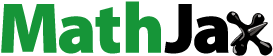
Abstract
The current research was designed for selective green synthesis of N-(substituted)-2-(5-(1-(4-nitrophenylsulfonyl)piperidin-4-yl)-1,3,4-oxadiazol-2-ylthio)acetamide hybrids and their pharmacological applications to overcome the enzymatic diseases. Environment friendly sodium hydride as an activator was employed to catalyze the reaction for the synthesis of target compounds. Structural characterization was performed using spectroscopic techniques (1H-NMR, 13C-NMR, IR, and EI-MS). All of the synthesized compounds were screened for acetylcholinesterase (AChE) and urease inhibition potential. Three compounds, 6a, 6k, and 6p, were found to be the best inhibitors of AChE enzyme and the whole array of compounds showed urease inhibition potential even better than the reference. Eserine was the reference standard for AChE inhibition and thiourea for urease inhibition. The computational and BSA binding studies were performed to explore the binding modes and interaction between synthesized compounds and respective enzymes. Molecular docking studies explained the presence of some hydrophobic interactions and have good correlation with calculated free energy of binding (DG binding). The finding of these new unique compounds having high potential to inhibit the activity of urease enzyme as compared to standard showing the prospective to replace the existing drugs and surprisingly effective addition of highly active drug discovery in the field of pharmacology against described enzyme.
Public Interest Statement
The increased level of resistance of disease causing enzymes for the available drugs is an alarming issue in the whole world. The current research is the successful addition in the field of pharmacy to combat the enzymatic issues of the humanity even in better, effective, economical and safe style by replacing the existing candidates applied for the mentioned enzymatic issues.
1. Introduction
Heterocyclic systems embedded with nitrogen and oxygen are a useful source for biological and pharmacological application to combat the deadly microbes having threatening effect for humanity (Citation1). Compounds based on oxadiazole rings found to be active as antibacterial (Citation2, 3), antifungal (Citation4, 5), anticonvulsant, anti-enzymatic (Citation6), and anticancer (Citation7). In the same way, piperidine-based compounds are also the potential source of pharmacological applications. The combined effect of oxadiazole and piperidine compounds in one unit gave the best impetus for the search of highly active biological and pharmacological agents.
Computational chemistry and biological applications have potential for designing new active drug systems and speeding up the drug discovery system. Molecular docking system is very effective to understand the active site for interaction between drugs and receptors and also helpful to understand the binding orientation of drug, its affinity and activities for target protein (Citation8, 9). Generally, the proteins are the basic molecules in the living organisms responsible for the effective action of various drugs which could be justified through the binding of typical drug with the proteins. The binding of drugs with the protein gave the idea about the effectiveness of the drug, so the studies of synthesized drugs–protein binding interaction are the active area of research (Citation10). Comprehensive BSA binding studies have been carried out on oxadiazole molecules and quenching mechanism of fluorescence by synthesized compounds (Citation11). Similarly, the interaction of synthesized compounds with BSA was studied at different pH values and concentrations of synthesized compounds (Citation12).
Keeping in view the biological applications of 1,3,4-oxadiazoles and piperidine and continuous effort for the search of new therapeutic molecules, we hereby report the synthesis, biological applications, and docking studies of hybrids of N-(substituted)-2-(5-(1-(4-nitrophenylsulfonyl)piperidin-4-yl)-1,3,4-oxadiazol-2-ylthio)acetamide having both 1,3,4-oxadiazoles and piperidine under one construct to enhance their biological applications.
2. Results and discussion
The current research work has been designed in the search of biologically active compounds. To attain the peak of success of this search, we synthesized a series of N-(substituted)-2-(5-(1-(4-nitrophenylsulfonyl)piperidin-4-yl)-1,3,4-oxadiazol-2-ylthio)acetamide having a wide range biological applications. The synthesis of targeted compounds was initiated by the synthesis of sulfonamide through 4-nitrobenzene sulfonyl chloride and piperidine termed as compound 1, which was further modified into hydrazide the key product of this series. Hydrazide was converted into 1,3,4-oxadiazole and finally by the reaction 1,3,4-oxadiazole and a variety of acetamides to synthesize highly biologically active anti-enzymatic series of compounds (6a–p). All the synthesized compounds were characterized by spectroscopic techniques like 1H-NMR, 13C-NMR, EI-MS, and IR to confirm the established structure of synthesized compounds. All the compounds were synthesized in good yield and purity of excellent level. In the next stage compounds were screened for their anti-enzymatic studies and their percent inhibition against specific enzymes and IC50 values were recorded to understand the effectiveness of these compounds against these enzymes. Almost all the compounds were more and highly active as compared to the standard which was a great achievement in search of new drugs for the treatment of enzymatic diseases.
