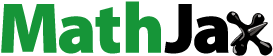
Abstract
A simple liquid-liquid extraction (LLE) procedure and liquid chromatography-tandem mass spectrometer (LC-MS/MS) were validated to analyze the residues of diflubenzuron and novaluron in tomatoes. Validation criteria included; linearity in the range of 2–100 μg.kg−1 and 5–200 μg.kg−1 with a correlation coefficient (R2) of ≥ 0.998, trueness (% recovery) of 90.55–97.51% at spiking levels of 10, 100, and 1000 mg.kg−1, the limit of detection (LOD) of 0.46 and 3.22 μg.kg−1, the limit of quantitation (LOQ) of 5 and 10 μg.kg−1, intraday repeatability (RSDr) of 9.31% and 6.63%, and inter-day repeatability (RSDR) of 13.72% and 8.87%, respectively for diflubenzuron and novaluron. Due to the matrix effect, negligible enhancement in instrument response was observed for the tested analytes. The dissipation behaviour of diflubenzuron (24 % SC) and novaluron (10 % EC) in greenhouse tomatoes after treatment with 192 and 40 g a.i. ha-1 (authorized dosage) and 336 and 80 g a.i. ha-1 (high dosage) was investigated. Dissipation kinetics were best fitted to the first-order reaction. Despite the application dose for novaluron being about three times smaller compared to diflubenzuron, the residues of novaluron dissipated more rapidly than those of diflubenzuron with constant rates (k) of 0.342–0.356 and 0.194–0.154 day-1 and half-lives (t0.5) of 1.77–1.73 and 3.57–4.49 days respectively, at the doses tested. The terminal residues of diflubenzuron and novaluron were 0.013–0.328 and 0.011–0.171 mg.kg-1 after 2 and 3 times application, respectively. The calculated risk quotients were less than 1, indicating that spraying tomatoes with the tested insecticides at the applied doses poses no significant potential risks to Saudi consumers.
1. Introduction
Tomatoes (Lycopersicum esculentum Mill.) are a popular vegetable crop grown in greenhouses and outdoors. In Saudi Arabia, 6.2 million tons of tomatoes were harvested from 15,000 hectares in 2021, representing 0.24% of the world’s production (FAOSTAT, Citation2023). Due to their high content of nutrients such as vitamins, lycopene, iron, potassium, and phosphorus, they are beneficial to health (Carvalho & Pagliuca, Citation2007). Tomatoes are susceptible to various diseases during cultivation, so pesticides are often used to avoid crop losses (Jankowska et al., Citation2016).
Diflubenzuron (1-(4-chlorophenyl)-3-(2,6-di-fluoro-benzoyl) urea) (Figure ) was the first commercially available insecticide from the benzoylphenylureas group (Grosscurt & Jongsma, Citation1987 Citation1987). It has potent and dose-dependent induction in human hepatocytes (Chen et al., Citation2014) and has been classified as chronic risk level III (Nougadère et al., Citation2011). Diflubenzuron is used to control of tomato moth (Spodoptera littoralis) (Keith & Walker, Citation1992) and acts as an effective stomach and contact poison by preventing the incorporation of N-acetylglucosamine into chitin and thus forming the insect cuticle (exoskeleton) (Nakagawa et al., Citation1993). Novaluron [(±)-1-[3-chloro-4-(1,1,2-trifluoromethyl) phenyl]-3-(2,6-difluoro-benzoyl) urea] (Figure ) is a new chitin synthesis inhibitor, an insect growth regulator (IGR) with a broad spectrum of insecticidal activity (Sharma et al., Citation2021) that is safer for beneficial insects and compatible with integrated pest management for the control of tomato leafminer or tomato Borer (e.g. Tuta absoluta) (IRAC, Citation2011; Tomlin, Citation1997). Few data are available on how diflubenzuron and novaluron dissipated in agricultural products. Diflubenzuron residues have been studied in peaches (Dong et al., Citation2019; Si et al., Citation2017), peppers and zucchini (López‐López et al., Citation2004), and strawberries (Chang et al., Citation2020). Conversely, Novaluron has been studied in chilli, brinjal (Das, Citation2007), tomatoes (Malhat et al., Citation2014), and citrus (Dong et al., Citation2018).
Growing under greenhouse conditions creates year-round agroecology for plant development (Emekli et al., Citation2010). However, there is also a negative side: the possibility of pesticide residues being present in high concentrations that can harm human health in various ways and are also responsible for ecosystem destruction (VSS et al., Citation2020; Yalçın et al., Citation2023), especially when overused in greenhouses, as the sprayed pesticides are poorly dispersed by photosynthesis (blocking specific light wavelengths) and evaporation (Katsoulas et al., Citation2012).
Developing precise analytical methods and appropriate field trials to collect essential data for establishing the pre-harvest interval (PHI) and the maximum residue limit (MRL) is necessary for assessing human risks (Arrebola et al., Citation2001; Valverde Garcia et al., Citation1993, Citation1993). Different chromatographic techniques were used for the analysis of diflubenzuron and novaluron in various crops; Liquid chromatography with a diode array detector (HPLC-DAD) (Chang et al., Citation2020; Malhat et al., Citation2014; Miliadis et al., Citation1999), with a UV detector (Tsiropoulos et al., Citation1999), and with tandem mass spectrometry (HPLC-MS/MS) (Chang et al., Citation2020; Chen et al., Citation2014; Dong et al., Citation2019; Si et al., Citation2017; Yalçın et al., Citation2023), and gas chromatography in conjunction with a mass spectrometer (GC-MS) for the determination of diflubenzuron (Wimmer et al., Citation1991) and in conjunction with an electron capture detector (GC-ECD) for the determination of novaluron (Sharma et al., Citation2021).
