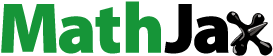
Abstract
The ultrasonic velocity (U), density (ρ), and viscosity (η) of an ethanolic extract of drug Piper nigrum with MgCl2 (metal ions) have been measured as a function of the number of moles n = (0.7009, 1.4018, 2.1027, 2.8036 and 3.5045) at 303.15, 308.15, 313.15 and 318.15 K temperature. Various thermoacoustic and their excess values such as adiabatic compressibilities (β), intermolecular free lengths (Lf), excess adiabatic compressibility (βE), excess intermolecular free length () have been computed using values of ultrasonic velocity (U), density (ρ), and viscosity (η). The excess values of ultrasonic velocity, specific acoustic impedance are positive, whereas isentropic compressibility and intermolecular free lengths are negative over the entire composition range of MgCl2 + P. nigrum which indicates the presence of specific interactions between unlike molecules. Molecular association is reflected by ultrasonic investigation. This may be interpreted due to the of complex formation. The chemical interaction may involve the association due to the solute–solvent and ion–solvent interaction and due to the formation of charge-transfer complexes, which is useful to understand the mechanism of their metabolism in living systems. The results obtained from these studies are helpful for pharmacological applications of drugs, transport of drugs across biological membranes.
Public Interest Statement
Synthesis and ultrasonic investigation of herbal drug is an emerging field for the researchers working in the area of pharmaceuticals. The paper deals with the hot extraction synthesis of drug Piper nigrum which belongs to Piperaceae family. Ultrasonic and thermoacoustic parameters are used to investigate the intermolecular interaction between drug and metal ions. Mg++ ions play an important role in respiratory system. Therefore, Mg++ ions are selected to study the interaction with P. nigrum as an antioxidant. The influences of drug concentration and temperature have been systematically investigated. The liquid mixture of P. nigrum and metal ion have been investigated using experimental parameters such as (ultrasonic velocity (U), density (ρ), and viscosity (η)), various thermoacoustic parameters such as adiabatic compressibility (β), intermolecular free length (Lf), and specific acoustic impedance (Z) measurements. The results obtained from these studies are helpful for pharmacological drugs applications, transport of drugs across biological membranes.
1. Introduction
Though spectroscopic method (Min et al., Citation2004; Rahman, Maruyama, Okada, Yamasaki, & Otagiri, Citation1993) plays a major role in the molecular interaction studies, the non-spectral studies such as calorimetric (Bruylants, Wouters, & Michaux, Citation2005; Haq, Citation2002), magnetic (Gupta et al., Citation2011; Karplus & McCammon, Citation2002), ultrasonic velocity and viscosity measurements (Pradhan, Dash, Moharana, & Swain, Citation2012; Priya, Nithya, Velraj, & Kanappan, Citation2010) have been widely used, in the elucidation of the formation of complexes. Ultrasound is regarded as being of low intensity when there is no permanent changes takes place in the material during propagation of ultrasonic waves (Blitz & Simpson, Citation1995). The study of molecular interactions can be carried out by diffraction methods such as dielectrics (Hill, Citation1969; Pethig, Citation1992), Raman effect (Logan, Citation1989; Tanabe & Hiraishi, Citation1982), IR (Awasthi & Shukla, Citation2003; Wang, Wu, Xuan, & Wang, Citation2002) and ultrasonic (Ali, Hyder, & Nain, Citation1999; Fort & Moore, Citation1965; Kannappan, Thirumaran, & Palani, Citation2009), etc. Ultrasonic technique employed to investigate the properties of any substance to understand the nature of molecular interactions in pure liquid (Jacobson, Citation1952), liquid mixtures (Bradley, Ashokkumar, & Grieser, Citation2013), solutions (Sarvazyan, Citation1982), aqueous (Nikam, Hasan, Pawar, & Sawant, Citation2004; Pavai, Vasantharani, & Kannappan, Citation2004), non aqueous, (Kannappan & Vinayagam, Citation2007; Keller, Legendziewicz, Gliński, & Samela, Citation2000) mixed electrolytic solutions, (Darbari, Richelson, & Petrucci, Citation1970; Zafarani-Moattar & Shekaari, Citation2005) etc. has led to new insights into the process of ion–ion and ion–solvent interaction. These measurements are used to estimate the different elastic properties of the molecules from which the type of molecular interactions can be very well understood. In the recent years, many attempts have been made in the field of physical acoustics and understood in solids, liquids, and gases. The ion dipole interaction mainly depends on ion size and polarity of solvent. Study of ion dipole attraction is directly proportional to the size of the ions, magnitude of the dipole, but inversely proportional to the distance between ion and molecules (Sonar & Pawar, Citation2010). When two or more liquids are mixed, there occur some changes in physical and thermodynamic properties because of free volume changes, change in energy, and change in molecular orientations. The practical application of mixed solvent rather than single solvent in industrial and biological process has been recognized all over the world as they provide a wide choice of solutions with appropriate properties (Thirumaran & Priya, Citation2013). Ultrasonic measurements of acoustic parameters to change in number of moles give an insight into the molecular process (Bhandakkar, Bhat, Chimankar, & Asole, Citation2014). There are two types of molecular interactions in liquids, namely long range and short range. Long range arises without overlap of electron clouds and is highly directional, whereas short range arises due to overlapping of electron clouds. In Nomoto’s theory, it is supposed that the volume does not change on mixing. Therefore, no interaction between the components of liquid mixtures has been taken into account. The assumption for the formation of ideal mixing relation is that the ratio of specific heats of an ideal mixture and the volumes is also equal. Again, no molecular interaction is taken into account. But on mixing two liquids, the interactions between the molecules of the two liquids takes place because of the presence of various types of forces such as dispersion forces, charge transfer, hydrogen bonding, dipole–dipole and dipole-induced dipole interactions (Babavali, Shakira, Srinivasu, & Narendra, Citation2015). The sign of the deviation of an excess property may be negative or positive from the ideal value depending on the type and extent of interactions between unlike molecules. Activeness of a drug is often catalyzed by the presence of metal ions (Umaley, Pethe, & Aswar, Citation2013). Investigation of such drugs, the interaction of their chemical groups with metal ions and their interactions in solution play an important role in understanding the biochemical processes occurring in living systems (Malasane & Aswar, Citation2005). All pharmokinetics processes involve transport of drugs across biology membrane which are well understood by transport property measurement such as ultrasonic velocity, density, and viscosity (Bronzino, Citation1999). From these parameters intermolecular interactions in aqueous solutions of drug can be directly predicted. It gives an idea about activeness of the drug. Drugs compress the solvent, but it shows different solute solvent interactions due to their different sizes, shape, and structure. Alternative to traditional therapy, since low dosage is required with lesser side effects (Avery et al., Citation2006). A knowledge of the use of drugs involving physiological and biochemical effects, and their mechanism of action at macromolecular /sub cellular/organ system levels can be studied in pharmokinetics (Syal, Thakur, Chauhan, & Sharma, Citation2005).
