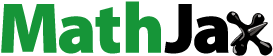
Abstract
Recent progress in the field of ultra-high energy cosmic rays has been driven by the data collected by the Pierre Auger Observatory in the southern hemisphere, and also by the Telescope Array in the northern hemisphere. These hybrid observatories combine large ground arrays with fluorescence detection techniques. In this paper, selected results will be reviewed. Future challenges and prospects will be briefly addressed.
Public Interest Statement
Ultra-high energy cosmic rays are the highest energy particles in the universe. They are rare window to the most energetic astrophysical phenomena, and open questions remain concerning their nature, origin and acceleration mechanisms. Knowing more about ultra-high energy cosmic rays will bring a better understanding of the most extreme objects and phenomena in the Universe. The very low flux of ultra-high energy cosmic rays requires huge detection areas. The largest detectors currently in operations are the Pierre Auger Observatory, in the southern hemisphere, with an area of 3,000 km, and the Telescope Array, in the northern hemisphere, covering 700 km
. Present results and future prospects in the field are discussed in this article.
1. Introduction
Ultra-high energy cosmic rays are a unique window to the most energetic phenomena in the universe and to particle interactions at energies well above human-made accelerators. Recent progress on the field of ultra-high energy cosmic rays has been driven by the data collected by the Pierre Auger Observatory (Auger) in the southern hemisphere and also by the Telescope Array (TA) in the northern hemisphere. In this paper, selected results of the Pierre Auger Observatory are presented and, in some cases, compared with TA results. Given the theme of this symposium, any possible links to the dark matter quest will be mentioned. Finally, some comments on the future challenges and prospects in the field will be added.
2. The observatories
Present-day ultra-high energy cosmic ray observatories are hybrid detectors combining large ground arrays with fluorescence telescopes watching the atmosphere above the arrays. Fluorescence telescopes are sensitive to the electromagnetic part of the cosmic ray air showers and provide a direct measurement of their longitudinal development profiles, which are directly related to the shower energy. Ground detectors are sensitive to both the electromagnetic and muonic shower components (in proportions that depend on the specific detection technique used). Combining the measurement of signal intensities and arrival times in the different stations, the shower lateral profile at ground level is obtained. Surface detector (SD) arrays, with a 100% duty cycle, are the most effective way to increase the amount of data collected. Fluorescence detectors (FDs), on the other hand, provide a rather direct calorimetric measurement of the shower energy, allowing for a data calibration of the SDs.
The Pierre Auger Observatory (Aab et al., The Pierre Auger Collaboration, CitationXXXX) covers an area of approximately 3,000 km in the Pampa Amarilla, province of Mendoza, Argentina. The observatory is located at a latitude of 35.3
S and an altitude of 1,400 m. The Auger SD is composed of 1,660 water-Cherenkov tanks disposed in a triangular lattice of 1.5 km. The observatory FD has four sites at the borders of the array viewing its full area. The Observatory has been in operation since 2004.
TA (Tokuno, The Telescope Array Collaboration, Citation2012) covers an area of about 700 km in the Utah desert, USA. The observatory is located at a latitude of 39.4
N and an altitude of 1,400 m. It is composed of scintillation detectors placed in a square 1.2 km grid and surrounded by fluorescence telescopes located in three sites.
3. Selected results
3.1. Energy spectrum
One decade ago, the discrepancy between the Hires and AGASA experiments brought much attention to the upper energy end of the cosmic ray spectrum. The fact that AGASA did not seem to observe the expected Greisen–Zatsepin–Kuzmin cut-off (GZK), due to the interaction of the highest energy protons with the cosmic microwave background, opened the way to a large number of exotic scenarios, in particular those related to super heavy dark matter (SHDM). Most of these scenarios are today excluded or highly disfavoured.
In fact, Auger has unambiguously established the existence of a suppression on the cosmic ray spectrum at the highest energies and accurately measured the ankle region. The energy spectrum as measured by Auger is shown in Figure . The comparison with the measurements of other experiments, in particular TA, is also shown. More details on the energy spectrum measurement can be found in Schulz, for the Pierre Auger Collaboration (Citation2013).
