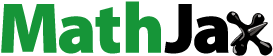
Abstract
A series of trivalent europium-doped M3SiO5 (M = Sr, Ca and Mg) phosphors were synthesized using sol–gel process at 950°C. Samples were further reheated at high temperature to study the effect of reheating on crystal structure and optical characteristics. X-ray diffraction measurement of these materials was carried out to know the crystal structure. Diffraction pattern showed monoclinic structure having space group Cm for Ca3SiO5 materials. However, tetragonal phase with space group P4/ncc was observed for Sr3SiO5 materials. Mg3SiO5 material show mixed diffraction peaks at 950 and 1,150°C. Transmission electron microscopic analysis was used to estimate the particle size of silicates. Photoluminescence emission spectra were recorded to check the luminescence properties of prepared materials. These phosphors exhibited a strong orange-red light under excitation at 395 nm. The prepared phosphors exhibited most intense peak in 610–620 nm region due to the 5D0→7F2 transition of europium (III) ion available in lattice. To overcome the deficiency of red silicates, M3SiO5 materials were explored and they might be integrated with ultraviolet LEDs to generate light which may be suitable for display applications.
Public Interest Statement
Research and development of nanophosphor is a part of rapidly growing nanoscience and nanotechnology. Silicate-based phosphors have attracted significant interest owing to their excellent luminescence characteristics, high chemical and physical stability, and low synthetic cost. Doping of rare earth into the host matrix demonstrate an approach to develop highly efficient and stable nanophosphors for solid-state lighting and display technology. Specially, europium(III) is an effective dopant that emits strong red light due to 5D0→7F0–4 transitions generated under external energy excitation. Europium(III) doped phosphors have wide applicability in the field of color television display, flat panel display, plasma display panels, device indicators, lasers, automobile headlights, etc. and hence they are emerging as an important class of optical materials.
1. Introduction
Most of the commercial available phosphor materials belong to certain host lattices like oxides, silicates, aluminates, sulfides, oxy-sulfides, nitrides etc. Currently, silicate matrices have attracted a lot of interest to develop efficient luminescent materials as they exhibit excellent photoluminescence properties, good chemical stability, and relative ease of preparation than others (Singh et al., Citation2014). Silica-based materials can be explored at general synthetic conditions and their composition, shape, and size can be easily controlled to improve the photoluminescence performance of the phosphor materials (Kang et al., Citation2011).
Nowadays, lighting and display devices are based mainly on white light-emitting diodes (WLEDs) as they are used in a number of applications like device indicators, general illumination, digital cameras, cell phones, LCDs, computer monitors, automobile headlights, etc. (Kang et al., Citation2005; Qiao, Zhang, Ye, Chen, & Guo, Citation2009). These applications are due to the small size, long service life, high efficiency, low voltage, light weight, fast response time, good stability, robustness, adjustable color and energy-saving characteristics of WLEDs (Hsu, Sheng, & Tsai, Citation2009; Jang et al., Citation2008). Silicate-based materials having composition BaSc2Si3O10:Eu2+ (blue) and Ca2MgSi2O7:Eu3+ (orange-red) are significant in the field of WLEDs (Sahu, Bisen, & Brahme, Citation2015; Wang et al., Citation2015). Ultraviolet LED/phosphor combinatorial chemistry explored different phosphor materials to accomplish the purpose of white light generation (Kim, Jeon, Choi, & Park, Citation2005). Phosphors including Ba3MgSi2O8:Eu2+ and MgY4Si3O13:Eu3+ give blue and intense red color when excited with ultraviolet light source (Ci et al., Citation2015; Yonezaki & Takei, Citation2016). Recently, some silicate (Sr2SiO4:Eu2+, Sr3SiO5:Eu2+ and Sr3SiO5:Eu2+, Ba2+) phosphor materials are integrated with InGaN blue LED chip into a single package to generate white light (Park, Choi, Yeon, Lee, & Kim, Citation2006). NUV LED chip-excited tricolor luminescent materials may also be used to produce white light which have high efficiency, good coloration, and low thermal quenching (Li, Wang, Yang, Guo, & Li, Citation2009; Zhang, Xu, Qiu, & Yu, Citation2013). Therefore, it is quite logical to develop efficient red luminescent materials having such characteristics. Near ultraviolet (NUV) LED excited Ba9Y2Si6O24:Ce3+ phosphor material has been developed to emit blue-green light (Brgoch et al., Citation2013). Several silicates of Sr, Ca, Mg, and Ba absorb ultraviolet radiations (Park, Choi, Kim, & Kim, Citation2005) and generate red light when activated with europium (III) ion. Previously, silicate-based materials have been prepared using solid-state method (Kang et al., Citation2011; Sun, Zhang, Zhang, Luo, & Wang, Citation2008). But, materials synthesized using solid-state method require long reaction time and high temperature processing conditions, which correspondingly enhances the particle size of materials (Bisen & Sharma, Citation2016). Repeated milling and washing with chemicals tends to degrade the luminescence property of materials and gives deformed shaped crystals. Sol–gel is an efficient process for the synthesis of silicate phosphors due to homogenous mixing of initial precursors, low reaction temperature, and more homogenous products formation (Singh, Sheoran, Tanwar, & Bhagwan, Citation2016).