Metal ions are recognized as essential micronutrients, which provide structure and activity basis to several bimolecular systems. Many researchers have studied acoustical properties of solutions containing transition metal ions (Das & Jha, Citation1992; Ravichandran & Ramanathan, Citation2010). Ultrasonic velocity and viscosity studies have been made for various salts (Roy, Jha, & Choudhury, Citation2004; SingháGill, Citation1995; Victor, Das, & Hazra, Citation2001) but these studies are rare for drugs. The significance of metal ions in biological reactions has gained much importance during the last two decades. The study of molecular association in liquid mixture having alcohol as one of the components is of particular interest since alcohols are strongly self-associated liquids having three-dimensional network of hydrogen bonding and can be associated with any other group having some degree of polar attraction (Ramteke, Citation2012). The hydroxyl group makes the alcohol molecule polar. Those groups can form hydrogen bonds to one another and to other compounds. This hydrogen bonding means that alcohols can be used as protic solvents. Two opposing solubility trends in alcohols are: the tendency of the polar OH to prepare solubility in water, and the tendency of the carbon chain to resist it. Ethanol can dissolve a large number of chemical substances such as alkaloids, alkaloidal salts, and tannins. Piper nigrum is pungent alkaloid, insoluble in water, and soluble in ethanol (Bhaskar & Patel, Citation2014; Bhatia, Bhatia, & Dubey, Citation2010) hence ethanol is used as a solvent for the extraction of P. nigrum. After review of literature survey the detail study of drugs under an identical set of experimental condition is still lacking. P. nigrum has been used extensively in Ayurveda medicine for centuries, as it is nontoxic (Uddin, Samiulla, Singh, & Jamil, Citation2012) and has a variety of therapeutic properties including antioxidant (Meghwal & Goswami, Citation2013), inflammatory (Mujumdar, Dhuley, Deshmukh, Raman, & Naik, Citation1990), antibacterial (Venkat Reddy et al., Citation2004), properties etc. P. nigrum is used for respiratory system (Jamwal & Singh, Citation1993; Trivedi & Mishra, Citation2010). Molecular interaction studies of the metal ions like Na++, K+, Ca++, Zn++, Cu++, and Fe++ with antioxidant drugs (curcumin, Piper longum) and their derivatives will be useful in understanding the mechanism of action of antioxidants in the living system. The medicinal plants are an important source of minerals, vitamins, and trace element and are regarded as a potentially safe drug. Identification of interaction between metal ions with drug become more interesting due to the complex structure of drug and their activities involves interaction with biological membranes. To interpret the physicochemistry of biological systems, it is essential to examine the properties of drug with metal ions. Therefore, we have undertaken a systematic study on the ultrasonic investigation of an antioxidant ethanolic extract of P. nigrum with magnesium chloride to investigate interactions present in that system. Various thermoacoustic and their excess values such as adiabatic compressibilities (β), intermolecular free lengths (Lf), excess adiabatic compressibility (βE), excess intermolecular free length () have been computed using values of ultrasonic velocity (U), density (ρ), and viscosity (η) to determine presence of intermolecular interactions occurring in the mixtures.
A vital role is played by drug–metal ion interactions in all the metabolic pathways or biological processes occurring inside the body. Studies of such interactions led to modern drug discovery. Recently, we have reported a detailed investigation on ultrasonic studies of ZnCl2 with the drug P. nigrum system. The conclusion drawn from such investigation is that the mixture acts as structure breaker and there is a complex formation between ZnCl2 and P. nigrum for the system as a function of concentration and temperature (Nalle, Birajdar, Shinde, Dorik, & Jadhav, Citation2016).
Mg++ ions play a major role in the respiratory system and P. nigrum has medicinal properties against disease occurring in the respiratory system (Ram, Joseph, Balachandar, & Singh, Citation2009). Therefore, Mg++ ions are selected to study the interaction with P. nigrum as an antioxidant.
2. Materials and methods
2.1. Preparation of extract from P. nigrum seeds
The pure dried seeds of P. nigrum of good quality were purchased from the local market and used as such. Seeds were ground to a coarse powder. Fifty grams of powdered seed were boiled with half liter ethanol in a conical flask for 30 min, cooled at room temperature and then it is decanted. The solutions were prepared by adding a known molecular weight of magnesium chloride in ethanol for the preparation of one molar solution of magnesium chloride in ethanol using a magnetic stirrer until a clear solution was obtained. Then known number of moles of drug (P. nigrum) was added into a fixed volume of solvent.