This article aims to validate a simple, rapid, and effective method for the determination of diflubenzuron and novaluron in tomato fruits using acetonitrile as an extraction solvent for liquid-liquid extraction (LLE), and a liquid chromatography-tandem mass spectrometer (LC-MS/MS) for quantitation. The validated method was used to investigate the dissipation profile and terminal residues of diflubenzuron and novaluron in tomatoes grown under greenhouse conditions in Najran, Saudi Arabia after different doses had been applied to determine pre-harvest intervals (PHI) and to assess the dietary risk associated with diflubenzuron and novaluron residues in tomatoes.
2. Materials and methods
2.1. Chemicals
Diflubenzuron and novaluron certified reference standards with purity ≥ 98.7% were brought from Chem Service Inc. (West Chester, PA, USA). LC-MS grade acetonitrile (MeCN), methanol (MeOH), ammonium format, and formic acid were brought from Fisher Scientific (Loughborough, UK). High-purity sodium chloride (NaCl) and anhydrous magnesium sulfate (MgSO4) were brought from Chem-Lab NV (Zedelgem, Belgium). Ceramic homogenizer was sold from Agilent Technologies Inc. (Wilmington, DE, USA). A commercial suspension concentrate (SC) of diflubenzuron (24%; Difuse®, Agriphar, Belgium) and an emulsifiable concentrate (EC) of novaluron (10%, Equo®, Samtrtade, Spain) were purchased locally. High-purity deionized water (grade 1) was produced using the Ultra ClearTM system (Evoqua Water Technologies LLC, Guenzburg, Germany).
2.2. Standard solution preparation
The active ingredients diflubenzuron and novaluron were accurately weighed individually at 0.1005 and 0.1013 g in the dark, calibrated 100-mL volumetric flask, and dissolved in acetonitrile to obtain approximately 1000 mg. L-1 stock solution. A portion of each standard stock solution was diluted 10-fold in acetonitrile to give an intermediate standard solution of 100 mg. L−1. An equal volume of each intermediate standard solution was mixed and diluted in acetonitrile to obtain a working standard solution of 10 mg. L−1. The working standard solution was used to prepare serial concentrations to establish solvent and matrix calibration curves. All standard solutions were stored at −20°C.
2.3. Sample preparation
A representative 10 g of a homogenized frozen tomato was weighed to the nearest 0.2 g into 50 ml Polytetrafluoroethylene (PTFE) centrifuge tube, and 10 ml of extraction solvent MeCN was added, then the tube was vortexed for 1 min after adding a ceramic homogenizer to increase homogeneity between sample and solvent. For phase separation, a mixture of 4 g MgSO4 and 1 g NaCl salts was added, and then the tube was vortexed again for 30 seconds and centrifuged at 5000 rpm for 5 min. 500 µl of the upper organic upper was diluted 2-fold with acetonitrile. The diluted extract was vortexed for 30 seconds, then filtered through a syringe filter (0.22-micron) (Whatman, USA) for LC-MS/MS analysis.
2.4. LC-MS/Ms
Chromatographic separation was conducted on the Dionex Ultimate 3000 RS UHPLC (Thermo Fisher Scientific, Austin, TX, USA) using an Accucore RP-MS C18 column (150 × 2.1 mm, 2.6-μm film thickness, Thermo Fisher Scientific) at 40 °C, the analytes were eluted at 0.3 ml/min flow rate, and the injection volume was 2 µl. The mobile phase consisted of water (A) and acetonitrile (B). Initial gradient conditions were set at 50% B (held for 1 min), then increased to 90% (1–4 min), and held for 2 min (4–6 min), then returned to 50% and held for 5 min for column equilibration (6.1–11 min). The TSQ Altis triple quadrupole mass spectrometer (MS/MS, Thermo Fisher Scientific, Austin, TX, USA) was operated with an electrospray ionization source in positive mode (ESI+). The ion source and de-solvation temperatures were optimized to 325 °C and 350 °C, respectively. The capillary voltage was set to 3.8 kV. Sheath and auxiliary gasses were 40 and 10 Arb. Trace Finder software (version 4.1) was used for data acquisition and processing.
2.5. Method validation
In accordance with European Commission regulations (SANTE/12682/Citation2019), the effectiveness of the proposed procedure has been evaluated. The linearity, limit of detection (LOD), limit of quantitation (LOQ), precision, trueness (recovery), and the matrix effect (how the co-eluted matrix affected the analyte response) were studied. To investigate the linearity range, analytical curves were constructed using a standard solution of diflubenzuron and novaluron in pure solvent and a tomato matrix extract at a concentration range of 1 to 500 µg. L−1. The recovery percentage was studied by adding an appropriate volume (not more than 200 µl) of the standard solution to the homogenized tomato samples at spiking levels of 10, 100, and 1000 µg. kg−1. The method precision expressed as RSD was studied by analyzing six replicates of samples spiked at the concentration of LOQ on one day (intra-day repeatability, RSDr) and on different days (inter-day repeatability, n = 18, 5 days’ interval). The LOD was estimated and considered acceptable when a signal response of the detected analyte equivalent to three times the baseline noise was achieved (S/N = 3). The concentration at which the S/N ratios are 10, and achieved recovery and precision of 70–120% and ≤ 20%, respectively, was denoted as the limit of quantitation (LOQ). The matrix effect (ME %) was determined by comparing the slopes of the solvent- and matrix-matched calibration curves using EquationEquation 11
1 (Guedes et al., Citation2016).
2.6. Field experiments
The field experiments were conducted from March to May 2023 in Khubash City, Najran Region, southern Saudi Arabia. The application was conducted in four greenhouses (10 m × 40 m each). Each greenhouse was divided into five subplots (10 m × 8 m), three plots for each treatment, and two as buffers. Diflubenzuron (24%, SC, Difuse®) was applied separately at 192 and 336 g a.i. ha-1. Novaluron (10%, EC, Equo®) was applied at 40 and 80 g a.i. ha-1 at the fruiting stage. The tested pesticide formulations were diluted separately with water to 1000 L. ha−1 and applied using a knapsack sprayer. The dissipation pattern of the tested pesticides was investigated by randomly collecting samples (2–3 kg, n = 3) 0 (2 h), 1, 3, 5, 7, 10, 15, and 21 days after spraying the respective dose.
