genes determined in MDG-treated MCF-7 (breast cancer) and MCF-12A (normal breast epithelium) cell lines.
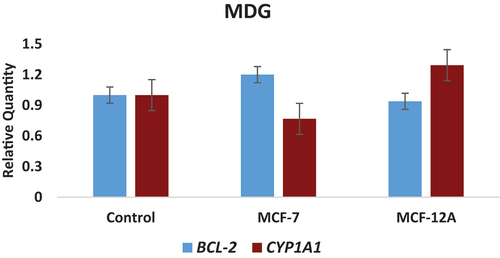
In MCF-12A (normal breast epithelium) cells, a decrease in gene expression levels was observed in all substances at the BCL-2 gene expression level compared to the control group. While the decrease in the determined dose of MSG was 0.63 times compared to the control group, it reached the lowest level with 0.47 times in MPG (P < 0.05). A change of approximately 0.93 fold was observed in MDG application. An approximately 2-fold increase in the determined dose of MSG was detected in the CYP1A1 gene expression level compared to the control group (P < 0.05). This increase reached the highest level with 5 times in MPG. In MDG application, it increased approximately 1.3 times (P < 0.05) Table and Figures ).
Many studies in the literature have reported that the CYP1A1 encodes one of the main cytochrome P450 enzymes, examined extensively for its capacity to activate compounds with carcinogenic properties (Androutsopoulos et al., Citation2009; Annick et al., Citation2019; Masson et al., Citation2005). Continuous exposure to some chemicals and environmental carcinogens is thought to increase the level of CYP1A1 expression in extrahepatic tissues. Cancer progression or prevention may depend on the balance of procarcinogen activation/detoxication of CYP1A1 and dietary natural product extrahepatic metabolism (Malekinejad et al., Citation2022). In addition, CYP1A1 plays an important role in the detoxication of environmental carcinogens, as well as in the metabolic activation of dietary compounds with cancer preventative activity (Aaron et al., Citation2018; Abu Zeid et al., Citation2021; Zhu et al., Citation2019).
Additionally, apoptosis is a very important biological process. Impaired apoptosis plays an important role in cancer development and also reduces the efficacy of conventional cytotoxic treatments (Colin et al., Citation2020; Koohpeyma et al., Citation2021). Recently, many researchs have focused on the role of the BCL-2 gene and BCL-2 family proteins in regulating apoptosis and how BCL-2 genes are affected by diet. Members of the B-cell lymphoma 2 (BCL-2) protein family have anti-apoptotic activities (Soumerai et al., Citation2022). They have been extensively studied for their importance in regulating tumorigenesis and cellular responses to anticancer therapy. All findings point to the potential of BCL-2 family proteins in cancer therapy (Alakananda Basu, Citation2022; Kassab et al., Citation2022; Zhang et al., Citation2021).
The results obtained from current study support each other with the literature studies mentioned above. As a result, the data obtained from this study were evaluated as a whole, the BCL-2 gene was expressed more than its normal function against the MSG, MPG and MDG in MCF-7 (breast cancer) cells, and it further promoted the unscheduled cell proliferation event seen in cancer cells by increasing the expression level of the BCL-2 gene due to MSG, MPG and MDG. In MCF-12A cells, on the other hand, the BCL-2 gene was less expressed than its normal function against the substances and could not fully express its function due to these substances.
Similarly, CYP1A1 gene is less expressed than its normal function against the MSG, MPG and MDG in MCF-7 cells and it was not enough to eliminate the toxicity caused by these substances. In other words, the MSG, MPG and MDG worsened the situation of coping with toxicity in cancer cells. And also, in MCF-12A cells, the CYP1A1 gene was overexpressed against the substances than its normal function and was overexpressed to counteract the toxicity from these agents.