Proper calibration of each temperature was achieved with double-distilled water as standard. After mixing the sample, the bubble-free homogeneous sample was transferred into the measuring cell of the interferometer which is specially designed double-walled vessel with provision to circulate water at required temperature. The velocity of ultrasonic waves in the pure liquids and liquid mixtures was determined using a single frequency interferometer (Model F-81) with a high degree of accuracy operating at frequency 2 MHz supplied by Mittle enterprises, New Delhi. The high-frequency generator excites a quartz crystal fixed at the bottom of the vessel, at its resonant frequency. A fine micrometer screw at the top of the cell is used to raise or lower the reflector plate in the liquid through a known distance. The measuring cell is connected to the output terminals of the high frequency generator through a cable. Ultrasonic waves normal to quartz crystal are reflected from the reflector plate. Stationary waves are formed in the region between the reflector plate and the quartz crystal. The micrometer is slowly moved till a number of maximum readings (N) of the anode current passed. The total distance (d) moved by the micrometer is noted. The velocity of ultrasonic waves in the pure liquid and liquid mixture was determined using the relation.(1)
(1)
where = wavelength of the ultrasonic waves in the liquid mixture and f is the frequency of the generator (2 MHz).
The density (ρ) of the liquid mixture was determined by a specific gravity bottle of 25 ml capacity. The specific gravity bottle with the liquid mixture was immersed in a temperature controlled water bath. The density was determined using the relation:(2)
(2)
where w1, w2, ρ1, and ρ2 are masses of distilled water, mass of liquid mixture, density of distilled water, and density of liquid mixture, respectively.
The viscosity of (η) of pure liquids and liquid mixture was determined by an Ostwald viscometer containing the liquid was immersed in a temperature controlled water bath and the time flow was measured by a stop watch. The viscosity was determined using the relation:
(3)
(3)
where η1, η2, t1, and t2 are coefficient of viscosity of distilled water, coefficient of viscosity of liquid mixture, time of flow of distilled water and time of flow of liquid mixture, respectively.
3. Theory and calculations
3.1. Physical parameters
Various acoustical and thermo dynamical parameters are calculated from the measured data, such as:
3.1.1. Adiabatic compressibility (β)
The adiabatic compressibility (β) has been calculated from the ultrasonic velocity (U) and density (ρ) of the medium using the equation as:
(4)
(4)
Adiabatic compressibility is a measure of intermolecular association or dissociation or repulsion. The structural arrangement of the molecule affects the adiabatic compressibility.
3.1.2. Specific acoustic impedance (Z)
The specific acoustic impedance is given by following equations, where (U) and (ρ) are the ultrasonic velocity and density of the liquid respectively.
(5)
(5)
Specific acoustic impedance supports the possibility of strong interaction between unlike molecules.
3.1.3. Intermolecular free length (Lf)
(6)
(6)
where K is temperature-dependent constant called as Jacobson constant it is 2.075 × 10−6 for 303.15 K
Intermolecular free length calculates the distance between the surfaces of the neighboring molecules which gives the molecular distance in terms of free length.
3.1.4. Relaxation time (τ)
The relaxation time can be calculated from the relation as:
(7)
(7)
where β and η are adiabatic compressibility and viscosity of the liquid mixture.
Relaxation time is time taken for the excitation energy to appear as translational energy and it depends on temperature and impurities. So this parameter gives the idea about impurities present in liquid mixture.
3.1.5. Relaxation amplitude (α/f2)
The relaxation amplitude can be calculated from the relation as:(8)
(8)
where (η) and (ρ) are viscosity and density of the liquid mixture.
The variation in relaxation amplitude in each curve with concentration strongly supports the presence of strong intermolecular interactions between the constituent molecules of this binary liquid system.
3.2. Excess parameters
The corresponding excess thermo-acoustical parameters such as have been calculated by using the standard equations. The above excess parameters are fitted to the following Redlich–Kister equation.
(9)
(9)
where is
and X represents number of moles of the component and subscript 1 and 2 stands for component 1 and 2.
for parameters of mixture or solution.
4. Results and discussions
The experimental values of ultrasonic velocity (U), density (ρ), viscosity (η) of pure liquids are given in Table (Khan, Citation2009; Naidu & Prasad, Citation2004). Measured and calculated parameters give evidence of structural arrangement.
Table 1. Comparison of experimental ultrasonic velocity (U), density (ρ), and viscosity (η) of pure liquids with literature values at 303.15 K
The experimental data for ultrasonic velocity (U), density (ρ) and viscosity (η) of MgCl2 + P. nigrum system at 303.15, 308.15, 313.15 and 318.15 K are reported in Table and graphically represented in Figure (a)–(c). Various calculated physical and thermodynamical parameters such as adiabatic compressibility (β), intermolecular free length (Lf), specific acoustic impedance (Z), relaxation amplitude (α/f2), and relaxation time (τ) were calculated from the ultrasonic velocity, density, and viscosity and the results are presented in Table and graphically represented in Figure (a)–(e). All these parameters are discussed in terms of solute–solvent interactions occurring in the P. nigrum and MgCl2.
Table 2. Ultrasonic velocities (U), densities (ρ), and viscosities (η) for MgCl2 + P. nigrum system at 303.15, 308.15, 313.15, and 318.15 K
Figure 1. Variation in (a) ultrasonic velocity (U), (b) density (ρ), and (c) viscosity (η) of MgCl2 + P. nigrum (n) system at 303.15, 308.15, 313.15, and 318.15 K.