The terminal residue experiment was conducted by applying the tested formulations 2 and 3 times at 7-day intervals between treatments. On days 7, 10, and 15 after the last application of diflubenzuron, and on days 3, 7, and 10 after the final application of novaluron, tomato samples were taken for analysis. During the field experiment, relative humidity and average temperature varied between 38 to 79%, and 18 to 32 °C, respectively. All samples were immediately sent to the laboratory under controlled temperature conditions, homogenized (Stephan Universal UM5, Stephan Machinery GmbH, Hameln, Germany), and kept at − 20 ◦C before analysis.
2.7. Calculations
Dissipation kinetics were estimated using the exponential equation Ct=C0e−kt, where Ct is the residue concentration (mg.kg−1) at time t (days), C0 is the initial residue concentration (mg.kg−1), and the slope (k) is the rate constant (day−1). The half-life (t1/2) was calculated using the equation t1/2=ln 2/k. The pre-harvest interval (PHI) was calculated using the equation PHI =Ln (MRL/C0)/k (Abdallah et al., Citation2021; Hingmire et al., Citation2015; Hoskins, Citation1961).
2.8. Dietary exposure and risk assessment
Terminal residues of diflubenzuron and novaluron in tomatoes were used in a chronic dietary exposure assessment using EquationEquation 22
2 . Human health risks were characterized by comparing the national estimated dietary intake (NEDI) (mg/kg. bw) with the acceptable daily intake (ADI) (mg/kg.bw). The chronic health risk was calculated as RQc (chronic risk quotient) using EquationEquation 3
3
3 :
STMR is the supervised median residue from the field trial, F is the average food consumption (0.3197 kg. day−1), and body weight is the average for adults (60 kg). ADI (mg/kg.bw) is the acceptable daily intake level of 0.1 mg/kg.bw. day−1 for diflubenzuron (EFSA, Citation2012) and 0.01 mg/kg.bw. day−1 for novaluron (Anastassiadou et al., Citation2022). Residues of the tested insecticides in tomatoes are considered unsafe for human consumption if the percentage chronic risk quotient (%RQ) is greater than 100 (Abdallah et al., Citation2019a, Citation2021; Hlihor et al., Citation2016).
3. Results and discussions
3.1. LC-MS/MS optimization
The tested analytes were tuned for individual standard solutions at a concentration of 0.5 mg. L−1 in methanol/water (50/50, v/v) using a Harvard infusion pump (Harvard Apparatus, South Natick, MA, USA). The first step was the selection of the protonated [M+H]+ precursor ion for each analyte. The optimal cone voltage for the precursor ions and the optimal collision energy for the product ions were selected, with the transitions of the most abundant product ions chosen as a quantifier and those of the second most abundant as a qualifier. The precursor ions for diflubenzuron and novaluron were observed at 311.1 m/z and 493 m/z, respectively, in full scan mode. The positive ion spray voltages were automatically optimized. The values of 4 kV were the best for the positive voltages. The mass spectra obtained in the positive ESI mode contained several ions with high relative intensities, which gave good measurement selectivity (SANTE/12682/2019). Table shows the optimal conditions.
Table 1. Optimization conditions of ESI+-MS/MS for the analysis of diflubenzuron and novaluron
3.2. Method validation
3.2.1. Selectivity, linearity, and matrix effect
The instrument response values for the blank sample and pure solvent were less than 30% of the LOQs for the retention times of the target analytes, indicating that no interferences significantly affected the analysis, suggesting selectivity (Figure ). The linearity range of the instrument was evaluated over seven concentration levels from 1–500 µg. L−1 (corresponding to 2–1000 µg. kg−1). The dynamic range of diflubenzuron in solvent and matrix extracts was 1–50 µg. L−1 (corresponding to 2–100 µg. kg−1) with R2 of 0.9989 and 0.9992, respectively; for novaluron, the dynamic range was 2.5–250 µg. L−1 (equivalent to 5–500 µg. kg−1) with R2 of 0.9986 and 0.9994, respectively (Table ). Linearity was further tested by comparing the back-calculated concentrations of the standards with their actual values. All points examined met the criterion of a maximum deviation of 20%.
Figure 2. Chromatograms of blank tomato extract (A and C), and spiked tomato extract at 5 µg/kg for diflubenzuron (B) and 10 µg/kg for novaluron (D).
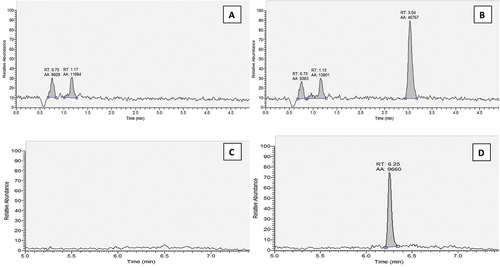
Table 2. Results of the validation parameters of diflubenzuron and novaluron in tomatoes
The matrix effect affects the qualitative and quantitative performance of the method. It affects precision and accuracy, increases the detection limit, and may affect the linearity of the method. In addition, it can lead to misleading results when the requirements are met and misleading results when they are not (Olejnik et al., Citation2013). The ME % is the signal suppression or enhancement of an analyte due to the co-extracted compounds (Abdallah et al., Citation2017); an enhancement (positive effect) in the responses of diflubenzuron and novaluron was observed at 4.33 and 2.24 %, respectively (Table ) and was classified as no matrix effect (Ferrer et al., Citation2011).