In conclusion, MSG, MPG and MDG, cytotoxic and genotoxic effects were examined, were included in the “ADI Not Specified-ADI (Daily Acceptable Intake) Not Determined” group by JECFA. On the other hand, it has been included in the “GRAS-Generally Recognized As Safe” class by the FDA. Although the food additives in question are considered safe for human health by all health, food authorities and scientific organizations in the world, it was concluded that they have genetic risk in vitro conditions and at high concentrations, according to the results of this study. However, these results need to be supported by using the expression potential of different cancer-related genes with other cell lines. In our study, it has been shown that gene expression analysis is important to understand the mechanism involved in the genotoxicity of these chemicals and to identify early molecular biomarkers associated with food additive-induced carcinogenicity in these cells. In addition, it has been determined that the genes examined in our study are good candidate for future cancer research.
Statement contribution of the authors
This study’s experimentation, analysis and writing, etc. all steps were taken by the authors.
Statement of ethics
There is no need for an ethics committee decision for the studies in the article.
Disclosure statement
Authors have no any financial or personal relationships with other individuals or organizations that might inappropriately influence this work during the submission process.
Additional information
Funding
Notes on contributors
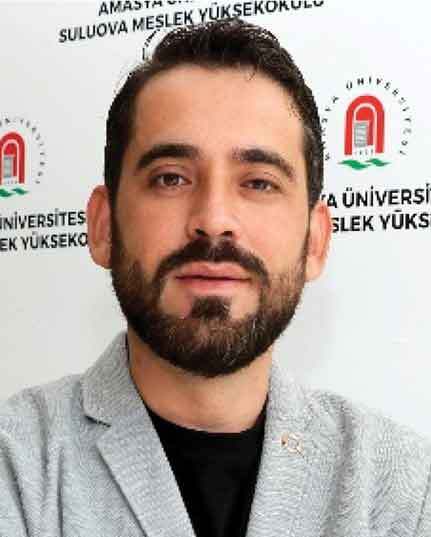
Ekrem Bolukbasi
Ekrem Bölükbaşi graduated a degree in Biology at Erciyes University in 2009. He continued his studies within the Institute of Natural and Applied Sciences at Ankara University, completing his master's degree in 2013 and his PhD in 2018 both in the field of Biotechnology and Molecular Biology. Throughout his academic journey, his research interests have focused on Molecular Biology, Biotechnology, Plant Biotechnology, Agricultural Biotechnology, Environment Biotechnology, Food Biotechnology, Genotoxicity, Methylation Models, Gene Expression, Cancer Biology and Polar (Antarctic Peninsula) Molecular studies. Currently, he studies as an Associate Professor Doctor at Amasya University, working within the Department of Biology and Biotechnology, as well as the Department of Environmental Protection Technologies and the Central Research Laboratory.
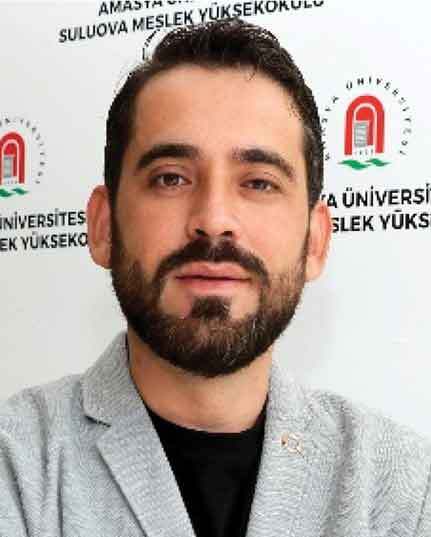
Ece Avuloglu-Yilmaz
Ekrem Bölükbaşi graduated a degree in Biology at Erciyes University in 2009. He continued his studies within the Institute of Natural and Applied Sciences at Ankara University, completing his master's degree in 2013 and his PhD in 2018 both in the field of Biotechnology and Molecular Biology. Throughout his academic journey, his research interests have focused on Molecular Biology, Biotechnology, Plant Biotechnology, Agricultural Biotechnology, Environment Biotechnology, Food Biotechnology, Genotoxicity, Methylation Models, Gene Expression, Cancer Biology and Polar (Antarctic Peninsula) Molecular studies. Currently, he studies as an Associate Professor Doctor at Amasya University, working within the Department of Biology and Biotechnology, as well as the Department of Environmental Protection Technologies and the Central Research Laboratory.