In what concerns the comparison with TA (Tinyakov, for the Telescope Array Collaboration, Citation2014), there is a very good agreement in the ankle region if a shift of 10% in energy is allowed for. A difference of the order of 50% in the cut-off position and shape is observed and is under investigation (Dawson et al., for the Pierre Auger, Telescope Array and Yakutsk Collaborations, Citation2013; Matthews, for the Pierre Auger and Telescope Array Collaborations, Citation2013).
In spite of this well-established result, the measurement of the spectrum alone does not allow one to separate a GZK-like effect from the exhaustion of the sources. This will remain as a challenge for the future, requiring further statistics and composition measurements.
Figure 1. Top: The energy spectrum as measured by Auger (the numbers show the number of events in each energy bin); Bottom: The Auger energy spectrum is compared with the measurements of other experiments, in particular TA (see Schulz, for the Pierre Auger Collaboration, Citation2013 for details).
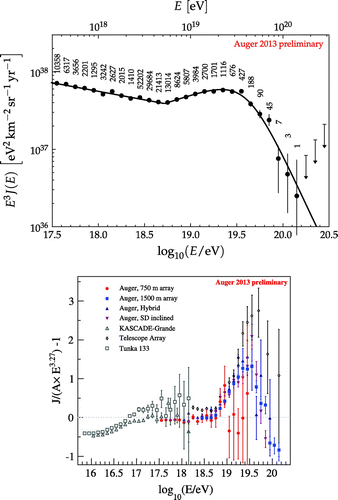
3.2. Arrival directions
The arrival directions of the highest energy cosmic rays are key to the understanding of the acceleration mechanisms of these particles at the far end of the spectrum, and to open a window to the most extreme objects and processes in our universe. Details on the different anisotropy searches conducted in Auger can be found in Aab et al., The Pierre Auger Collaboration (Citation2015).
Overall, the distribution of arrival directions is found to be remarkably isotropic. At the highest energies and for a small angular correlation window ( EeV,
, angular distance
), a small excess with a significance of about 2.5
is observed in the correlation with directions of active galactic nuclei galaxies (AGN). This is driven by Centaurus-A, the nearest AGN. The evolution of the number of AGN correlating events as a function of the total number of events, ordered by energy, is shown in Figure . TA has recently announced a “hot spot” excess (below 5
) in the northern sky, in a region not seen by Auger and not clearly associated with a known object (Abbasi et al., The Telescope Array Collaboration, Citation2014).
Figure 2. Number of event directions correlating with AGN as a function of the total number of events ordered by energy. The 1, 2 and 3 bands are also shown (Selvon for the Pierre Auger Collaboration, Citation2014).
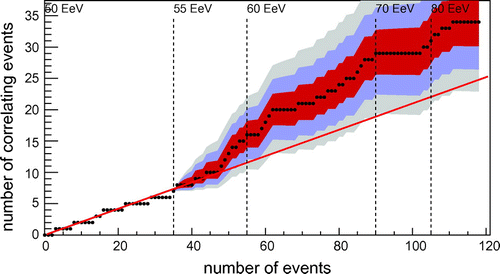
Searches for anisotropies in the large-scale distribution of the sources, in the form of dipoles and quadrupoles, have also been performed. In particular, strong bounds have been placed on densely and uniformly distributed sources in the galactic plane emitting in all directions. As a general comment, we can say that only larger statistics will allow one to settle these issues and to really examine the location and spectra of the sources.
3.3. Search for photon primaries
Photons would be particularly appealing ultra-high energy cosmic ray primaries for a number of reasons. First, in this energy range, the universe becomes again more transparent to photons. Also, they would point to their sources at all energies. In addition to that, photon primaries would provide clues on whether we are really observing the GZK cut-off or simply the exhaustion of sources. Finally, photons arise in a number of exotic scenarios, which include Lorentz invariance violation and also dark matter models such as SHDM.
Figure 3. Current limits on the flux of primary photons are shown, together with the prediction for cosmogenic (GZK) and different types of exotic models (Abraham et al., The Pierre Auger Collaboration, Citation2009).