3.2.2. LOD and LOQ
The estimated value of LOD was 0.46 and 3.22 μg. Kg−1, and LOQ was 5 and 10 μg. Kg−1 for diflubenzuron and novaluron, respectively (Table ). The estimated LOQs were verified by the proposed method. They resulted in recoveries and precisions of 88.11% and 9.31% for diflubenzuron, and 90.55% and 6.63% for novaluron, respectively, which follow the SANTE/12682/2019 guideline requirements. The verified LOQ is twice the established MRL of 10 µg. kg−1 for diflubenzuron and corresponds to the MRL of 10 µg. kg−1 for novaluron in tomatoes. (EU-MRL).
Table 3. Recovery percent and RSDs (n = 6) of diflubenzuron and novaluron in tomatoes
3.2.3. Recovery and precision
The mean recoveries and relative standard deviation (RSD) of diflubenzuron and novaluron ranged from 90.55 to 97.51% and 3.74 to 8.22%, respectively (Table ). The method’s precision was studied at 5 and 10 µg. kg−1 spiking, respectively. The intraday precision (RSDr) of six replicate measurements on the same day (intraday repeatability) was 9.31 and 6.63%, respectively, while the interday repeatability (RSDR) measured on three different days (five days apart) (n = 18) was 13.72 and 8.87%, respectively (Table ).
According to the SANTE/12682/2019 guideline, the recovery rate should be between 70% and 120%, and the precision should be less than 20%. Both diflubenzuron and novaluron results met these criteria (SANTE/12682/2019).
3.3. Dissipation of diflubenzuron and novaluron in tomatoes
3.3.1. Diflubenzuron
The initial deposition of 0.192 mg. kg−1 and 0.318 mg. kg−1 after 2 hours from spraying the low authorized dose (192 g a.i. ha−1) and the high authorized dose (336 g a.i. ha−1), respectively, decreased to 0.151 and 0.221 mg. kg−1 by day 1, and 0.011 and 0.018 mg. kg−1 by day 21. The dissipation profile showed moderately rapid dissipation of residues from day 1 to day 7, with 21.3% and 75%, respectively, after applying the low authorized dose. After applying the high authorized dose, the dissipation rate was higher (30.5%) than the low authorized dose on day 1 and lower than the corresponding dose on day 7, with a dissipation rate of 70.75%. On days 15 and 21, after the application of both doses, the residues were almost close to the MRL value of 0.01 mg. kg−1 and <LOQ, respectively.
A linear relationship was obtained between log concentration (log c) and time (Figure ) with a co-efficient of determination (r2) of 0.9773 and 0.9792 for low and high authorized doses, respectively. Hence, the first-order model best describes the dissipation behaviour of diflubenzuron, which is consistent with López‐López et al. (Citation2004), who reported that the residue data of diflubenzuron after application to zucchini and peppers were mathematically consistent with the first-order model (López‐López et al., Citation2004).
Figure 3. Semi-logarithmic graph showing dissipation kinetics of diflubenzuron (A) and novaluron (B) in tomatoes (n=3).
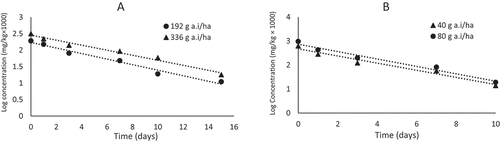
The exponential equation (EquationEquation 11
1 ) for the dissipation kinetics of diflubenzuron characterized by Ct = 0.1714 e−0.194t and Ct = 2689 e−0.154t, and the calculated half-lives (t0.5) after application of the authorized low and high doses were 3.6 and 4.5 days, respectively (Table ).
Table 4. Dissipation kinetics of diflubenzuron and novaluron in/on tomatoes
3.3.2. Novaluron
The initial deposition of novaluron was 0.627 and 0.964 mg. kg−1 in tomato fruits. The residues highly dissipated by 54.5% and 55.5% after one day of applying the authorized (40 g a.i. ha−1) and double authorized (80 g a.i. ha−1) doses, respectively. On the 3rd and 7th days, the residues decrease to more than 80 and 90% after application of both doses, then to more than 98% on the 10th day after application. On the 15th day, the residues were less than 0.01 mg. kg−1 (<LOQ).
The results showed that the dissipation kinetics of novaluron in tomatoes was suitable and characterized by a simple first-order kinetic model. The co-efficient of determination (r2) was 0.9644 and 0.9714 after applying the authorized and double-authorized doses, respectively (Figure ).
The exponential equation obtained as the relationship between residue concentration (mg. K−1) as a function of time (t) was Ct = 0.4729e−0.342t (after applying the authorized dose of 40 g a.i. ha−1), and Ct = 0.7413e−0.356t (after application of double the authorized dose of 80 g a.i. ha−1) (Table ), and the calculated half-life (t0.5) was 1.77 and 1.73 days, respectively.
