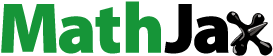
Abstract
When an object rolls on the surface of the mountain, the structure of surface may be destroyed subsequently with a regeneration region. Corresponding experimental simulation presents that the regenerating region exists three regions: compression, thin, and accumulation regions; the shape of regeneration region, as a quasi-parabola, is related to the size and initial velocity of the sphere as well as the slope of surface. Our study suggests that the length maximum of regenerating region is not associated with the initial velocity of sphere; it is found that the length of thin region increases with both the sphere size and the slope of surface.
Public Interest Statement
The granular matters ranging from 1 μm to 104 m, which is the intermediate state of condensed matter with certain behaviors of solid and fluid, but cannot be described by the traditional solid and fluid theory. On the one hand, researches with particle provide possibility to study the theory with non-linear and complex systems through experiment; on the other hand, the classic model is also helpful for engineering design in the engineering field. Many matter movements are related to the granular matter, such as earthquakes, avalanches, debris flows, and landslides. In the present paper, region-regenerating shape of the granular medium surface has been discussed in detail, which plays an important role in understanding the physical mechanism of above the matter movement.
1. Introduction
Granular matter is fascinating because of its solid-like and fluid-like properties. Many matter movements are related to the granular matter, such as earthquakes, avalanches, debris flows, and landslides. Understanding of its physical mechanism will help us to take effective measures to reduce the losses. To disclose these natural phenomena, there have been numerous studies on an object how to interfere with the granular medium, such as rolling a sphere on the surface of granular medium (Aguirre, Ippolito, Calvo, Henrique, & Bideau, Citation1997; Batista-Leyva, Pacheco-Vázquez, & Ruiz-Suárez, Citation2010; De Blasio & Saeter, Citation2009a, Citation2009b; Fukumoto, Sakaguchi, & Murakami, Citation2013; Henrique, Aguirre, Calvo, Ippolito, & Bideau, Citation1997; Henrique et al., Citation1998; Samson, Ippolito, Bideau, & Batrouni, Citation1999; Vasconcelos & Veerman, Citation1999; Yan, Shi, Huang, & Sun, Citation2007) or through the granular medium (Pacheco-Vázquez & Ruiz-Suárez, Citation2009), and making a sphere impact with the surface (Deboeuf, Gondret, & Rabaud, Citation2009; Heil, Rericha, Goldman, & Swinney, Citation2004; Hou, Peng, Liu, & Lu, Citation2005; Kolb, Goldenberg, Inagaki, & Clément, Citation2006; Long et al., Citation2014; Lumay & Vandewalle, Citation2010; Varas, Vidal, & Géminard, Citation2009). Among it, Vasconcelos and Veerman (Citation1999) and Yan et al. (Citation2007) mainly studied a small sphere moving on the fixed monolayer granular medium. However, they did not consider the influence of friction between granules. Batista-Leyva et al. (Citation2010) investigated a small disk-shaped intruder sliding on the unfixed monolayer granular medium; they found a frictional force with a dependence on the speed of the intruder. Fukumoto et al. (Citation2013) investigated the effects of rolling friction of the particles on the packing of granular assemblies. De Blasio and Saeter (Citation2009a, 2009b) observed the microscopic motion of both the sphere and the surrounding granular medium by the high-speed camera, together with the relationship between the move distance and the density of sphere. Lumay and Vandewalle (Citation2010) studied the rearrangement due to a small local cyclic perturbation applied to a packing of rigid grains, which were characterized by particle displacement, the packing fraction, and the coordination number. Makse (Citation1999) studied the segregation of granular mixtures in the continuous avalanche regime in the rotating drums; they found the segregation depends on the species size and surface properties. They (Makse, Citation2000) also found that the grain segregation exists three mechanisms in Aeolian sand ripples.
However, information on the region-regenerating surface shape being affected is still scarce. For this reason, we mainly investigated the relationship between the shape of regeneration region and some quantities, i.e. the size and the initial velocity of sphere, together with the slope of granular medium surface.