In this paper, we report the optical characterization of silicate materials having composition M3-xEuxSiO5 (where M = Sr, Ca and Mg and x = 0.03 mol) prepared using sol–gel method (Singh & Sheoran, Citation2016). Different techniques (PL, XRD, and TEM) were used to study the characteristics of these luminescent materials. Effect of temperature on luminescence properties of these materials has been studied using photoluminescence analysis. Crystal structure of prepared luminescent materials was analyzed using X-ray diffraction study and transmission electron microscopic analysis estimated the particle size of these materials.
2. Experimental details
2.1. Synthesis of nanophosphors
Eu3+ doped M3SiO5 (M = Sr, Ca and Mg) luminescent materials were synthesized using sol–gel technique at 950°C. The chemicals used were mainly Eu(NO3)3·6H2O, Sr(NO3)2, Mg(NO3)2·6H2O, Ca(NO3)2·4H2O, and silica powder. The reagents used were of analytical grade (AR) and purchased from chemical drug house (CDH) and HiMedia. Initially, silica gel was prepared by dissolving a mole of silica powder in concentrated nitric acid solution in a round-bottom flask (RBF). Then, allowed the contents of RBF to stir on a magnetic stirrer for the formation of silica gel which occurred nearly in 10 min. On the other behalf, a stochiometric amount of europium nitrate and corresponding metal nitrates of Sr, Ca, and Mg were mixed homogenously in a silica crucible using distilled water. Then, nitrate mixture of silica crucible was slowly added into the flask containing gel and this mixed solution was again stirred for nearly 30 min. Stirring was done to acquire uniform mixing of precursors. Precursors of the flask were transferred again into the silica crucible which was further warmed on a heating plate for semi-solid paste formation. This semi-solid paste was now put in the furnace at 950°C (as prepared) for 1 h to produce white powders of silicate materials. The resulting white powders were then reheated at a temperature of 1,050 and 1,150°C to check the effect of reheating on the crystal structure and photoluminescence properties of materials. Photographs of as-prepared europium doped silicate materials were taken in the presence and absence of ultraviolet light and shown in the Figure . The synthetic procedure used for material synthesis was also explained with the help of flow chart as given in Figure . Probable chemical reaction between reactants is shown below that gives rise to the final products.(1)
(1)
(2)
(2)
(3)
(3)
2.2. Instrumentation
Crystal structure of phosphor materials was examined from X-ray diffraction pattern recorded using Rigaku Mini Flex 600 diffractometer. CuKα radiation was used for recording the spectrum having a wavelength of 1.540 Ao. Diffraction pattern was recorded from 10 to 70o with a step interval of 0.02o having a scanning speed of 4o/min. Photoluminescence properties were measured at room temperature from 400 to 700 nm using Horiba Jobin YVON Fluorolog Model FL-3–11 Spectrophotometer equipped with Xe-lamp as the source of excitation. Transmission electron micrographs were taken with the help of Hitachi F-7,500 to estimate the particle size of these powdered materials.