Ece Avuloglu Yilmaz is an Assistant Professor Doctor at Department of Health Information Systems, Technical Sciences Vocational School, Amasya University in TÜRKİYE. Her research interests have focused on Biology, Molecular Biology, Biotechnology, Genotoxicity, Cytotoxicity, Cell culture, Cytogenetics, Cancer Biology.
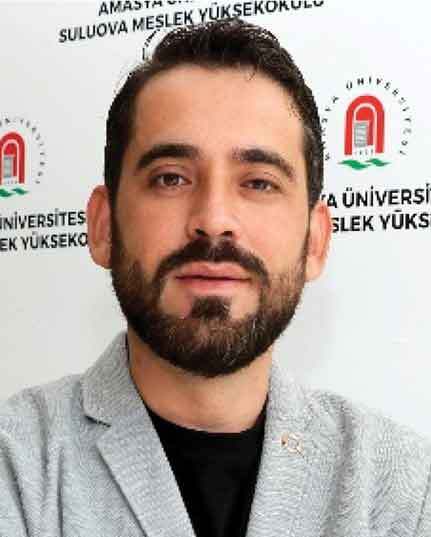
Tuba Yildirim
Ekrem Bölükbaşi graduated a degree in Biology at Erciyes University in 2009. He continued his studies within the Institute of Natural and Applied Sciences at Ankara University, completing his master's degree in 2013 and his PhD in 2018 both in the field of Biotechnology and Molecular Biology. Throughout his academic journey, his research interests have focused on Molecular Biology, Biotechnology, Plant Biotechnology, Agricultural Biotechnology, Environment Biotechnology, Food Biotechnology, Genotoxicity, Methylation Models, Gene Expression, Cancer Biology and Polar (Antarctic Peninsula) Molecular studies. Currently, he studies as an Associate Professor Doctor at Amasya University, working within the Department of Biology and Biotechnology, as well as the Department of Environmental Protection Technologies and the Central Research Laboratory.
Tuba Yildirim is a Professor Doctor at Department of Biology, Faculty of Arts and Science, Amasya University. She has many studies related to her subjects as Medical Biotechnology, Vaccine Technology and Molecular Microbiology.
References
- Aaron, G., Bart, A. G., & Scott, E. E. (2018). Structures of human cytochrome P450 CYP1A1 with bergamottin and erlotinib reveal active-site modifications for binding of diverse ligands. Journal of Biological Chemistry, 293(50), 19201–11. https://doi.org/10.1074/jbc.RA118.005588
- Abu Zeid, E. H., El Sharkawy, N. I., Moustafa, G. G., Anwer, A. M., & Al Nady, A. G. (2021). The palliative effect of camel milk on hepatic CYP1A1 gene expression and DNA damage induced by fenpropathrin oral intoxication in male rats. Ecotoxicology and Environmental Safety, 207, 111296. https://doi.org/10.1016/j.ecoenv.2020.111296
- Adeyemo, O. A., & Farinmade, A. E. (2013). Genotoxic and cytotoxic effects of food flavor enhancer, Monosodium Glutamate (MSG) using allium cepa assay. African Journal of Biotechnology, 12(13), 1459–1466. https://doi.org/10.5897/AJB12.2927
- Akin, A., & Sumer, S. (1991). The mutagenic effects of sodium nitrite and monosodium glutamate used as food additives demonstrated by the salmonella microsome test system. Mikrobiyoloji Bülteni, 25(1), 94–107. https://pubmed.ncbi.nlm.nih.gov/1881365
- Alakananda Basu, A. (2022). The interplay between apoptosis and cellular senescence: BCL-2 family proteins as targets for cancer therapy. Pharmacology & Therapeutics, 230, 107943. https://doi.org/10.1016/j.pharmthera.2021.107943
- Altug, T. (2009). Gıda Katkı Maddeleri. In T. Altuğ (Ed.), Food additives (3rd ed., pp. 1–16). Kan Yılmaz Publishing.