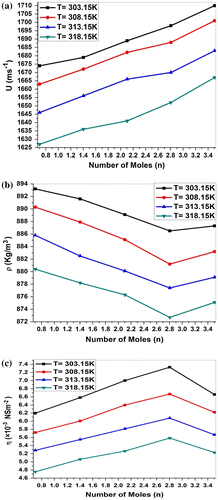
Table 3. Adiabatic compressibility (β) (×10−10 kg−1 ms2), intermolecular free lengths (Lf) (×10−11 m), specific acoustic impedance (Z) (×105 Kgm−2 s−1), relaxation amplitude (α/f2) (×10−14 Npm−1 s2), relaxation time (τ) (×10−12) for MgCl2 + P. nigrum system at 303.15, 308.15, 313.15 and 318.15 K
Figure 2. Variation in (a) adiabatic compressibility (β), (b) intermolecular free length (Lf), (c) specific acoustic impedance (Z), (d) relaxation amplitude (α/f2), and (e) relaxation time (τ) of MgCl2 + P. nigrum (n) system at 303.15, 308.15, 313.15 and 318.15 K.
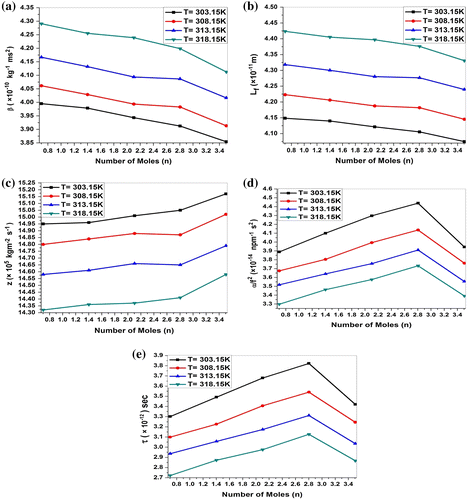
Excess ultrasonic velocity (), density
) and viscosity (
) of MgCl2 + P. nigrum system at 303.15, 308.15, 313.15, and 318.15 K temperature are reported in Table and graphically represented in Figure (a)–(c). Calculated excess physical and thermodynamical parameters such as excess adiabatic compressibility (
), excess intermolecular free length (
), excess specific acoustic impedance (
), excess relaxation amplitude (
), and excess relaxation time (
) are presented in Table and graphically represented in Figures (a)–(e).
Table 4. Excess values of ultrasonic velocity (
), excess density (
) and excess viscosity (
) for MgCl2 + P. nigrum (n) at 303.15, 308.15, 313.15, and 318.15 K
Figure 3. (a) Variation in excess ultrasonic velocity(UE), (b) excess density (ρE), and (c) excess viscosity (ηE) of MgCl2 + P. nigrum (n) system at 303.15, 308.15, 313.15 and 318.15 K.
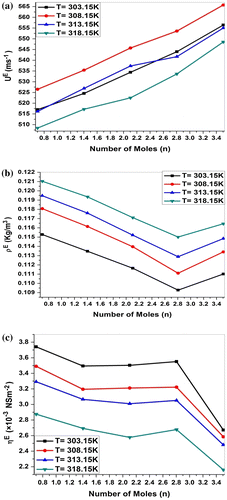
Table 5. Excess values of adiabatic compressibility (
) (×10−10 kg−1 ms2), excess intermolecular free length (
) (×10−11 m), specific acoustic impedance (
) (×105 Kgm−2 s−1), relaxation amplitude (
) (×10−14 Npm−1 s2) and relaxation time (
) (×10−12) for MgCl2 + P. nigrum (n) system at 303.15, 308.15, 313.15 and 318.15 K
Figure 4. (a) Variation in excess adiabatic compressibility (βE), (b) excess intermolecular free length (), (c) excess specific acoustic impedance (ZE), (d) excess relaxation amplitude (
), and (e) excess relaxation time (τE) of MgCl2 + P. nigrum (n) system at 303.15, 308.15, 313.15 and 318.15 K.
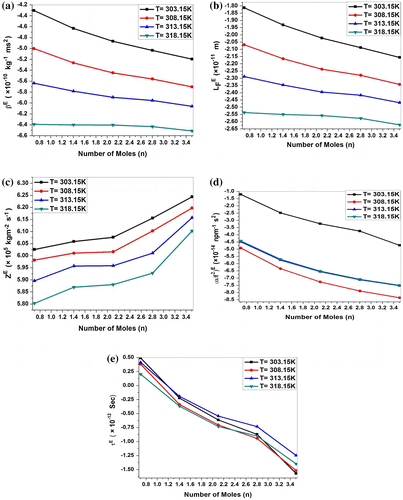
Figure (a) contains the plot of experimental ultrasonic velocity (U) versus number of moles (n) concentration at 303.15, 308.15, 313.15, and 318.15 K temperature. It is observed that ultrasonic velocity (U) increases with an increase in the concentration of P. nigrum in MgCl2 indicating more stability of P. nigrum molecules. The increase in ultrasonic velocity can be attributed to the increase in molecular interaction between P. nigrum and magnesium metal ion. The linear variation in ultrasonic velocity with concentration indicates the occurrence of complex formation between MgCl2 and P. nigrum. Chemical interaction may involve due to the association between the ion and dipole molecule. Increase in relaxation time (Figure (e)) increases the relaxation amplitude (Figure (d)) in this binary liquid system up to concentration from n = 0.7009 to n = 2.8036. At concentration n = 3.5045 relaxation time (τ) and relaxation amplitude (α/f2) both decrease for all temperatures. The variation in relaxation amplitude in each curve with concentration strongly supports the presence of strong ion–dipole intermolecular interactions between the constituent molecules of this binary liquid system. The molecular association becomes maximum which is reflected by an increase in velocity. This may be interpreted due to complex formation producing a displacement of electrons and molecules. The chemical interaction may involve the association due to ion–dipole interaction or due to the formation of charge-transfer complexes. As kinetic energy of the system decreases with increasing temperature, the interactions between solute and solvent become stronger, this causes the speed of sound in the solution to become less pronounced. As the interactions between solute and solvent increases, the sound waves cannot easily pass through the MgCl2 + P. nigrum solutions and hence the speed of sound decreases with increasing temperature (Pawar & Chimankar, Citation2015).