3. Results and discussion
3.1. Optical properties
Photoluminescence emission spectra of trivalent europium-doped M3SiO5 (M = Sr, Ca, Mg and x = 0.03 mol) phosphor materials were recorded with the excitation of 395 nm. Luminescence in phosphor materials is generated due to 4f→4f electronic transition of europium (III) ion. Optical properties of phosphor materials get influenced by concentration of dopant ion and temperature employed for reheating of materials. Luminescence intensity of Sr3SiO5 materials enhances with europium concentration up to a limit (3 mol %) but, after that, it starts decreasing due to concentration quenching effect as seen in Figures and . This effect originated due to increase in nonradiative energy transfer between Eu3+ ions which correspondingly decreases the luminescence intensity as the concentration of the Eu3+ ion further increased. Although size and ionic charge issues exists wherever a bivalent metal ion is substituted by trivalent europium ion but, each time variation of metal ion (Sr2+, Ca2+ and Mg2+ having ionic size 1.12 Ao, 0.99 Ao and 0.72 Ao, respectively) in the host lattice, gives rise to a distinct emission spectra as seen in Figure having several peaks originated due to different transitions of Eu3+ (0.95 Ao) ion available in the lattice. The origin of these transitions depends upon the location of activator ion in lattice and type of transition is determined by selection rules. These transitions were illustrated by drawing energy level diagram of europium (III) ion as shown in Figure . These phosphor materials exhibited sharp spectral peaks in 400–700 nm region due to 5D0 →7FJ (J = 1–3) transitions of Eu3+ ion. 5D0 →7F2 is the most intense and electric dipole allowed transition located at 616 nm in Sr3SiO5 (Li et al., Citation2009) and Ca3SiO5 phosphors. In Mg3SiO5 material, this transition is centered at 614 nm which is dominant over 5D0 →7F1 (magnetic dipole transition). Peak positions and corresponding color of these silicate phosphors are reported in Table . Further reheated samples at 1,050 and 1,150°C were used to study the effect of temperature on luminescence intensity of these silicates materials. Remarkable enhancement in luminescence intensity was observed for these silicates with reheating temperature as clear from Figure . Relative emission spectrum was also used to compare the luminescence intensity between the prepared series of materials as in Figure . From the luminescence results, it is clear that highest photoluminescence intensity is observed in case of red emitting Mg3SiO5 material. Emission characteristics of the materials are determined in terms of color coordinate (x and y) values. In phosphor materials, chromaticity coordinates plays an important role in the white light-emitting diode applications. Color coordinates of the M3SiO5: Eu3+ (M = Sr, Ca, Mg and x = 0.03 mol) phosphors are calculated using chromaticity calculator and reported in Table . Values corresponding to these color coordinates are represented in CIE (Commission Internationale de l’Eclairage) triangle that indicates that these materials are emitting light in the orange-red region as shown in Figure .
Figure 3. Photoluminescence emission spectra of Sr3SiO5 materials recorded at 950°C using different concentration of europium ion.
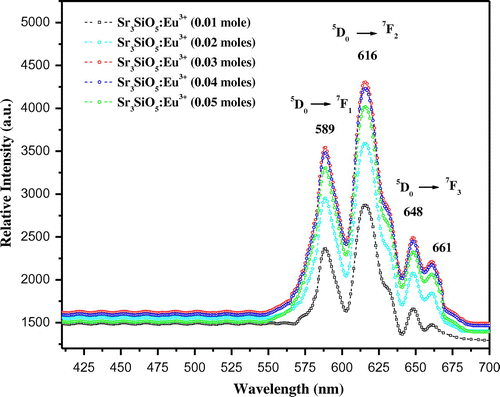
Figure 5. Photoluminescence emission spectra of europium (III) doped (a) Sr3SiO5, (b) Ca3SiO5, and (c) Mg3SiO5 materials at different temperatures.
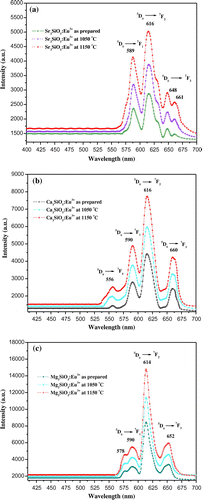
Figure 6. Energy level diagram of europium (III) ion showing different transitions in prepared materials.