Many factors affect pesticide dissipation, including the physical and chemical properties of the pesticide, such as vapour pressure and water solubility, and plant-specific characteristics, such as growth rate, plant morphology, and microbial activity, in addition to environmental factors, such as rainfall, temperature, and duration of direct sunlight (Abdallah et al., Citation2019b, Citation2021, Citation2019a, Citation2023, Citation2023; Algethami et al., Citation2022; Fantke & Juraske, Citation2013). The Octanol-Water partition coefficients (log Kow) of diflubenzuron and novaluron are 3.89 (MacBean, Citation2008–2010) and 5.25 (Tomlin, Citation1997), respectively, indicating that both insecticides are highly hydrophobic. Tomatoes have a waxy exterior that allows the sprayed pesticide to be absorbed so that it slowly dissipates. It was also found that the rate of dissipation of novaluron (k = 0.342–0.356) was faster than that of diflubenzuron (k = 0.154–0.194), which can be interpreted by the high value of vapour pressure of novaluron (3.75 × 10−6 mmHg, at 40 °C) compared to diflubenzuron (9 × 10−10 mmHg, at 25 °C). From the results, the difference in the initial deposition of the tested insecticides is clear, which can be explained by the difference in the concentration of the applied dose and the difference in the type of formulation, which can also play an essential role in the dissipation behaviour. In this study, it is hypothesized that the observed significant difference between the half-life of diflubenzuron and novaluron in tomato fruits may not be due to the effect of dilution during plant growth because both pesticides were applied at the same time and the same stage of fruiting (Fenoll et al., Citation2008), and in the absence of rainfall. Previous studies reported half-lives of diflubenzuron: 25 and 9 days in peaches when applied in 2015 and 2016, respectively (Dong et al., Citation2019), 1.4 days in strawberries (Chang et al., Citation2020), 2.5 and 1.8 days in cucumbers, 7.3 and 8.2 days in shallots (Hyeong Wook Jo & Kyuwon, Citation2020), 2.35–7.56 days in peppers, and 2.78 days in zucchini (López‐López et al., Citation2004). Studies on tomatoes show that novaluron has a short half-life of 2.05 days (Malhat et al., Citation2014); for chilli, the half-life was 1.80–1.95 days, and for brinjal, 1.8–2.08 days (Das, Citation2007), which is consistent with our study. However, the half-life of novaluron in citrus was quite long at 28.9 days (Dong et al., Citation2018). These differences may be partly due to plant variety, application doses, local environment, and differences in plant growth.
The MRL for diflubenzuron and novaluron in tomatoes in Saudi Arabia has yet to be established. A maximum residue limit (MRL) for diflubenzuron and novaluron in tomatoes has yet to be established by Codex (WHO/FAO) and the European Union. In the European Union regulation, the MRL for the two insecticides was set at 0.01 mg.kg−1 as the limit of determination. The residues of diflubenzuron and novaluron were below the LOQ level of 0.005 and 0.01 mg.kg−1, respectively, 21 days and 15 days after applications of the authorized dose and high dose, respectively. The pre-harvest interval (PHI) was calculated more consistently using Equation 4. The calculated PHI are 14.6 and 17.8 days for diflubenzuron and 11.2 and 12.1 days for novaluron (Table ), which is safe for consumers and does not hinder international trade.
3.4. Final residues
Residues of diflubenzuron and novaluron in tomato fruit after 2 and 3 times application of the authorized dose and high dose are shown in Table . Residues of diflubenzuron in tomato samples taken 7, 10, and 15 days after treatment ranged from 0.048 to 0.328, 0.054 to 0.131, and 0.013 to 0.032 mg. kg−1, respectively. In contrast, residues of novaluron in samples taken after 3, 7, and 10 days ranged from 0.064 to 0.17, 0.036 to 0.083, and 0.011 to 0.021 mg kg−1, respectively. The residue levels of diflubenzuron and novaluron in tomatoes were above the EU MRL of 0.01 when diflubenzuron was harvested at 7, 10, and 15 days and novaluron were harvested at 3, 7, and 10 days, while they were below the LOQs of 0.005 and 0.01 mg. kg−1 at 21 and 15 days, respectively, which are below the EU MRL of 0.01 mg. Kg−1. As far as we know, diflubenzuron and novaluron can be applied to tomatoes in Saudi Arabia, and the Saudi authorities have not established a maximum residue limit for tomatoes. Therefore, the above data and the EU-MRL of 0.01 mg. kg−1 could be used as a reference for the Saudi government to set the maximum residue limit for diflubenzuron and novaluron in tomatoes, and the dietary risk of the two insecticides in tomatoes could be evaluated based on the final residue results.
Table 5. Terminal residues and chronic risk assessment of diflubenzuron and novaluron in tomato fruits
3.5. Risk assessment
The acute risk exposure calculation was skipped because an acute reference dose (ARfD) was unnecessary for diflubenzuron and novaluron (FAO, Citation2001; EFSA, Citation2012). Comparison of the NEDI (national estimated daily intake) and ADI (acceptable daily intake) for both insecticides based on terminal residue data from field trials allowed a risk assessment for long-term consumption of diflubenzuron and novaluron (Table ). According to the GEMS/Food regional diet (cluster diet G04) (WHO, Citation2003) and assuming the worst case that 100% of the fruits contain residues, a daily intake of tomato of 0.3197 kg/adult was considered for the calculation of NEDI. Adults’ supposed body weight is 60 kg (Ambrus, Citation2009). The ADI of diflubenzuron and novaluron were 0.1 and 0.01 mg.kg−1 bw, respectively, based on the European Food Safety Authority (EFSA, Citation2012). The NEDI values were calculated based on the STMR and considering the worst-case scenario of high residue levels using Equation 5. According to the STMR values, the NEDI value for diflubenzuron and novaluron was estimated to be less than 1.14E–03 mg.kg−1 and 6.07E–04 mg.kg−1, respectively. High RQ values indicate higher pesticide residue levels. A %RQ value greater than 100 poses an unacceptably high health risk to the consumer (Damalas & Eleftherohorinos, Citation2011). The RQ of diflubenzuron and novaluron for Saudi consumers was predicted to be 1.14 and 6.074 %, respectively. Therefore, the residues of diflubenzuron and novaluron in tomatoes will not cause significant potential health risks to consumers after applying 24% SC and 10% EC at the authorized and high doses.