Density of all concentrations of P. nigrum in MgCl2 is listed in Table . It is observed from Table that the density decreases with the increase in concentration of P. nigrum in MgCl2 except at 2.8036 mol concentration. The decrease in density can be attributed to the increase in molecular interaction between P. nigrum and magnesium metal ion. It means molecular interaction increases as the concentration of the drug increases, but at 2.8036 mol concentration, it decreases for all temperatures. Figure (b) shows the variation in density as a function of concentration. It can be seen from Figure (b) that the density decreases with P. nigrum concentration which suggests that the existing intermolecular interaction becomes weak with increasing concentration. Density is a measure of solute–solvent and ion–solvent interactions. Density of various temperatures of P. nigrum with Mg++ is listed in Table and shown graphically in Figure (b). It is observed from Table that density decreases with an increase in temperature of P. nigrum with Mg++ ions. The decrease in density can be attributed to the weakness of intermolecular forces between metal ions and drug molecules.
Viscosity (η) of P. nigrum and magnesium chloride has been determined using Ostwald Viscometer. It is observed from Table that viscosity increases with an increase in concentration of ethanolic extract of P. nigrum in MgCl2. From Figure (c), it is seen that the value of viscosity increases with the increase in concentration of P. nigrum drug except 3.5045 mol concentration. It suggests that the existed intermolecular interaction becomes weak with increasing concentration except at 3.5045 mol value. It is observed from Table and Figure (c) that viscosity decreases with an increase in temperature, which suggests that as the temperature of the solution increases the ordered structure of molecule breaks and therefore more spacing is formed between the molecules. Hence, the intermolecular force decreases due to the increase in thermal energy of the system, which causes an increase in the volume expansion and hence viscosity decreases with temperature. Relation concludes the structure-breaking tendency of metal ions for the cluster of drug molecules.
As expected, the adiabatic compressibility, (β), decreases suggesting interaction between MgCl2 + P. nigrum molecules. Adiabatic compressibility (β) shows an inverse behavior as compared to the ultrasonic velocity. Such trends in the variations of (β) have also been reported by others (Kincaid & Eyring, Citation1938; Nikam & Hiray, Citation1991; Rafiqul Islam & Quadri, Citation1985) during the ultrasonic study of binary mixtures. The added P. nigrum tends, for one thing, to the occasionally break associates present in the MgCl2 molecules with a consequent increase in (β) and (Lf). But, because of the simultaneous formation of strong hydrogen bonding between the unlike molecules there is a compensating effect resulting in an overall decrease in (β) and (Lf), with concentration is observed. The observed trends in the values of (β) and (Lf) with a concentration (Figure (a) and (b)) reinforce the above view. A similar explanation was suggested (Ali, Nain, & Kamil, Citation1996) in order to explain physicochemical studies of nonaqueous binary liquid mixtures of pure N, N dimethylformamide (DMF), ethanol and their binary mixtures at 298.15, 303.15, 308.15, 313.15, and 318.15 K over the whole mole fraction range.
Specific acoustic impedance (Z) increases with the increase in concentration. This supports the possibility of strong interaction between unlike molecules. Acoustic impedance is also given by the product of the ultrasonic velocity and density Z = Uρ and is used for assessing the absorption of sound in a medium.
An ideal solution should be considered as non-associated and for ideal mixture values of excess property is zero. For nonideal mixtures, difference between experimental values and ideal values is significant. The excess parameters are a measure of deviation from ideal behavior and are found to be highly sensitive to intermolecular interactions between component molecules of the mixture. Weak interaction between unlike molecules gives positive excess values, whereas those of dipole–dipole, dipole-induced dipole, charge transfer, and hydrogen bonding between unlike molecules gives negative excess parameters.
The positive values of excess velocity, excess density, and excess viscosity and negative values of excess adiabatic compressibility, excess intermolecular free length and indicate strong interactions between solute and solvent molecules, resulting from disruption of molecular association (Thanuja, Kanakam, & Nithya, Citation2013).
The positive excess values represent the dispersion forces, while the negative values interpret the ion–dipole and charge transfer interaction and hydrogen bonding between unlike molecules (Elangovan & Mullainathan, Citation2013; Alcolea Palafox, Gil, & Núñez, Citation1993). Further, in order to examine the nature and strength of molecular association in these systems, excess adiabatic compressibility and excess intermolecular free length (
) have also been evaluated. The dispersion forces should make a positive contribution to excess values while ion–dipole, dipole–dipole, dipole-induced dipole, charge–transfer interaction, and hydrogen bonding between unlike components should make negative contributions (Fort & Moore, Citation1965). For such systems, the excess adiabatic compressibility and excess intermolecular free length show inverse behavior compared to the ultrasonic velocity. This further supports the molecular association by complex formation. In the case of MgCl2 + P. nigrum system, the negative values of
and
become more negative as concentration and temperature increases due to complex formation. This clearly indicates the presence of strong hydrogen bonding which becomes maximum where the minima of
and
occur. This is in conformity with the earlier results of Fort and Moore (Fort & Moore, Citation1965) and Gour et al. (Gour, Tomar, & Varma, Citation1986) on the study of complex formation in mixtures. The strength of the interaction between the components increases when excess values tend to become increasingly negative. This may be qualitatively interpreted in terms of a closer approach of unlike molecules leading to reductions in compressibility and volume. The excess properties are found to more negative with increasing temperature. The above discussion clearly indicates the occurrence of complex formation between unlike molecules, which is maximum at the concentration indicated by the maxima or the minima corresponding to the respective parameters for the system. (Awasthi & Shukla, Citation2003).