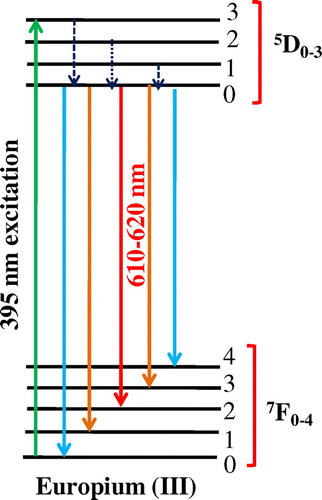
Table 1. Representing different transitions of europium (III) ion and their corresponding color in prepared lattices
Table 2. Showing color coordinates of the Eu3+ doped silicate materials
3.2. Structure determination using X-ray diffraction analysis
X-ray diffraction pattern of the silicate materials prepared using sol–gel technique is presented in the Figure . Sr3SiO5 material synthesized at 950°C has tetragonal form with a space group of P4/ncc. Diffraction pattern of this sample is closely matched with JCPDS No. 26-0984 (Li et al., Citation2009; Zhang et al., Citation2013). Diffraction profile of this material at 1,150°C also exhibits similar polymorphic form with significant enhancement in peak intensity. Dopant ion does not influence the crystal structure of prepared materials. Ca3SiO5 material is known to exhibit seven different polymorphic forms (Song, Lee, Cho, & Ok, Citation2012; Staněk & Sulovský, Citation2002; Taylor & Aldridge, Citation1993). Some notations like TI, TII, TIII, MI, MII, MIII, and R were used for triclinic, monoclinic, and rhombohedral forms of tricalcium silicate (De La Torre, Bruque, Campo, & Aranda, Citation2002). Diffraction peaks of sol–gel-derived Ca3SiO5 material are well consistent with PDF No. 42-551 exhibiting monoclinic (M3) form (Jadhav & Debnath, Citation2011). Although the peaks are intense and more distinct in the phosphor materials prepared using sol–gel method. Eu3+ doped Ca3SiO5 material crystallizes in non-centro symmetric (NCS) monoclinic form possessing a space group of Cm (No. 8) (Song et al., Citation2012). Further reheating at 1,150°C correspondingly enhances the peak intensity of the material. Particle size of these materials is calculated with the help of Debye–Scherrer’s equation:(4)
(4)
Figure 9. X-ray diffraction pattern of europium (III) doped (a) Sr3SiO5, (b) Ca3SiO5, and (c) Mg3SiO5 materials.
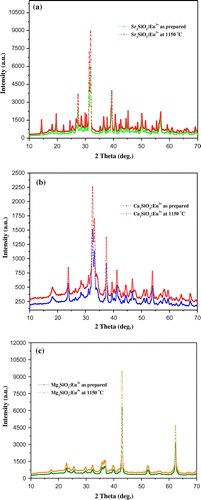
where D is the particle size, λ is the wavelength of X-ray used, β is the full width at half maximum, and θ is the angle of incidence. Particle size has been calculated using most intense peak in XRD pattern of these phosphors which is summarized in Table . Diffraction profile of europium (III) doped Mg3SiO5 material at 950 and 1,150°C is shown in Figure . Literature data were not available before this report to confirm about the crystal structure of Mg3SiO5 material. X-ray pattern shows mixed diffraction peaks of Mg3SiO5 and MgO JCPDS No. 01-1235 (Li, Xu, Li, Liu, & Jia, Citation2014) phosphor.
Table 3. Representing particle size of as prepared europium-doped silicate materials
3.3. Transmission electron microscopic analysis
The morphological features including shape and size of prepared phosphor materials are estimated using transmission electron microscopic analysis. Phosphors particles exhibited nearly spherical shape and they are also aggregated as seen in Figure . TEM micrographs confirmed the size of prepared phosphors in nano-range. The measured particle size of as-prepared silicate materials is reported in Table which is in accordance with particle size calculation from XRD analysis. These luminescent materials are found to be in nano (13–75 nm) range which have great applicability in the field of lighting and display technology.
4. Conclusion
Nanosized M3SiO5 (M = Sr, Ca, Mg and x = 0.03 mol) silicate materials were successfully explored using sol–gel method and their characteristics were studied using different techniques. Emission spectra of these materials exhibited intense peaks in 400–700 nm region due to the different transitions of trivalent europium ion. XRD pattern gave sharp peaks indicating the crystalline structure of prepared materials. Particle size of these materials was calculated from diffraction analysis using Scherrer’s equation. TEM micrographs confirmed the existence of particles size in nano-range. M3SiO5 silicates showed better luminescence properties with doping of europium (III) ion in crystal lattice which emitted red light under 395 nm excitation. Hence, these nanophosphors might be integrated with LED chip to generate red light which could be suitable for lighting applications.
Funding
This work has been financially supported by University Research Scholarship (URS) granted by Maharshi Dayanand University, Rohtak, India.
Acknowledgments
The authors also thanks to Electron Microscopy Department, AIIMS, New Delhi, for providing microscopic analysis facility.