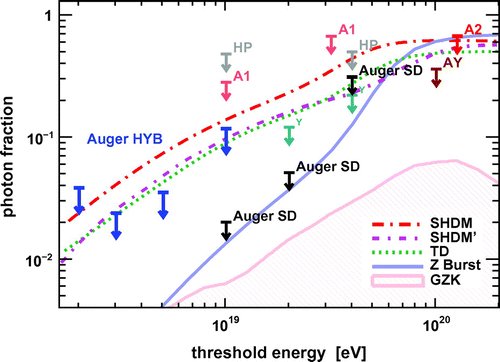
In Auger, primary photons are searched for combining the information collected by the FD and SD detectors. While the FD provides information based on the depth of the shower maximum, the SD provides larger statistics and information based on the lateral profile of the shower and on variables related to the shower front shape. The Auger limits on photon primaries are given in Figure . Top-down models are highly disfavoured, and the sensitivity levels are now approaching the most optimist predictions of cosmogenic photon fluxes. Further details can be found in Abraham et al., The Pierre Auger Collaboration (Citation2009). A recent analysis searching for photon sources rather than a diffuse photon flux is described in Kuempel, for the Pierre Auger Collaboration (Citation2013). No source was identified in this analysis.
3.4. Composition
Composition studies in ultra-high energy cosmic ray detectors are to a large extent based on the fluorescence measurement of the depth in the atmosphere at which the shower reaches its maximum, . The results published by Auger on both
and its RMS are shown in Figure and show a trend towards heavier primaries at the highest energies, when interpreted according to currently available hadronic interaction models.
Detailed fits to these data yield a preferred composition scenario which is a mixture of intermediate mass elements such as helium and nitrogen, disfavouring iron, and with a proton fraction that decreases with energy—and might reappear in the last energy bin, but the statistics is really not enough to tell. This conflicts with the expectations of most current astrophysics scenarios. More details on the Auger composition analyses can be found in Aab et al., The Pierre Auger Collaboration (Citation2014) and de Souza, for the Pierre Auger Collaboration (Citation2013). TA results are compatible with a proton scenario in all energy ranges (Tinyakov, for the Telescope Array Collaboration, Citation2014). However, the statistics are low and part of the difference could be related to the models and analysis techniques rather than to data (Hanlon, for the Telescope Array and Pierre Auger Collaborations, Citation2013). A recent analysis in fact demonstrates that TA results are not incompatible with the Auger interpretation (Abbasi et al., for the Telescope Array and Pierre Auger Collaborations, Citation2014).
Figure 4. Depth of shower maximum: average value (left) and RMS (right). Auger results are shown together with model expectations (Aab et al., The Pierre Auger Collaboration, Citation2014 ; de Souza, for the Pierre Auger Collaboration, Citation2013).
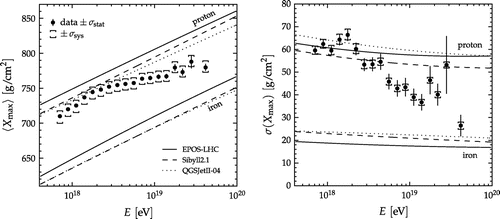
Alternatively, interpreting these data as proton-only at all energies leads to the prediction of a sudden increase in proton–air cross section at the highest energies. The study of the muon component in air showers is a key aspect to extend composition studies. The combination of the information with the muon content of the shower would appreciably improve the discriminating power on the nature of the primary. On the other hand, muon content measurements are based on the SD, and this would allow one to increase the available statistics.
With the muon production depth technique, the depth at which the muonic shower reaches its maximum has been measured by Auger (Garcia-Gamez, for the Pierre Auger Collaboration, Citation2013). The results show the same trend as the electromagnetic , with somewhat heavier primaries favoured at the highest energies. Measurements of the muon content of the shower with respect to model expectations have also been performed (Farrar, for the Pierre Auger Collaboration, Citation2013; Kegl, for the Pierre Auger Collaboration, Citation2013; Valiño, for the Pierre Auger Collaboration, Citation2013).