5. Conclusions
It can be concluded from the above experimental data of ultrasonic velocity (U), density (ρ), and viscosity (η) that the interferometer technique requires minimum efforts. It is a direct method and has its own identity and significance in material science, which can give an idea about the effectiveness of solvent. The extraction of P. nigrum is successfully prepared and same is used to reconstitute in an ethanolic molar solution of MgCl2 with different concentration and temperature. On the basis of the above discussion, it is clear that the acoustical parameters and negative excess values hint to the presence of ion–dipole interactions are present between the component molecules in the mixture studied as a function of concentration and temperature. It is also observed that the acoustic parameters and their excess values are highly affected by changes in concentration and temperature. Thus, it can be concluded that in describing the thermal and acoustic parameters plays a vital role in unlike molecules. Molecular association becomes maximum, which is reflected by the ultrasonic investigation. This may be interpreted due to the formation of strong hydrogen bonding resulting in complex formation producing a displacement of electrons and nuclei. The chemical interaction may involve the association due to the solute–solvent and ion–solvent interaction or due to the formation of charge-transfer complexes. Calculated thermoacoustic and their excess parameters also support the existence of drug–solvent interactions. The results obtained from these studies can thus be helpful for pharmacological applications of drugs as well as to understand pharmacokinetics processes such as transport of drug across biological membranes, drug action, and physicochemical properties.
Funding
The authors received no direct funding for this research.
References
- Alcolea Palafox, M., Gil, M., & Núñez, J. (1993). Structure and spectral characteristics of o-aminobenzoic acid by AM1. Vibrational Spectroscopy, 6, 95–105.10.1016/0924-2031(93)87026-P
- Ali, A., Nain, A., & Kamil, M. (1996). Physico-chemical studies of non-aqueous binary liquid mixtures at various temperatures. Thermochimica Acta, 274, 209–221.10.1016/0040-6031(95)02719-X
- Ali, A., Hyder, S., & Nain, A. (1999). Studies on molecular interactions in binary liquid mixtures by viscosity and ultrasonic velocity measurements at 303.15 K. Journal of Molecular Liquids, 79, 89–99.10.1016/S0167-7322(98)00105-6
- Avery, R. L., Pearlman, J., Pieramici, D. J., Rabena, M. D., Castellarin, A. A., Nasir, Ma’an A., ... Patel, A. (2006). Intravitreal bevacizumab (avastin) in the treatment of proliferative diabetic retinopathy. Ophthalmology, 113, 1695–1705.e6.10.1016/j.ophtha.2006.05.064
- Awasthi, A., & Shukla, J. (2003). Ultrasonic and IR study of intermolecular association through hydrogen bonding in ternary liquid mixtures. Ultrasonics, 41, 477–486.10.1016/S0041-624X(03)00127-6
- Babavali, S. F., Shakira, P., Srinivasu, C., & Narendra, K. (2015). Comparative study of theoretical ultrasonic velocities of binary liquid mixtures containing quinoline and mesitylene at temperatures T = (303.15, 308.15, 313.15 and 318.15) K. Karbala International Journal of Modern Science, 1, 172–177.
- Bhandakkar, V., Bhat, V., Chimankar, O., & Asole, A. (2014). Thermo acoustical study of tetrahydrofuran with ethanol using ultrasonic technique at 323 K. Advances in Applied Science Research, 5, 80–85.
- Bhaskar, V., & Patel, P. (2014). Formulation and evaluation of reservoir type transdermal patches of 18-Β-glycyrrhetic acid with piperine as bioenhancer. Indo American Journal of Pharmaceutical Research, 4, 1298–1308.
- Bhatia, S. C., Bhatia, R., & Dubey, G. P. (2010). Thermophysical and sonochemical behavior of binary mixtures of hexan-1-ol with halohydrocarbons at 303.15K. Journal of Molecular Liquids, 152, 39–52.10.1016/j.molliq.2010.01.001
- Blitz, J., & Simpson, G. (1995). Ultrasonic methods of non-destructive testing (Vol. 2). London: Springer Science & Business Media.
- Bradley, M., Ashokkumar, M., & Grieser, F. (2013). Multibubble sonoluminescence in ethylene glycol/water mixtures. The Journal of Physical Chemistry B, 118, 337–343.
- Bronzino, J. D. (1999). Biomedical engineering handbook (Vol. 2). Boca Raton, FL: CRC Press.
- Bruylants, G., Wouters, J., & Michaux, C. (2005). Differential scanning calorimetry in life science: Thermodynamics, stability, molecular recognition and application in drug design. Current Medicinal Chemistry, 12, 2011–2020.10.2174/0929867054546564
- Darbari, G., Richelson, M., & Petrucci, S. (1970). Ultrasonic study of aqueous solutions of ZnCl2: From dilute solutions to hydrated melts. The Journal of Chemical Physics, 53, 859–866.10.1063/1.1674150
- Das, A., & Jha, B. (1992). Thermo-acoustic behaviour of aqueous solutions of some cobalt salts. Acoustics Letters, 15, 165–171.