2. Experimental setup and method
The setup (Figure ) mainly consists of two flumes, one is granular medium flume filled with the granule of 0.05 mm, the scale of which is 70.00 cm × 18.70 cm × 14.00 cm. The other is accelerating groove made of rigid aluminum, the surface of which has a V-shaped slot; the length of groove is 60.00 cm. According to Fabio’s experimental results (De Blasio & Saeter, Citation2009a), when the filled depth of the flume is 14.00 cm, the results are independent of the depth. In our experiment, when the width of the flume is 18.70 cm, we have found that the width is not related to the motion of sphere. To observe the clear regenerating region shape, a thin layer of black granules was covered on the surface of the granular medium. The sphere starts and accelerates from the static states at L0 from the top. While the sphere rolling, the surface structure of granular medium will be destroyed and restructured and the corresponding regenerating region shape was recorded by camera. Then, we used the photo processing technology to make the shape more clear, and GETDATA software to read out the coordinates of each point at the regenerating region boundary. The origin of coordinates is positioned at the center of the sphere. The experiment was repeated five times at the same condition.
3. Results and discussion
3.1. Description for characteristic of region-regenerating shape
On the experiment photo (Figure ), we choose two perpendicular lines as x and y coordinate axis along the direction of the width (r) and the length (L) of the regenerating region, respectively. When the sphere moves on the granular medium surface along the L direction, it will consecutively collide with the granular medium. When they stopped, the regeneration region was formed in front of sphere. We found this region exists three regions, namely compression region, thin, and accumulation regions. Among it, the compression ridge was created by the sphere embedding into granular medium, and this region had much energy dissipation; the thin region was mainly from granule accumulation from the compression ridge; the accumulation region was created by the high-velocity granular. The thickness of regeneration region is not constant and that of the middle part is the thinnest along the L direction. Its shape is a quasi-parabola and symmetrical (Figure ), and related to the size of sphere and the slope of granular medium surface. During experiment, we also found that the regeneration region will not stable due to the larger slope and the granular medium being easier to slide, if the slope of granular medium surface is larger than 25°.
Figure 2. Experimental photo of regeneration region with three regions: compression region (C-region), thin region (T-region), and accumulation region (A-region).
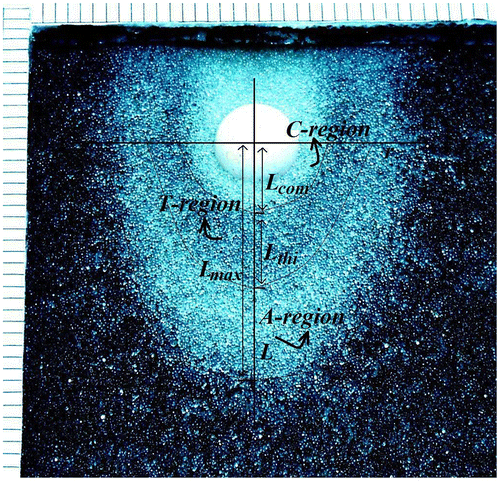
Figure 3. Region-regenerating shape on the granular medium surface is mainly created by collision with different sizes of the sphere, the velocity of which is 98.8 cm/s. And the slope of granular medium surface is 19°.
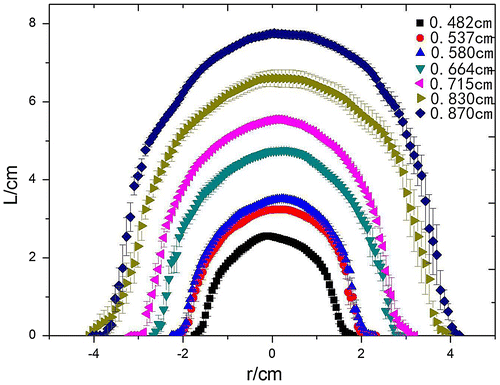
Some parameters to describe the shape, i.e. area (S), length maximum (Lmax), width maximum (Wmax), as a function of initial velocity of sphere V (a–c), radius R (d–f), and slope θ (g–i), respectively (Figure ). From Figure (a), the S firstly increases and then decreases with the initial velocity V, which results from change in the width rather than the length (due to its little change with the velocity (Figure (b))). Thus, change in the Wmax is the same to the S (Figure (c)). When the sphere moves at the lower velocity, the sinking depth of the sphere increases with the initial velocity V; once the velocity exceeds a critical value, the depth decreases with it (De Blasio & Saeter, Citation2009a). So the change in the width of the regeneration region is not monotonic with the velocity of the sphere. The change in the length Lmax implies that the energy of granular is independent of the sphere velocity V (Figure (b)), which indicates that the granular is mainly affected by the sphere radius and slope of surface. Note that the S square-like increases with the sphere radius R, and meanwhile both Lmax and Wmax also linear-like increase with the R (Figure (e) and (f)). It proves that the sphere size plays an important role in forming the regeneration region. As the radius of sphere increases, the range of the collision between the sphere and the granular medium is expanded, and the sinking depth of the sphere is also increased. Therefore, the total area is increased. The S non-linearly increases with the θ, meanwhile the Lmax changes in similar way with the S, but the Wmax firstly increases to the maximum and eventually tends to a constant (Figure (g–i)). With the increase in the slope (θ) surface, the granular medium is easier to roll, and consequently the influenced region is expanded, thus both the length and width also increased.