Additional information
Notes on contributors
Devender Singh
Devender Singh is presently working as an assistant professor (Stage-III) of Chemistry at Maharishi Dayanand University, Rohtak, Haryana. He has completed his PhD (2005) in Inorganic Chemistry under the supervision of Ishwar Singh and Sang Do Han (KIER, S. Korea) from the Maharshi Dayanand University, Rohtak, India. His current research interest focuses on light-emitting materials and their fabrication for optoelectronic display applications. He has published more than 62 research papers in well-reputed international journals. Presently, he is a principal investigator for the Major Research Project funded by the University Grant Commission, New Delhi.
References
- Bisen, D. P., & Sharma, R. (2016). Mechanoluminescence properties of SrAl2O4:Eu2+ phosphor by combustion synthesis. Luminescence, 31, 394–400. doi:10.1002/bio.2972
- Brgoch, J., Borg, C. K. H., Denault, K. A., Mikhailovsky, A., DenBaars, S. P., & Seshadri, R. (2013). An efficient, thermally stable cerium-based silicate phosphor for solid state white lighting. Inorganic Chemistry, 52, 8010–8016. doi:10.1021/ic400614r
- Ci, Z., Guan, R., Nie, K., Liu, L., Han, L., Zhang, J., & Wang, Y. (2015). Temperature-sensitive photoluminescence property and energy transfer mechanism in the silicate phosphor MgY4Si3O13:Eu3+. Journal of the American Ceramic Society, 98, 2488–2492. doi:10.1111/jace.13628
- De La Torre, Á. G., Bruque, S., Campo, J., & Aranda, M. A. G. (2002). The superstructure of C3S from synchrotron and neutron powder diffraction and its role in quantitative phase analyses. Cement and Concrete Research, 32, 1347–1356. doi:10.1016/S0008-8846(02)00796-2
- Hsu, W.-H., Sheng, M.-H., & Tsai, M.-S. (2009). Preparation of Eu-activated strontium orthosilicate (Sr1.95SiO4:Eu0.05) phosphor by a sol–gel method and its luminescent properties. Journal of Alloys and Compounds, 467, 491–495. doi:10.1016/j.jallcom.2007.12.014
- Jadhav, R., & Debnath, N. C. (2011). Computation of X-ray powder diffractograms of cement components and its application to phase analysis and hydration performance of OPC cement. Bulletin of Materials Science, 34, 1137–1150. ISSN: 10.1007/s12034-011-0134-0
- Jang, H. S., Yang, H., Kim, S. W., Han, J. Y., Lee, S.-G., & Jeon, D. Y. (2008). White light-emitting diodes with excellent color rendering based on organically capped CdSe quantum dots and Sr3SiO5:Ce3+, Li+ phosphors. Advanced Materials, 20, 2696–2702. doi:10.1002/adma.200702846
- Kang, E.-H., Choi, S.-W., Chung, S. E., Jang, J., Kwon, S., & Hong, S.-H. (2011). Photoluminescence characteristics of Sr3SiO5:Eu2+ yellow phosphors synthesized by solid-state method and pechini process. Journal of The Electrochemical Society, 158, J330–J333. doi:10.1149/2.016111jes
- Kang, H. S., Hong, S. K., Kang, Y. C., Jung, K. Y., Shul, Y. G., & Park, S. B. (2005). The enhancement of photoluminescence characteristics of Eu-doped barium strontium silicate phosphor particles by co-doping materials. Journal of Alloys and Compounds, 402, 246–250. doi:10.1016/j.jallcom.2005.04.143
- Kim, J. S., Jeon, P. E., Choi, J. C., & Park, H. L. (2005). Emission color variation of M2SiO4:Eu2+ (M=Ba, Sr, Ca) phosphors for light-emitting diode. Solid State Communications, 133, 187–190. doi:10.1016/j.ssc.2004.10.017
- Li, L.-X., Xu, D., Li, X.-Q., Liu, W.-C., & Jia, Y. (2014). Excellent fluoride removal properties of porous hollow MgO microspheres. New Journal of Chemistry, 38, 5445–5452. doi:10.1039/c4nj01361a