- Amin, L., Azad, M. A. K., & Samian, A. L. (2013). Factor influencing risk perception of food additives. Journal of Food, Agriculture and Environment, 11(2), 66–72. https://doi.org/10.1234/4.2013.4192
- Androutsopoulos, V. P., Tsatsakis, A. M., & Spandidos, D. A. (2009). Cytochrome P450 CYP1A1: Wider roles in cancer progression and prevention. BMC Cancer, 9(1), 187. https://doi.org/10.1186/1471-2407-9-187
- Annick, D. B., Villevoye, J., Nijmeijer, S. M., Van den Berg, M., & Majorie, B. M. (2019). Anti-tumor properties of methoxylated analogues of resveratrol in malignant MCF-7 but not in non-tumorigenic MCF-10A mammary epithelial cell lines. Toxicology, 422, 35–43. https://doi.org/10.1016/j.tox.2019.04.009
- Appaiah, K. M. (2010). Monosodium glutamate in foods and its biological effects. In C. Boisrobert, S. Oh, A. Stjepanovic, & H. Lelieveld Eds., Ensuring global food safety (pp. 217–226). https://doi.org/10.1016/B978-0-12-374845-4.00013-8
- Ataseven, N., Yuzbasioglu, D., Keskin, A. Ç., & Unal, F. (2016). Genotoxicity of monosodium glutamate. Food and Chemical Toxicology, 91, 8–18. https://doi.org/10.1016/j.fct.2016.02.021
- Avuloglu-Yilmaz, E., Bolukbasi, E., & Yildirim, T. (2020). Investigation of the cytotoxic effects of three different food additives using MTT assay. International Eurasian Conference on Biotechnology and Biochemistry, December 16-18, 2020, Ankara, Turkey.
- Avuloglu-Yilmaz, E., Yuzbasioglu, D., & Unal, F. (2020). In vitro genotoxicity assessment of monopotassium glutamate and magnesium diglutamate. Toxicology in Vitro, 65, 104780. https://doi.org/10.1016/j.tiv.2020.104780
- Basoglu, F. (2016). Fundamentals of food quality control and food safety management systems (3rd ed.). Dora Publishing.
- Bolukbasi, E. (2021). Expression analysis of some stress-related genes induced by cadmium on tomato (S. lycopersicum L.) plants. Hittite Journal of Science and Engineering, 8(4), 339–345. https://doi.org/10.17350/HJSE19030000247
- Bolukbasi, E. (2022). Influence of boron treatments on fatty acid desaturase metabolism in different safflower cultivars. Soil and Environment, 68(10), 479–486. https://doi.org/10.17221/228/2022-PSE
- Buyuk, I., Bolukbasi, E., & Aras, E. S. (2016). Expression of CtFAD2 gene for early selection in safflower oleic linoleic oil content. Journal of Animal and Plant Sciences, 26(5), 1383–1388. https://www.thejaps.org.pk/docs/v-26-05/27.pdf
- Canitezer, T., & Topaktas, M. (2012). Effects of monosodium glutamate (MSG) on sister chromatid exchange in human peripheral lymphocytes. Cukurova University Journal of the Faculty of Engineering, 28(2), 76–85. https://fbe.cu.edu.tr/storage/fbeyedek/makaleler/cild28sayi2-76-85.html
- Cartus, A., & Schrenk, D. (2017). Current methods in risk assessment of genotoxic chemicals. Food and Chemical Toxicology, 106, 574–582. https://doi.org/10.1016/j.fct.2016.09.012
- Colin, D., Godwin, O. M., Bates, S. R. J., George, S., Laszlo, G. S., & Walter, R. B. (2020). Anti-apoptotic BCL-2 family proteins confer resistance to calicheamicin-based antibody-drug conjugate therapy of acute leukemia. Leukemia & Lymphoma, 61(12), 2990–2994. https://doi.org/10.1080/10428194.2020.1786553
- Erden Calişir, Z., & Caliskan, D. (2003). Food additives and effects on the human health. Journal of Faculty of Pharmacy of Ankara University, 32(3), 207–206. https://doi.org/10.1501/Eczfak_0000000411