4. Conclusions
A simple, rapid, highly accurate, and precise method for determining diflubenzuron and novaluron residues in tomato fruits was developed and validated. The method includes three main steps: liquid-liquid extraction (LLE) with acetonitrile, salting-out for phase separation with MgSO4 and NaCl (4:1 w/w), and quantification with LC-MS/MS. This is the first work to provide a dissipation profile for the two insecticides studied in greenhouse tomatoes. Also, the risk of dietary exposure to diflubenzuron and novaluron in tomatoes has not been evaluated in previous reports, although this is important for risk monitoring. The validated method was used to investigate the dissipation behaviour of diflubenzuron (24% SC) and novaluron (10% EC) in tomatoes after application of the authorized and high doses under greenhouse conditions in Najran, Saudi Arabia. The field trials showed that novaluron dissipated faster than diflubenzuron at both applied doses. Residue concentrations of diflubenzuron and novaluron were below the EU-MRL value of 0.01 mg.kg−1 at 21 and 15 days after application. The calculated risk quotient (RQ) was well below 100%, indicating that the residues of diflubenzuron and novaluron in tomatoes do not pose a risk to Saudi consumers.
Highlights
What does the current manuscript add to the existing knowledge? (Research Highlights)
(1) Diflubenzuron and novaluron have been determined in tomatoes using a developed and validated method.
(2) The dissipation of diflubenzuron and novaluron in tomatoes showed strong first-order kinetics.
(3) The half-lives of diflubenzuron and novaluron in tomatoes and the pre-harvest interval (PHI) are proposed.
(4) The terminal residues and risk assessment were studied in tomatoes
(5) This study could help Saudi Arabia to establish MRLs for diflubenzuron and novaluron in tomatoes.
Author contributions
Osama I. Abdallah contributed to the conception and design of the experiment and wrote the manuscript. Osama I. Abdallah and Mohamed F. Ramadan collected the real samples and performed the analysis. Jari S. Algethami and Mohsen A. M. Alhamami, Amal F. Seliem, and Yaser A. Alnaam performed the data analysis and revision of the manuscript.
Disclosure statement
No potential conflict of interest was reported by the author(s).
Additional information
Funding
References
- Abdallah, O., Abdel Ghani, S., & Hrouzková, S. (2017). Development of validated LC-MS/MS method for imidacloprid and acetamiprid in parsley and rocket and evaluation of their dissipation dynamics. Journal of Liquid Chromatography & Related Technologies, 40(8), 392–13. https://doi.org/10.1080/10826076.2017.1310112
- Abdallah, O. I., Abd El-Hamid, R. M., & Raheem, E. H. A. (2019a). Clothianidin residues in green bean, pepper and watermelon crops and dietary exposure evaluation based on dispersive liquid-liquid microextraction and LC–MS/MS. Journal of Consumer Protection and Food Safety, 14(3), 293–300. https://doi.org/10.1007/s00003-019-01218-4
- Abdallah, O., El Agamy, M., Abdelraheem, E., & Malhat, F. (2019b). Buprofezin dissipation and safety assessment in open field cabbage and cauliflower using GC/ITMS employing an analyte protectant. Biomedical Chromatography, 33(6), e4492. https://doi.org/10.1002/bmc.4492
- Abdallah, O., Soliman, H., El-Hefny, D., Abd El-Hamid, R., & Malhat, F. (2021). Dissipation profile of sulfoxaflor on squash under Egyptian field conditions: A prelude to risk assessment. International Journal of Environmental Analytical Chemistry, 103(16), 1–15. https://doi.org/10.1080/03067319.2021.1915297
- Abdallah, O. I., Abd El-Hamid, R. M., Ahmed, N. S., Saleh, S. M., & Alminderej, F. M. (2023). Terminal residues and risk assessment of Spiromesifen and Spirodiclofen in tomato fruits. Plants, 12(7), 1493. https://doi.org/10.3390/plants12071493
- Abdallah, O. I., Ahmed, N. S., Abd El-Hamid, R. M., & Alhewairini, S. S. (2023). Residues of difenoconazole in various ready premixes with propiconazole, cyflufenamid, and mandipropamid in/on tomato fruits. Acta Chromatographica. https://doi.org/10.1556/1326.2023.01134
- Algethami, J. S., Alhamami, M. A., Ramadan, M. F., & Abdallah, O. I. (2022). Residues of the acaricides abamectin, Hexythiazox, and Spiromesifen in eggplant (Solanum melongena L.) fruits grown under field conditions in Najran, Saudi Arabia. Agriculture, 13(1), 116. https://doi.org/10.3390/agriculture13010116
- Ambrus, Á. (2009). Submission and evaluation of pesticide residues data for the estimation of maximum residue levels in food and feed. Food and Agriculture Organization of the United Nations.
- Anastassiadou, M., Bellisai, G., Bernasconi, G., Brancato, A., Cabrera, L. C., Ferreira, L., Greco, L., Jarrah, S., Kazocina, A., & Leuschner, R. (2022). Review of the existing maximum residue levels for novaluron according to article 12 of Regulation (EC) no 396/2005. The EFSA Journal, 20(1). https://doi.org/10.2903/j.efsa.2022.7041
- Arrebola, F. J., Egea‐González, F. J., Moreno, M., Fernández‐Gutiérrez, A., Hernández‐Torres, M. E., & Martínez‐Vidal, J. L. (2001). Evaluation of endosulfan residues in vegetables grown in greenhouses. Pest Management Science: Formerly Pesticide Science, 57(7), 645–652. https://doi.org/10.1002/ps.332
- Carvalho, J. D., & Pagliuca, L. G. (2007). Tomate, um mercado que não para de crescer globalmente. Hortifruti Brasil, 6(58), 6–14.