- Elangovan, S., & Mullainathan, S. (2013). Ultrasonic studies of intermolecular interaction in binary mixture of n-methyl formate with 1-propanol at various temperatures. Indian Journal of Physics, 87, 659–664.10.1007/s12648-013-0288-2
- Fort, R., & Moore, W. (1965). Adiabatic compressibilities of binary liquid mixtures. Transactions of the Faraday Society, 61, 2102–2111.10.1039/tf9656102102
- Gour, S., Tomar, J., & Varma, R. (1986). Study of molecular interactions in binary mixtures using excess thermodynamic parameters. Indian Journal of Pure and Applied Physics, 24, 602–604.
- Gupta, S. C., Prasad, S., Kim, J. H., Patchva, S., Webb, L. J., Priyadarsini, I. K., & Aggarwal, B. B. (2011). Multitargeting by curcumin as revealed by molecular interaction studies. Natural Product Reports, 28, 1937–1955.10.1039/c1np00051a
- Haq, I. (2002). Thermodynamics of drug–DNA interactions. Archives of Biochemistry and Biophysics, 403(1), 1–15.10.1016/S0003-9861(02)00202-3
- Hill, N. E. (1969). Dielectric properties and molecular behaviour (1st ed., Vol. 1, pp. 1–107). New York, NY: Van Nostrand Reinhold.
- Jacobson, B. (1952). Ultrasonic velocity in liquids and liquid mixtures. The Journal of Chemical Physics, 20, 927–928.10.1063/1.1700615
- Jamwal, D. S., & Singh, J. (1993). Effects of piperine on enzyme activities and bioenergetic functions in isolated rat liver mitochondria and hepatocytes. Journal of Biochemical Toxicology, 8, 167–174.10.1002/(ISSN)1522-7146
- Kannappan, V., & Vinayagam, S. C. (2007). Ultrasonic investigation of ion-solvent interactions in aqueous and non-aqueous solutions of transition and inner transition metal ions. Indian Journal of Pure and Applied Physics, 45, 143–150.
- Kannappan, A., Thirumaran, S., & Palani, R. (2009). Volumetric and thermodynamic studies of molecular interactions in ternary liquid mixtures at 303, 308 and 313 K. Journal of Physical Science, 20, 97–108.
- Karplus, M., & McCammon, J. A. (2002). Molecular dynamics simulations of biomolecules. Nature Structural Biology, 9, 646–652.10.1038/nsb0902-646
- Keller, B., Legendziewicz, J., Gliński, J., & Samela, S. (2000). Investigation of the optical and ultrasonic properties of praseodymium and cerium chlorides in nonaqueous solutions. Journal of Alloys and Compounds, 300-301, 334–340.10.1016/S0925-8388(99)00762-8
- Khan, I. (2009). Effect of KCl and KNO3 on partial molal volumes and partial molal compressibilities of some amino acids at different temperatures. International Journal of Thermophysics, 30, 475–489.
- Kincaid, J. F., & Eyring, H. (1938). Free volumes and free angle ratios of molecules in liquids. The Journal of Chemical Physics, 6, 620–629.10.1063/1.1750134
- Logan, D. E. (1989). The Raman noncoincidence effect in dipolar binary mixtures. Chemical Physics, 131, 199–207.10.1016/0301-0104(89)80169-7
- Malasane, P., & Aswar, A. (2005). Thermodynamic and ultrasonic studies of biomolecules in aqueous solutions of metal ion at 303.15 K. Indian journal of chemical technology, 12, 689–694.
- Meghwal, M., & Goswami, T. (2013). Piper nigrum and piperine: An update. Phytotherapy Research, 27, 1121–1130.10.1002/ptr.v27.8
- Min, J., Meng-Xia, X., Dong, Z., Yuan, L., Xiao-Yu, L., & Xing, C. (2004). Spectroscopic studies on the interaction of cinnamic acid and its hydroxyl derivatives with human serum albumin. Journal of Molecular Structure, 692, 71–80.10.1016/j.molstruc.2004.01.003
- Mujumdar, A. M., Dhuley, J. N., Deshmukh, V. K., Raman, P. H., & Naik, S. R. (1990). Anti-inflammatory activity of piperine. Japanese Journal of Medical Science and Biology, 43, 95–100.10.7883/yoken1952.43.95
- Naidu, P. S., & Prasad, K. R. (2004). Ultrasonic velocity and allied parameters in solutions of cypermethrin with xylene and ethanol. Indian Journal of Pure and Applied Physics, 42, 512–517.
- Nalle, P. B., Birajdar, S. D., Shinde, B., Dorik, R., & Jadhav, K. (2016). Effect of drug Piper nigrum on physicochemical properties of zinc chloride at varying concentration and temperature investigated through ultrasonic tool. Cogent Chemistry, 2, Article ID: 1216721.
- Nikam, P., & Hiray, A. (1991). Temperature and concentration dependence of ultrasonic velocity and allied parameters of monochloroacetic acid in ethanol-nitrobenzene mixtures. Indian journal of pure & applied physics, 29, 601–605.
- Nikam, P., Hasan, M., Pawar, T., & Sawant, A. (2004). IPC code: G 01D. Indian journal of pure & applied physics, 42, 172–178.
- Pavai, R. E., Vasantharani, P., & Kannappan, A. (2004). Ultrasonic studies on aqueous ternary electrolytes. Indian Journal of Pure and Applied Physics, 42, 934–936.
- Pawar, N., & Chimankar, O. (2015). Comparative study of ultrasonic absorption and relaxation behavior of polar solute and non-polar solvent. Journal of Pure and Applied Physics, 37, 11–14.
- Pethig, R. (1992). Protein-water interactions determined by dielectric methods. Annual Review of Physical Chemistry, 43, 177–205.10.1146/annurev.pc.43.100192.001141
- Pradhan, S., Dash, S., Moharana, L., & Swain, B. (2012). Molecular interaction parameters of binary mixtures of diethyl ether and apolar solvents using ultrasonic probe. Indian Journal of Pure and Applied Physics, 50, 161–166.