- Fleming, K., Thorson, E., & Zhang, Y. (2006). Going beyond exposure to local news media: An information-processing examination of public perceptions of food safety. Journal of Health Communication, 11(8), 789–806. https://doi.org/10.1080/10810730600959705
- Ghasemi, M., Turnbull, T., Sebastian, S., & Kempson, I. (2021). The MTT assay: Utility, limitations, pitfalls, and interpretation in bulk and single-cell analysis. International Journal of Molecular Sciences, 22(23), 12827. https://doi.org/10.3390/ijms222312827
- Hwang, Y., & Lee, M. (2012, May). Comparison of the AdvanSure human papillomavirus screening real-time PCR, the Abbott RealTime high risk human papillomavirus test, and the hybrid capture human papillomavirus DNA test for the detection of human papillomavirus. Annals of Laboratory Medicine, 32(3), 201–205. https://doi.org/10.3343/alm.2012.32.3.201
- Jones, J. E., & Nebert, D. W. (1989). Transcriptional start site in the mouse CYP1A1 (cytochrome P1450) gene. DNA (Mary Ann Liebert, Inc), 8(7), 527–534. https://doi.org/10.1089/dna.1.1989.8.527
- Kassab, R. B., Theyab, A., Al-Ghamdy, A. O., Algahtani, M., Mufti, A. H., Alsharif, K. F., Abdella, E. M., Habotta, O. A., Omran, M. M., Lokman, M. S., Bauomy, A. A., Albrakati, A., Baty, R. S., Hassan, K. E., Alshiekheid, M. A., Abdel Moneim, A. E., & Elmasry, H. A. (2022). Protocatechuic acid abrogates oxidative insults, inflammation, and apoptosis in liver and kidney associated with monosodium glutamate intoxication in rats. Environmental Science and Pollution Research, 29(8), 12208–12221. https://doi.org/10.1007/s11356-021-16578-4
- Khatab, H. A., & Elhaddad, N. S. (2015). Evaluation of mutagenic effects of monosodium glutamate using allium cepa and antimutagenic action of origanum majorana L. and ruta chalepensis medical plants. British Biotechnology Journal, 8(1), 1–11. https://doi.org/10.9734/BBJ/2015/17695
- Knight, T., Luedtke, D., Edwards, H., Taub, J. W., & Ge, Y. (2019). A delicate balance-the BCL-2 family and its role in apoptosis, oncogenesis, and cancer therapeutics. Biochemical Pharmacology, 162, 250–261. https://doi.org/10.1016/j.bcp.2019.01.015
- Koohpeyma, F., Siri, M., Allahyari, S., Mahmoodi, M., Saki, F., & Dastghaib, S. (2021). The effects of L-carnitine on renal function and gene expression of caspase-9 and BCL-2 in monosodium glutamate‐induced rats. BMC Nephrology, 22(1), 162. https://doi.org/10.1186/s12882-021-02364-4
- Korsmeyer, S. J. (1999). BCL-2 gene family and the regulation of programmed cell death. Cancer Research, 59(7), 1693–1700. https://pubmed.ncbi.nlm.nih.gov/10197582/
- Kubista, M., Andrade, J. M., Bengtsson, M., Forootan, A., & Jonak, J. (2006). The real-time polymerase chain reaction. Molecular Aspects of Medicine, 2(3), 95–125. https://doi.org/10.1016/j.mam.2005.12.007
- Livak, J. K., & Schmittgen, D. T. (2001). Analysis of relative gene expression data using Real-time quantitative PCR and the 2−ΔΔCT method. Methods [Internet], 25(4), 402–408. https://doi.org/10.1006/meth.2001.1262
- Malekinejad, F., Fink-Gremmels, J., & Malekinejad, H. (2022). Zearalenone and its metabolite exposure directs oestrogen metabolism towards potentially carcinogenic metabolites in human breast cancer MCF-7 cells. Mycotoxin Research, 53(1), 45–56. https://doi.org/10.1007/s12550-022-00472-0
- Maluly, H. D., Arisseto‐Bragotto, A. P., & Reyes, F. G. (2017). Monosodium glutamate as a tool to reduce sodium in foodstuffs: Technological and safety aspects. Food Science & Nutrition, 5(6), 1039–1048. https://doi.org/10.1002/fsn3.499