Figure 4. Series changes in shape parameters for the regeneration region: changes in area (a, d, g), length (b, e, h), and width (c, f, i) of regeneration region with initial velocities, radius of sphere, slope of surface (for (a–c), radius of 0.715 cm, and flume slope of θ = 19.0°; for (d–f), velocity of 98.8 cm/s and θ = 19.0°; for (e), blank circles denote the theoretical values based on Equation 8; for (g–i), sphere velocity and radius of 98.8 cm/s and 0.715 cm, respectively, and the dashed line denotes the theoretical values based on Equation 8.
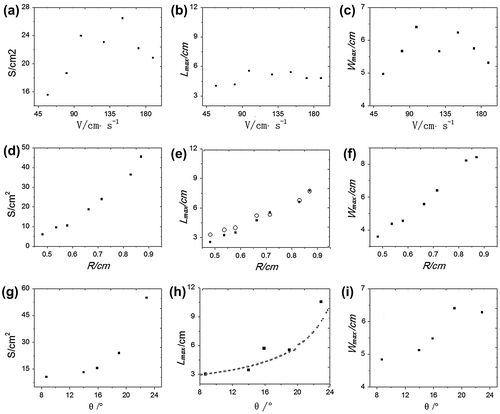
3.2. Theoretical analysis for the formation of regeneration region
To study the physical mechanism of the formation, we used the length derivative (dL/dr) with respect to the width r for shape curves (Figure ). It indicates that dL/dr increases firstly slowly, and then shapely when the width is larger than 2.0 cm. Furthermore, the differential curves are independent of the velocity of sphere and the angle of granular medium surface. Therefore, the regeneration region is mainly from the collision between the sphere and granule medium.
Figure 5. The differential curves of regeneration region shape, (a) the curves for different sizes of the sphere, where θ = 19° and v0 = 98.8 cm/s; (b) experiment curves for R = 0.715 cm, theory curves were calculated by Equations 6 and 7.
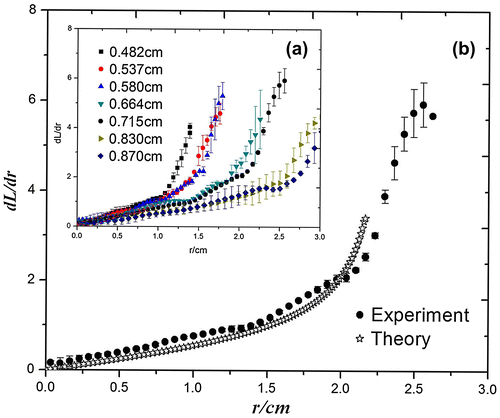
In the sketches of collision between the sphere and a granule (Figure ), v0 is the velocity of sphere before collision. The positive direction of the Y axis is along the movement direction of the sphere, α is the angle between the directions of velocity of both the sphere and the collided granule. Then, we put the velocity decomposed in the directions along the sphere-granular line, the component velocity is
(1)
(1)
Figure 6. Sketches of collision, (a) a top view, (b) a side view; black ball is a granule, and h is the sinking depth in the surface of granular medium.