As shown in Figure , none of the available hadronic models predicts enough muons to match the current measurements. This is a puzzling and interesting observation telling us that, besides composition, there are differences in the interactions at such high energies with respect to current models. One of the difficulties of such measurements is the fact that ground detectors measure a combination of the electromagnetic and muonic shower components, and systematics are large when trying to disentangle the two. An upgrade of the detectors with the goal of reaching a better separation of the electromagnetic and muonic shower components would be crucial for these studies.
Figure 5. Muonic () and electromagnetic (
) shower sizes normalized to the prediction of different models for 10
eV showers (Farrar, for the Pierre Auger Collaboration, Citation2013).
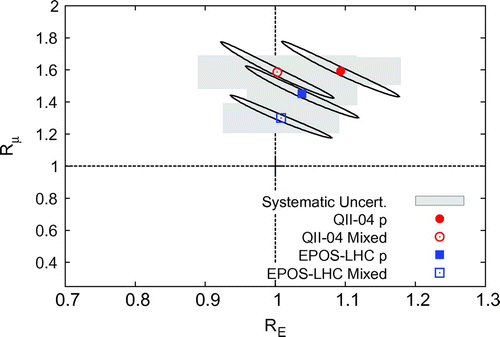
4. Prospects
Both Auger and TA have now presented a large set of results out of which only a few selected topics have been presented. Together with many answers, several questions and puzzles arised. The main challenges concerning ultra-high energy cosmic ray detection go in two different directions. One of them is statistics, and thus detection area. This critical issue concerns all aspects of the analysis, from the study of the spectrum of sources to the sensitivity to exotic primaries. On the other side, there is the need to improve the capability to measure air showers in more detail, measuring and combining different observables. A particularly important aspect is the separation of the electromagnetic and muonic shower components, crucial for composition studies.
Auger and TA will continue to take data, and both collaborations are currently pushing forward their upgrade plans. In Auger, proposed upgrades currently go mainly in the direction of a more detailed shower measurement, and in particular of the muon/electromagnetic shower separation, with intense R&D going on in the last few years. TA, on the other hand, envisages the extension of the SD array sensitivity both to lower and to higher energies, covering an area that can go up to four times the present TA. The years to come will thus provide a large quantity of valuable data which will be crucial to deepen our understanding of extreme energy cosmic rays.
Acknowledgements
This work was supported by FEDER/COMPETE-QREN and FCT, Portugal.
Additional information
Funding
Notes on contributors
Catarina Espirito Santo
Catarina Espirito Santo is a member of the Auger collaboration and of the Auger-LIP group, the Portuguese team participating in Auger. The Auger-LIP group actively participates in the analysis of the Auger data and is particularlyinterested in composition studies, hadronic interactions and other aspects of ultra-high energy cosmic ray physics that open windows to particle physics well above accelerator energies.
The team is deeply involved in the Augerupgrade plans and particularly interested in the measurement of the muon component as a handle to a deeper understanding of air showers. The author is also committed to exploring the large communication and outreach potential of cosmic rays as a window both to the infinitely large and to the infinitely small.
Notes
This article is associated with the From Higgs to Dark Matter 2014 meeting in Geilo, Norway.
References
- Aab, A., et al., The Pierre Auger Collaboration. (2014). Depth of maximum of air-shower profiles at the Pierre Auger Observatory. I. Measurements at energies above 1017.8 eV. Physical Review D, 90, 122005.
- Aab, A., et al., The Pierre Auger Collaboration. (2015). Searches for anisotropies in the arrival directions of the highest energy cosmic rays detected by the Pierre Auger Observatory. The Astrophysical Journal, 804, 15–32.
- Aab, A., et al., The Pierre Auger Collaboration. (XXXX). The Pierre Auger Cosmic Ray Observatory (submitted to Nuclear Instruments and Methods A). arXiv: 1502.01323.
- Abbasi, R., Bellido, J., Belz, J., et al., for the Telescope Array and Pierre Auger Collaborations. (2014). Report of the working group on the composition of ultra high energy cosmic rays. In Proceedings of UHECR 2014 Symposium. Springdale, UT.