- Priya, C. S., Nithya, S., Velraj, G., & Kanappan, A. (2010). Molecular interactions studies in liquid mixture using ultrasonic technique. International Journal of Advanced Science and Technology, 18, 59–74.
- Rafiqul Islam, M., & Quadri, S. K. (1985). Ultrasonic study of molecular interactions in binary liquid mixtures at various temperatures. Acoustics Letters, 8, 166–176.
- Rahman, M. H., Maruyama, T., Okada, T., Yamasaki, K., & Otagiri, M. (1993). Study of interaction of carprofen and its enantiomers with human serum albumin—I. Biochemical Pharmacology, 46, 1721–1731.10.1016/0006-2952(93)90576-I
- Ram, A., Joseph, D. A., Balachandar, S., & Singh, V. P. (2009). Medicinal plants from Siddha system of medicine useful for treating respiratory diseases. International Journal of Pharmaceuticals Analysis, 1, 20–30.
- Ramteke, J. (2012). Ultrasonic study of molecular interaction in binary liquid mixture containing α-picolin in ethanol at 301.15 K and 305.15 K. Advances in Applied Science Research, 3, 1832–1835.
- Ravichandran, S., & Ramanathan, K. (2010). Acoustical parameters of polyacrylamide with sodium (meta) silicate and potassium silicate solution at 303 K. Polymer Chemistry, 1, 698–701.10.1039/b9py00330d
- Roy, M. N., Jha, A., & Choudhury, A. (2004). Densities, viscosities and adiabatic compressibilities of some mineral salts in water at different temperatures. Journal of Chemical & Engineering Data, 49, 291–296.10.1021/je030205y
- Sarvazyan, A. (1982). Development of methods of precise ultrasonic measurements in small volumes of liquids. Ultrasonics, 20, 151–154.10.1016/0041-624X(82)90032-4
- SingháGill, D. (1995). Ultrasonic velocity, conductivity, viscosity and calorimetric studies of copper (I) and sodium perchlorates in cyanobenzene, pyridine and cyanomethane. Journal of the Chemical Society, Faraday Transactions, 91, 2789–2795.
- Sonar, A., & Pawar, N. (2010). Acoustic and volumetric properties of digoxin and Thiabendazole in 1, 4 Dioxane at 303 K. Journal of Chemistry, 7, 789–794.
- Syal, V., Thakur, S., Chauhan, S., & Sharma, P. (2005). Ultrasonic velocity studies of drug parvon-spas in mixed alcohol–water solvent systems at 25°C. International Journal of Thermophysics, 26, 807–826.10.1007/s10765-005-5579-1
- Tanabe, K., & Hiraishi, J. (1982). Raman linewidth study of molecular interactions in mixtures of hexafluorobenzene with benzene and with mesitylene. Journal of Raman Spectroscopy, 12, 274–277.10.1002/jrs.v12:3
- Thanuja, B., Kanakam, C., & Nithya, G. (2013). Study of molecular interactions in binary mixtures of 2-chloro-4′ methoxy benzoin with various solvents through ultrasonic speed measurements. Indian Journal of Physics, 87, 1225–1231.10.1007/s12648-013-0364-7
- Thirumaran, S., & Priya, D. (2013). Acoustical and excess thermodynamic studies of ternary liquid mixtures of some primary alkanols in mixed binary solvent at varying temperatures. Indian Journal of Pure & Applied Physics, 51, 413–420.
- Trivedi, A., & Mishra, S. (2010). A simple and rapid method for simultaneous estimation of glycyrrhetinic acid and piperine by HPTLC in a herbomineral formulation. Journal of advanced pharmaceutical technology & research, 1, 190–198.
- Uddin, Q., Samiulla, L., Singh, V., & Jamil, S. (2012). Phytochemical and pharmacological profile of Withania somnifera Dunal: A review. Journal of Applied Pharmaceutical Science, 2, 170–175.
- Umaley, K., Pethe, G., & Aswar, A. (2013). Volumetric, viscometric, acoustical, and optical studies of glutamic acid in aqueous zinc and copper chloride solutions. Russian Journal of Physical Chemistry B, 7, 11–22.10.1134/S1990793113010077
- Venkat Reddy, S., Srinivas, P. V., Praveen, B., Hara Kishore, K., China Raju, B., Suryanarayana Murthy, U., & Madhusudana Rao, J. (2004). Antibacterial constituents from the berries of Piper nigrum. Phytomedicine, 11, 697–700.10.1016/j.phymed.2003.04.004
- Victor, P. J., Das, B., & Hazra, D. K. (2001). A Study on the solvation phenomena of some sodium salts in 1,2-dimethoxyethane from conductance, viscosity, ultrasonic velocity, and FT-Raman spectral measurements. The Journal of Physical Chemistry A, 105, 5960–5964.10.1021/jp010510v
- Wang, J., Wu, Y., Xuan, X., & Wang, H. (2002). Ion–molecule interactions in solutions of lithium perchlorate in propylene carbonate+diethyl carbonate mixtures: An IR and molecular orbital study. Spectrochimica Acta Part A: Molecular and Biomolecular Spectroscopy, 58, 2097–2104.10.1016/S1386-1425(01)00686-2
- Zafarani-Moattar, M. T., & Shekaari, H. (2005). Apparent molar volume and isentropic compressibility of ionic liquid 1-butyl-3-methylimidazolium bromide in water, methanol, and ethanol at T=(298.15 to 318.15)K. The Journal of Chemical Thermodynamics, 37, 1029–1035.10.1016/j.jct.2005.01.009