- Chang, H.-R., You, J.-S., & Ban, S.-W. (2020). Residue dissipation kinetics and safety evaluation of insecticides on Strawberry for the harvest periods in plastic-covered greenhouse conditions. Korean Journal of Environmental Agriculture, 39(2), 122–129. https://doi.org/10.5338/KJEA.2020.39.2.16
- Chen, L., Chen, J., Guo, Y., Li, J., Yang, Y., Xu, L., & Fu, F. (2014). Study on the simultaneous determination of seven benzoylurea pesticides in oolong tea and their leaching characteristics during infusing process by HPLC–MS/MS. Food Chemistry, 143, 405–410. https://doi.org/10.1016/j.foodchem.2013.08.027
- Damalas, C. A., & Eleftherohorinos, I. G. (2011). Pesticide exposure, safety issues, and risk assessment indicators. International Journal of Environmental Research and Public Health, 8(5), 1402–1419. https://doi.org/10.3390/ijerph8051402
- Das, P. (2007). Dissipation of novaluron in chilli and brinjal. ANSINET.
- Dong, M., Ma, L., Zhan, X., Chen, J., Huang, L., Wang, W., & Zhao, L. (2019). Dissipation rates and residue levels of diflubenzuron and difenoconazole on peaches and dietary risk assessment. Regulatory Toxicology and Pharmacology, 108, 104447. https://doi.org/10.1016/j.yrtph.2019.104447
- Dong, M., Wen, G., Tang, H., Wang, T., Zhao, Z., Song, W., Wang, W., & Zhao, L. (2018). Dissipation and safety evaluation of novaluron, pyriproxyfen, thiacloprid and tolfenpyrad residues in the citrus-field ecosystem. Food Chemistry, 269, 136–141. https://doi.org/10.1016/j.foodchem.2018.07.005
- EFSA, European Food Safety Authority. (2012). Conclusion on the peer review of the pesticide risk assessment of confirmatory data submitted for the active substance diflubenzuron. The EFSA Journal, 10(9), 2870. https://doi.org/10.2903/j.efsa.2012.2870
- Emekli, N. Y., Kendirli, B., & Kurunc, A. (2010). Structural analysis and functional characteristics of greenhouses in the Mediterranean region of Turkey. African Journal of Biotechnology, 9(21), 3131–3139.
- EU-MRL. European Union Commission regulations for maximum residue limits of pesticides in foods and feeds. https://ec.europa.eu/food/plant/pesticides/eu-pesticides-database/start/screen/mrls. (Accessed on 19/6/2023).
- Fantke, P., & Juraske, R. (2013). Variability of pesticide dissipation half-lives in plants. Environmental Science & Technology, 47(8), 3548–3562. https://doi.org/10.1021/es303525x
- FAO (Food and Agriculture Organization of the United Nations). (2001). Pesticide residues in food—2001. In report of the joint meeting of the FAO Panel of experts on pesticide residues in food and the Environment and the WHO Core Assessment Group on pesticide residues. Food and Agricultural Organization.
- FAOSTAT. Food and Agriculture Organization of the United Nations. Retrieved August 25, 2023 Available at http://www.fao.org/faostat/en/#data/QC.
- Fenoll, J., Hellín, P., Camacho, M. D. M., Lopez, J., González, A., Lacasa, A., & Flores, P. (2008). Dissipation rates of procymidone and azoxystrobin in greenhouse grown lettuce and under cold storage conditions. International Journal of Environmental and Analytical Chemistry, 88(10), 737–746. https://doi.org/10.1080/03067310801975118
- Ferrer, C., Lozano, A., Agüera, A., Girón, A. J., & Fernández-Alba, A. (2011). Overcoming matrix effects using the dilution approach in multiresidue methods for fruits and vegetables. Journal of Chromatography A, 1218(42), 7634–7639. https://doi.org/10.1016/j.chroma.2011.07.033
- Grosscurt, A., & Jongsma, B. (1987). Mode of action and insecticidal properties of diflubenzuron (chitin and benzoylphenyl ureas. Springer.
- Guedes, J. A. C., de Oliveira Silva, R., Lima, C. G., Milhome, M. A. L., & Do Nascimento, R. F. (2016). Matrix effect in guava multiresidue analysis by QuEChERS method and gas chromatography coupled to quadrupole mass spectrometry. Food Chemistry, 199, 380–386. https://doi.org/10.1016/j.foodchem.2015.12.007
- Hingmire, S., Oulkar, D. P., Utture, S. C., Shabeer, T. A., & Banerjee, K. (2015). Residue analysis of fipronil and difenoconazole in okra by liquid chromatography tandem mass spectrometry and their food safety evaluation. Food Chemistry, 176, 145–151. https://doi.org/10.1016/j.foodchem.2014.12.049
- Hlihor, R. M., Pogăcean, M. O., Simion, I. M., Cozma, P., Apostol, L. C., & Gavrilescu, M. (2016). Assessment of human health risk of twelve pesticides applied in double dose in an apple orchard. Annals of the Academy of Romanian Scientists Series on Physics and Chemistry, 1(1), 25–35.
- Hoskins, W. (1961). Mathematical treatment of the rate of loss of pesticide residues. FAO Plant Protection Bulletin, 9(163168), 214–215.
- Hyeong Wook Jo, K. H., & Kyuwon, J. K. M. (2020). Dissipation pattern of Insecticide diflubenzuron on cucumber and shallot. The Korean Journal of Pesticide Science, 24(3), 304–311. https://doi.org/10.7585/kjps.2020.24.3.304
- IRAC, Insecticide Resistance Action Committee. (2011). Tuta absoluta the tomato leafminer or tomato borer. Recommendations for Sustainable and Effective Resistance Management Insecticide Resistance Management.
- Jankowska, M., Kaczynski, P., Hrynko, I., & Lozowicka, B. (2016). Dissipation of six fungicides in greenhouse-grown tomatoes with processing and health risk. Environmental Science and Pollution Research, 23(12), 11885–11900. https://doi.org/10.1007/s11356-016-6260-x
- Katsoulas, N., Boulard, T., Tsiropoulos, N., Bartzanas, T., & Kittas, C. (2012). Experimental and modelling analysis of pesticide fate from greenhouses: The case of pyrimethanil on a tomato crop. Biosystems Engineering, 113(2), 195–206. https://doi.org/10.1016/j.biosystemseng.2012.07.007
- Keith, L. H., & Walker, M. (1992). Epa’s pesticide fact sheet database. CRC Press.