- Abbasi, R. U., et al., The Telescope Array Collaboration. (2014). Indications of intermediate-scale anisotropy of cosmic rays with energy greater than 57 EeV in the northern sky measured with the surface detector of the telescope array experiment. The Astrophysical Journal Letters, 790, L21.
- Abraham, J., et al., The Pierre Auger Collaboration. (2009). Upper limit on the cosmic-ray photon fraction at EeV energies from the Pierre Auger Observatory. Astroparticle Physics, 31, 399–406.
- Dawson, B. R., et al., for the Pierre Auger, Telescope Array and Yakutsk Collaborations. (2013). The energy spectrum of cosmic rays at the highest energies. EPJ Web of Conferences, 53, 01005.
- de Souza, V., for the Pierre Auger Collaboration. (2013). An update on the measurements of the depth of shower maximum made at the Pierre Auger Observatory. In Proceedings of the 33rd International Cosmic Ray Conference (p. 35). Rio de Janeiro, arXiv: 1307.5059.
- Farrar, G., for the Pierre Auger Collaboration. (2013). The muon content of hybrid events recorded at the Pierre Auger Observatory. In Proceedings of the 33rd International Cosmic Ray Conference (p. 52). Rio de Janeiro, arXiv: 1307.5059.
- Garcia-Gamez, D., for the Pierre Auger Collaboration. (2013). Observations of the longitudinal development of extensive air showers with the surface detectors of the Pierre Auger Observatory. In Proceedings of the 33rd International Cosmic Ray Conference (p. 40). Rio de Janeiro, arXiv: 1310.0647.
- Hanlon, W., for the Telescope Array and Pierre Auger Collaborations. (2013). Progress towards understanding the analyses of mass composition made by the Auger and Telescope Array Collaborations. In Proceedings of the 33rd International Cosmic Ray Conference (p. 15). Rio de Janeiro, arXiv: 1310.0647.
- Kegl, B., for the Pierre Auger Collaboration. (2013). Measurement of the muon signal using the temporal and spectral structure of the signals in surface detectors of the Pierre Auger Observatory. In Proceedings of the 33rd International Cosmic Ray Conference (p. 48). Rio de Janeiro, arXiv:1310.0647.
- Kuempel, D., for the Pierre Auger Collaboration. (2013). Directional search for ultra-high energy photons with the Pierre Auger Observatory. In Proceedings of the 33rd International Cosmic Ray Conference (p. 72). Rio de Janeiro, arXiv:1307.5059.
- Matthews, J., for the Pierre Auger and Telescope Array Collaborations. (2013). Progress towards a cross-calibration of the Auger and telescope array fluorescence telescopes via an air-borne light source. In Proceedings of the 33rd International Cosmic Ray Conference (p. 7). Rio de Janeiro, arXiv: 1310.0647.
- Schulz, A., for the Pierre Auger Collaboration. (2013). The measurement of the energy spectrum of cosmic rays above 3 x 1017 eV with the Pierre Auger Observatory. In Proceedings of the 33rd International Cosmic Ray Conference (p. 27). Rio de Janeiro, arXiv: 1307.5059.
- Selvon, A. L. , for the Pierre Auger Collaboration. (2014). Highlights from the Pierre Auger Observatory. Brazilian Journal of Physics, 44, 560–570, arXiv:1310.4620.
- Tokuno, H., The Telescope Array Collaboration. (2012). New air fluorescence detectors employed in the telescope array experiment. NIM A, 676, 54–65.
- Tinyakov, P., for the Telescope Array Collaboration. (2014). Latest results from the telescope array. 4th Roma International Conference on Astroparticle Physics ( NIM A), 742, 29–34
- Valiño, I., for the Pierre Auger Collaboration. (2013). A measurement of the muon number in showers using inclined events recorded at the Pierre Auger Observatory. In Proceedings of the 33rd International Cosmic Ray Conference (p. 44). Rio de Janeiro, arXiv: 1310.0647.