- Masson, L. F., Sharp, L., Cotton, S. C., & Little, J. (2005). Cytochrome P-450 CYP1A1 gene polymorphisms and risk of breast cancer: A Huge review. American Journal of Epidemiology, 161(10), 901–915. https://doi.org/10.1093/aje/kwi121
- Petersen, D. D., McKinney, C. E., Ikeya, K., Smith, H. H., Bale, A. E., McBride, O. W., & Nebert, D. W. (1991). Human CYP1A1 gene: Cosegregation of the enzyme inducibility phenotype and an RFLP. American Journal of Human Genetics, 48(4), 720. https://pubmed.ncbi.nlm.nih.gov/1707592/
- Prasath, D., & Surya, S. M. (2013). Detection of genotoxicity and chromosomal aberrations induced by furadan and monosodium glutamate in allium cepa. South Asian Journal of Biological Science, 3(1), 11–24. https://worldveg.tind.io/record/50134#record-files-collapse-header
- Renjana, P. K., Anjana, S., & Thoppil, J. E. (2013). Evaluation of genotoxic effects of baking powder and monosodium glutamate using allium cepa assay. International Journal of Pharmacy and Pharmaceutical Science, 5(2), 311–315. https://worldveg.tind.io/record/50133
- Soumerai, J. D., Opat, S., Cheah, C. Y., Lasica, M., Verner, E., Peter, J., & Browett, P. J. (2022). CLL-118 a phase 1 study with the novel B-cell lymphoma 2 inhibitor BGB-11417 as monotherapy or in combination with zanubrutinib in patients with B-cell malignancies. Clinical Lymphoma Myeloma and Leukemia, 22(2), 267–268. https://doi.org/10.1016/S2152-2650(22)01326-X
- Tolosa, L., Donato, M. T., & Gómez-Lechón, M. J. (2015). General cytotoxicity assessment by means of the MTT assay. In Protocols in in vitro hepatocyte research (pp. 333–348). Humana Press. https://doi.org/10.1007/978-1-4939-2074-7_26
- Turkoglu, S. (2015). Evaluation of genotoxic effects of five flavour enhancers (glutamates) on the root meristem cells of allium cepa. Toxicology and Industrial Health, 31(9), 792–801. https://doi.org/10.1177/0748233713475509
- Uslu, D., & Tosun, H. (2013). Glutamic acid production and general application areas. Electronic Journal of Food Technologies, 8(2), 18–28. www.teknolojikarastirmalar.com
- Warren, C. F. A., Wong-Brown, M. W., & Bowden, N. A. (2019). BCL-2 family isoforms in apoptosis and cancer. Cell Death & Disease, 10(3), 177. https://doi.org/10.1038/s41419-019-1407-6
- Weltin, A., Kieninger, J., & Urban, G. A. (2017). Highly sensitive electrochemical glutamate microsensors for food analysis. Multidisciplinary Digital Publishing Institute Proceedings, 1(4), 521. https://doi.org/10.3390/proceedings1040521
- Yoruk, N. G., & Danyer, E. (2016). General information and definitions of food additives. Journal of Food Hygiene and Technology-Special Topics, 2(2), 1–10. https://doi.org/10.31795/baunsobed.580561
- Yuzbasioglu, D., Zengin, N., & Unal, F. (2014). Food preservatives and genotoxicity tests. Gıda/The Journal of Food, 39(3), 179–186. https://doi.org/10.5505/gida.24861
- Zhang, L., Lu, Z., & Zhao, X. (2021). Targeting BCL-2 for cancer therapy. Biochimica Et Biophysica Acta (BBA) - Reviews on Cancer, 1876(1), 188569. https://doi.org/10.1016/j.bbcan.2021.188569
- Zhu, W., Liu, H., Wang, X., Lu, J., Zhang, H., Wang, S., & Yang, W. (2019). Associations of CYP1 polymorphisms with risk of prostate cancer: An updated meta-analysis. Bioscience Reports, 39(3), 20181876. https://doi.org/10.1042/BSR20181876