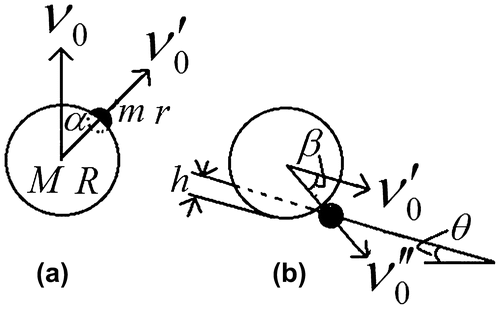
According to the conservation of momentum, there is(2)
(2)
where m (m = 1.31 × 10−3 g) is the mass of a single granular, M is the mass of sphere, vg is the single granular velocity after collision, and e is the coefficient of restitution (0 < e < 1). The sphere velocity after collision is ev0. From Equation 2, there is(3)
(3)
When the granule moves, we mainly consider the friction between granules. So the movement distance of the single granular along the granular medium surface is(4)
(4)
where μg is the friction coefficient between granules, h is the sinking depth of the sphere, R is the radius of sphere, and θ is the slope of granular medium surface. Because of the continuous motion of the sphere after the collision, the movement distance of the sphere is(5)
(5)
where μs is the friction coefficient between sphere and granular, μg and μs are the effective friction coefficient. Furthermore, there are(6)
(6)
(7)
(7)
When α = 0, the distance is the length maximum of the regeneration region, namely(8)
(8)
where the density of sphere is ρ = 3.23 g/cm3. By Equations 6 and 7, the dL/dr values and the curves of R = 0.715 cm are shown in Figure (b). When r is small, this result agrees to the experiment. But when r increases, this result has a slight difference with experiment, because the inter-granule viscidity cannot be ignored. In addition, the sinking depth of sphere increases with its radius R when they move on the granular medium surface. This result agrees to that of Hou et al. (Citation2005). According to Equation 8, Lmax increases with θ, R, and v0, respectively, but Lmax is independent of the initial velocity V from the experimental results. So the formation of regeneration region is only related to v0 and not related to the initial velocity of the sphere.
3.3. Thin region
For the thin region (Figure ), Lthi and Lcom are the lengths of thin region and compression region along the movement direction of the sphere, respectively. The thin region is axis-symmetry with trajectory of the sphere like horseshoe shape. It is mainly from the accumulation of the lower velocity granules, which may result from the collision between the granular and sphere as well as the granules of the compression region.
Figure (a) shows Lthi, Lcom, and Lacc increase with radius of the sphere with the same growth rate. Moreover, Lcom is larger than Lthi at the same R, indicating the close relationship between the length of the compression region and the radius of sphere. As shown in Figure (b), Lcom non-linearly increases with θ, first slowly and then dramatically, implying that the structure of the granular medium at a large slope is looser than that at the smaller slope. Meanwhile, the Lthi has the same change trend with the larger growth rate, but the growth rate of Lacc is larger than the one of Lthi. Figure (c) shows that the relationships between the sphere velocity and the Lthi, Lacc, and Lcom. The Lcom is not related to the velocity of sphere. Therefore, the velocity of the sphere has no effect on the length of compression region. And the Lthi slowly increases with the velocity of sphere with the value of Lthi smaller or larger than that of Lcom at the lower or higher velocity of sphere, respectively. The Lacc has a non-monotonic relationship with velocity with the first increase and then decreases.
Figure 7. Changes in the length of compression, accumulation, and thin regions with radius of the sphere (a), and the slope of granular medium surface (b) as well as velocity of the sphere (c), respectively; circle red points for the length of the compression region, and square black points for that of thin region, blue triangle for that of accumulation region.
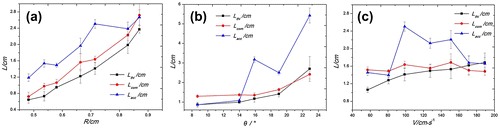
4. Conclusion
The region-regenerating shape, being mainly from the collision between the sphere and granular medium, is a quasi-parabola, and related to the following parameters, i.e. radius and velocity of the sphere, and slope of the granular medium surface. This region contains three parts, namely compression, thin, and accumulation regions. The lengths of both regeneration region and compression region along the movement direction of the sphere do not change with velocity of the sphere, and the length of region increases with the velocity of the sphere, but the length of accumulation region decreases with the velocity.
Additional information
Funding
Notes on contributors
Yousheng Yu
Yu Yousheng is a lecturer at School of Physics & Electronic Engineering, Kaili University, Guizhou, China. He received his MS from Guizhou University. His research interests include soft matter physics, nanoparticle, and molecule transport.
Lin Hu
Hu Lin is a professor at College of Science, Guizhou University. She has 15 years of postgraduate teaching experience. She has published more than 80 research papers in international journals. Her area of interests includes granular matter, biological physics, and optical .