- López‐López, T., Martínez‐Vidal, J. L., Gil‐García, M. D., Martínez‐Galera, M., & Rodríguez‐Lallena, J. A. (2004). Benzoylphenylurea residues in peppers and zucchinis grown in greenhouses: determination of decline times and pre‐harvest intervals by modelling. Pest Management Science: Formerly Pesticide Science, 60(2), 183–190. https://doi.org/10.1002/ps.812
- MacBean, C. E. Eds 2008-2010. E-pesticide manual 15th ver. 5.1 British Crop Protection Council. Diflubenzuron (35367-38-5)
- Malhat, F. M., Loutfy, N. M., & Ahmed, M. T. (2014). Dissipation kinetics of novaluron in tomato, an arid ecosystem pilot study. Toxicological & Environmental Chemistry, 96(1), 41–47. https://doi.org/10.1080/02772248.2014.911538
- Miliadis, G. E., Tsiropoulos, N. G., & Aplada-Sarlis, P. G. (1999). High-performance liquid chromatographic determination of benzoylurea insecticides residues in grapes and wine using liquid and solid-phase extraction. Journal of Chromatography A, 835(1–2), 113–120. https://doi.org/10.1016/S0021-9673(99)00053-9
- Nakagawa, Y., Matsumura, F., & Hashino, Y. (1993). Effect of diflubenzuron on incorporation of [3H]-N-acetylglucosamine ([3H] NAGA) into chitin in the intact integument from the newly molted American cockroach Periplaneta americana. Comparative Biochemistry and Physiology Part C: Pharmacology, Toxicology and Endocrinology, 106(3), 711–715. https://doi.org/10.1016/0742-8413(93)90231-9
- Nougadère, A., Reninger, J.-C., Volatier, J.-L., & Leblanc, J.-C. (2011). Chronic dietary risk characterization for pesticide residues: A ranking and scoring method integrating agricultural uses and food contamination data. Food and Chemical Toxicology, 49(7), 1484–1510. https://doi.org/10.1016/j.fct.2011.03.024
- Olejnik, M., Jedziniak, P., Szprengier‐Juszkiewicz, T., & Żmudzki, J. (2013). Influence of matrix effect on the performance of the method for the official residue control of non‐steroidal anti‐inflammatory drugs in animal muscle. Rapid Communications in Mass Spectrometry, 27(3), 437–442. https://doi.org/10.1002/rcm.6467
- SANTE/12682/2019. Guidance document on analytical quality control and method validation procedures for pesticides residues analysis in food and feed. https://ec.europa.eu/food/sites/food/files/plant/docs/pesticides_mrl_guidelines_wrkdoc_2019-12682.pdf.
- Sharma, K. K., Tripathy, V., Mohapatra, S., Matadha, N. Y., Pathan, A. R. K., Sharma, B. N., Dubey, J. K., Katna, S., George, T., Tayade, A., Sharma, K., Gupta, R., & Walia, S. (2021). Dissipation kinetics and consumer risk assessment of novaluron+ lambda-cyhalothrin co-formulation in cabbage. Ecotoxicology and Environmental Safety, 208, 111494. https://doi.org/10.1016/j.ecoenv.2020.111494
- Si, W., Wang, T., Dong, M., Zhao, L., Wang, W., Zhao, Z., & Song, W. (2017). Assessment of safety for diflubenzuron residues in peach fruit after application in field. Acta Agriculturae Shanghai, 33(3), 71–75.
- Tomlin, C. (1997). The pesticide manual 11th. British Crop Protection Council.
- Tsiropoulos, N. G., Aplada-Sarlis, P. G., & Miliadis, G. E. (1999). Determination of benzoylurea insecticides in apples and pears by solid-phase extraction cleanup and liquid chromatography with UV detection. Journal of AOAC International, 82(1), 213–216. https://doi.org/10.1093/jaoac/82.1.213
- Valverde Garcia, A., Gonzalez-Pradas, E., & Aguilera-Del Real, A. (1993). Analysis of buprofezin residues in vegetables. Application to the degradation study on eggplant grown in a greenhouse. Journal of Agricultural and Food Chemistry, 41(12), 2319–2323. https://doi.org/10.1021/jf00036a019
- Valverde Garcia, A., Gonzalez-Pradas, E., Aguilera-Del Real, A., Ureña-Amate, M., & Camacho-Ferre, F. (1993). Determination and degradation study of chlorothalonil residues in cucumbers, peppers and cherry tomatoes. Analytica chimica acta, 276(1), 15–23. https://doi.org/10.1016/0003-2670(93)85034-H
- VSS, T., Patil, P., & Kannan, K. (2020). Pesticide Residue in Mango Orchards and health risk. Acta scientific microbiology, 3(9), 08–14.
- WHO. (2003). Gems/food regional diets (regional per capita consumption of raw and semi‐processed agricultural commodities). Global Environment Monitoring System/Food Contamination Monitoring and Assessment Programme. https://iris.who.int/handle/10665/42833
- Wimmer, M. J., Smith, R. R., & Jones, J. P. (1991). Analysis of diflubenzuron by gas chromatography/mass spectrometry using deuterated diflubenzuron as internal standard. Journal of Agricultural and Food Chemistry, 39(2), 280–286. https://doi.org/10.1021/jf00002a012
- Yalçın, M., Turgut, N., Gökbulut, C., Mermer, S., Sofuoğlu, S. C., Tari, V., & Turgut, C. (2023). Removal of pesticide residues from apple and tomato cuticle. Environmental Science and Pollution Research, 30(6), 15821–15829. https://doi.org/10.1007/s11356-022-23269-1