- Li, P., Wang, Z., Yang, Z., Guo, Q., & Li, X. (2009). Preparation and luminescence characteristics of Sr3SiO5:Eu3+ phosphor for white LEDS. Chinese Science Bulletin, 53, 974–977. doi:10.1007/s11434-008-0044-8
- Park, J. K., Choi, K. J., Kim, K. N., & Kim, C. H. (2005). Investigation of strontium silicate yellow phosphors for white light emitting diodes from a combinatorial chemistry. Applied Physics Letters, 87, 031108(1–3). doi: 10.1063/1.1984103
- Park, J. K., Choi, K. J., Yeon, J. H., Lee, S. J., & Kim, C. H. (2006). Embodiment of the warm white-light-emitting diodes by using a Ba2+ codoped Sr3SiO5:Eu phosphor. Applied Physics Letters, 88, 043511(1–3). doi:10.1063/1.2166471
- Qiao, Y., Zhang, X., Ye, X., Chen, Y., & Guo, H. (2009). Photoluminescent properties of Sr2SiO4:Eu3+ and Sr2SiO4:Eu2+ phosphors prepared by solid state reaction method. Journal of Rare Earths, 27, 323–326. doi:10.1016/S1002-0721(08)60243-4
- Sahu, I. P., Bisen, D. P., & Brahme, N. (2015). Europium doped di-calcium magnesium di-silicate orange red emitting phosphor by solid state reaction method. Journal of Radiation Research and Applied Sciences, 8, 381–388. doi:10.1016/j.jrras.2015.02.007
- Singh, D., & Sheoran, S. (2016). Synthesis and luminescent characteristics of M3Y2Si3O12:Eu3+ (M = Ca, Mg, Sr and Ba) nanomaterials for display applications. Journal of Materials Science: Materials in Electronics, 1–12. doi:10.1007/s10854-016-5405-5
- Singh, D., Sheoran, S., Tanwar, V., & Bhagwan, S. (2016). Optical characteristics of Eu(III) doped MSiO3 (M = Mg, Ca, Sr and Ba) nanomaterials for white light emitting applications. Journal of Materials Science: Materials in Electronics, 1–11. doi: 10.1007/s10854-016-5914-2
- Singh, D., Tanwar, V., Bhagwan, S., Simantilleke, A. P., Singh, I., & Kadyan, P. S. (2014). Synthesis and optical characterization of terbium doped M2SiO4 nanophosphors. Advanced Science Letters, 20, 1531–1534. doi:10.1166/asl.2014.5522
- Song, J., Lee, D. W., Cho, Y.-H., & Ok, K. M. (2012). Solid state synthesis, characterization, and nonlinear optical properties of a monoclinic tricalcium silicate, Ca3SiO5. Bulletin of the Korean Chemical Society, 33, 2423–2426. doi:10.5012/bkcs.2012.33.7.2423
- Staněk, T., & Sulovský, P. (2002). The influence of the alite polymorphism on the strength of the Portland cement. Cement and Concrete Research, 32, 1169–1175. doi:10.1016/S0008-8846(02)00756-1
- Sun, X., Zhang, J., Zhang, X., Luo, Y., & Wang, X.-J. (2008). Long lasting yellow phosphorescence and photostimulated luminescence in Sr3SiO5:Eu2+ and Sr3SiO5:Eu2+, Dy3+ phosphors. Journal of Physics D: Applied Physics, 41, 195414 (1-4). doi:10.1088/0022-3727/41/19/195414
- Taylor, J. C., & Aldridge, L. P. (1993). Full-profile Rietveld quantitative XRD analysis of Portland cement: Standard XRD profiles for the major phase tricalcium silicate (C3S:3CaO.SiO2). Powder Diffraction, 8, 138–144. doi:10.1017/S0885715600018054
- Wang, Q., Zhu, G., Xin, S., Ding, X., Xu, J., Wang, Y., & Wang, Y. (2015). A blue-emitting Sc silicate phosphor for ultraviolet excited light-emitting diodes. Physical Chemistry Chemical Physics, 17, 27292–27299. doi:10.1039/c5cp02899j
- Yonezaki, Y., & Takei, S. (2016). Photochromism and emission–color change in Ba3MgSi2O8–based phosphors. Journal of Luminescence, 173, 237–242. doi:10.1016/j.jlumin.2016.01.026
- Zhang, X., Xu, X.-H., Qiu, J.-B., & Yu, X. (2013). Effects of Li+ on photoluminescence of Sr3SiO5:Sm3+ red phosphor. Chinese Physics B, 22, 097801(1-5). doi:10.1088/1674-1056/22/9/097801