References
- Aguirre, M. A., Ippolito, I., Calvo, A., Henrique, C., & Bideau, D. (1997). Effects of geometry on the characteristics of the motion of a particle rolling down a rough surface. Powder Technology, 92, 75–80.10.1016/S0032-5910(97)03231-2
- Batista-Leyva, A. J., Pacheco-Vázquez, F., & Ruiz-Suárez, J. C. (2010). Role of density in granular lubrication. Physical Review E, 82, 031304.10.1103/PhysRevE.82.031304
- De Blasio, F. V., & Saeter, M. B. (2009a). Rolling friction on a granular medium. Physical Review E, 79, 022301.10.1103/PhysRevE.79.022301
- De Blasio, F. V., & Saeter, M. B. (2009b). Small-scale experimental simulation of talus evolution. Earth Surface Processes and Landforms, 34, 1685–1692.10.1002/esp.v34:12
- Deboeuf, S., Gondret, P., & Rabaud, M. (2009). Dynamics of grain ejection by sphere impact on a granular bed. Physical Review E, 79, 041306.10.1103/PhysRevE.79.041306
- Fukumoto, Y., Sakaguchi, H., & Murakami, A. (2013). The role of rolling friction in granular packing. Granular Matter, 15, 0398.
- Heil, P., Rericha, E. C., Goldman, D. I., & Swinney, H. L. (2004). Mach cone in a shallow granular fluid. Physical Review E, 70, 060301(R).10.1103/PhysRevE.70.060301
- Henrique, C., Aguirre, M. A., Calvo, A., Ippolito, I., & Bideau, D. (1997). Experimental acoustic technique in granular flows. Powder Technology, 94, 85–89.10.1016/S0032-5910(97)03286-5
- Henrique, C., Aguirre, M. A., Calvo, A., Ippolito, I., Dippel, S., Batrouni, C. G., & Bideau, D. (1998). Energy dissipation and trapping of particles moving on a rough surface. Physical Review E, 57, 4743–4750.10.1103/PhysRevE.57.4743
- Hou, M., Peng, Z., Liu, R., & Lu, K. (2005). Dynamics of a projectile penetrating in granular systems. Physical Review E, 72, 062301.10.1103/PhysRevE.72.062301
- Kolb, E., Goldenberg, C., Inagaki, S., & Clément, E. (2006). Reorganization of a two-dimensional disordered granular medium due to a small local cyclic perturbation. Journal of Statistical Mechanics Theory and Experiment, 7, 07017.
- Long, E. J., Hargrave, G. K., Cooper, J. R., Kitchener, B. G. B., Parsons, A. J., Hewett, C. J. M., & Wainwright, J. (2014). Experimental investigation into the impact of a liquid droplet onto a granular bed using three-dimensional, time-resolved, particle tracking. Physical Review E, 89, 032201.10.1103/PhysRevE.89.032201
- Lumay, G., & Vandewalle, N. (2010). Flow of magnetized grains in a rotating drum. Physical Review E, 82, 040301(R).10.1103/PhysRevE.82.040301
- Makse, H. A. (1999). Continuous avalanche segregation of granular mixtures in thin rotating drums. Physical Review Letters, 83, 3186–3189.10.1103/PhysRevLett.83.3186
- Makse, H. A. (2000). Grain segregation mechanism in Aeolian sand ripples. The European Physical Journal E, 1, 127–135.10.1007/pl00014592
- Pacheco-Vázquez, F., & Ruiz-Suárez, J. C. (2009). Sliding through a superlight granular medium. Physical Review E, 80, 060301.10.1103/PhysRevE.80.060301
- Samson, L., Ippolito, I., Bideau, D., & Batrouni, G. G. (1999). Motion of grains down a bumpy surface. Chaos: An Interdisciplinary Journal of Nonlinear Science, 9, 639–648.10.1063/1.166437
- Varas, G., Vidal, V., & Géminard, J. C. (2009). Dynamics of crater formations in immersed granular materials. Physical Review E, 79, 021301.10.1103/PhysRevE.79.021301
- Vasconcelos, G. L., & Veerman, J. J. P. (1999). Geometrical model for a particle on a rough inclined surface. Physical Review E, 59, 5641–5646.10.1103/PhysRevE.59.5641
- Yan, H., Shi, Q. F., Huang, D. C., & Sun, G. (2007). Dynamics of a viscous ball rolling down on a rigid staircase. Physica A: Statistical Mechanics and its Applications, 374, 524–532.10.1016/j.physa.2006.07.037