The morphology of synthesized compounds was well justified as well as their structures were corroborated with aid of spectral data obtained by the detailed spectroscopic studies. Compound 6a was selected for comprehensive discussion as single compound. Compound 6a was acquired as a light brown amorphous solid with 83% yield and its molecular formula and molecular mass was justified by the molecular ion peak obtained at m/z 503 which help to erect the molecular formula as C21H21N5O6S2 on the basis of fragmentation pattern by EI-MS. The absorption peaks obtained at 3350 (N–H), 3071 (Ar C–H), 1674 (C=N), 1662 (C=O), 1599 (Ar C=C), 1523 (N=O), 1432 (S=O), 1075 (C–N), 605 (C–S) confirm the presence of their respective functional groups accordingly in aliphatic as well as aromatic regions. Further evidences for the structural confirmation were availed by 1H-NMR spectral studies. The peaks of two doublets resonating at 7.56 (d, J = 7.6 Hz, 2H, H-3″, H-5″) and 6.65 (d, J = 8.7 Hz, 2H, H-2″, H-6″) are because of four protons at present at position 3″, 5″ and 2″, 6″, respectively, verifying the aromatic ring of 4-nitrobenzene sulfonyl. The signals of one doublet and two triplets resonating at 7.36 (d, J = 8.7 Hz, 2H, H-2‴, H-6‴), 7.31 (t, J = 8.3 Hz, 1H, H-4‴) and 7.07 (t, J = 7.4 Hz, 2H, H-3‴, H-5‴) because of five protons confirm the presence of aromatic ring of amide. N–H group was firmly confirmed by the single peak obtained at 10.36 ppm. A signal of singlet obtained at 6.06 (s, 2H, H-1″″) is the evident for the presence of CH2directly attached with sulfur atom and carbonyl carbon of amide. Nine protons of piperidine ring confirmed by the presence of five multiplet signals resonating at 3.47–3.45 (m, 2H, Heq-2′, Heq-6′), 3.00–2.96 (m, 1H, H-4′), 2.39–2.35 (m, 2H, Hax-2′, Hax-6′), 2.05–2.00 (m, 2H, Heq-3′, Heq-5′), 1.72–1.66 (m, 2H, Hax-3′, Hax-5′). 13C-NMR studies made us able to identify the presence of total number carbon atoms and their respective positions. The signals obtained at 169.22 (C-2) and 164.77 (C-5) of two quaternary carbon of five-membered oxadiazole ring, at 162.77 (C-2″″) of quaternary carbon of carbonyl group, at 153.14 (C-4″) and 138.59 (C-1″) of two quaternary carbon of 4-nitrobenzenesulfonyl group, 129.42 (C-1‴) of quaternary carbon of aromatic ring of amide group confirm all the quaternary carbons present in the whole structure as back bone. The four carbons of 4-nitrobenzenesulfonyl group and five carbons of aromatic ring of amide group were confirmed by the presence signals at 128.84 (C-3″, C-5″), 123.62 (C-2‴, C-6‴), 119.85 (C-4‴), and 119.09 (C-3‴, C-5‴). Five carbons of piperidine ring were confirmed by the presence of peaks at 44.85 (C-2′, C-6′), 36.65 (C-4′), 27.86 (C-3′, C-5′) while carbon of CH2 group was identified by the presence of peak at 31.29 (C-1″″). The doublet peak of two H-3″, H-5″ appeared down field as compared doublet signal of two protons H-2″, H-6″ because of -NO2 group attached at para position of benzene ring of 4-nitrobenzensulfonyl group which is more electronegative and caused deshieding of the protons in its vicinity as compared to the -SO2 present at position one of the same ring. There exist five multiplet signals for the piperidine ring, the signals of 2H, Heq-2′, Heq-6′ were present down field as compared to axial protons signal present at same position because of directly attached nitrogen and SO2 which brings the electron density from these two proton and force the signals of these protons to appear towards more down filed at 3.47–3.45 ppm as compare to the signals of all other protons available at piperidine ring. Normally the signals of aliphatic alkyl group appear at 1–2 ppm in 1H NMR spectrum, but –CH2– of the compound under discussion exist at 6.06 ppm as singlet because it is directly attached with heteroatom and a carbon of carbonyl group. 13C-NMR studies to identify all the carbon atoms were carried out at same facts and pattern as that of 1H-NMR which works like a back bone study of the structure elucidation. Taking in view all the spectroscopic evidences, functional groups studies, fragmentation pattern, and computational chemistry, the compound 6a was confirmed with name of 2-(5-(1-(4-nitrophenylsulfonyl)piperidin-4-yl)-1,3,4-oxadiazol-2-ylthio)-N-phenylacetamide. The similar studies were carried out for the structural confirmation of all the synthesized compounds (6a–p) (Table ).
Table 1. Different substituents of aralkyl/phenyl/aryl group
2.1. AChE inhibition studies
Acetylcholinesterase inhibition activity was measured as percent inhibition and IC50 values (Table ). All the compounds were tested for the inhibition of AChE, a few of them were moderate IC50 values and inhibition capacity. But majority of compounds were found to be very active having high % inhibition values. Compounds 6a, 6k, and 6p were found to be very active acetylcholinesterase inhibitor having IC50 values 62.54 ± 0.12, 47.69 ± 0.13, and 28.54 ± 0.13 and % inhibition values of 85.36 ± 0.16, 88.72 ± 0.16, and 89.75 ± 0.18, respectively. The inhibition potential was enhanced as we move from 6a to 6p might be attributed because of different type of substitution at aromatic ring of amide group. The compound 6a has only aromatic ring there is no substitution at aromatic ring of amide group, as the ethyl group substituted at the pare position of aromatic ring of compound 6k inhibition potential was increased that was further enhanced in compound 6p by the substitution of NO2 group at ortho position of aromatic ring of amide. The discussed pattern of substitution can be summarized as: less inhibition potential showed by compound 6a might be due to the N-substituted aromatic ring showing hydrophobic interaction with AChE. While in compound 6k the inhibition potential was increased because of presence of ethyl group at para of N-substituted aromatic ring. The highest potential against the mentioned enzyme possessed by compound 6p was might be due to the presence of NO2 group showing hydrogen bonding and van der waal’s interaction with the amino residue and active sites of AChE. These interactions of compound 6p could be responsible to deactivate the action of AChE enzyme.
Table 2. AChE inhibition studies
2.2. Urease inhibition studies
Aims and objectives of our current studies were efficiently achieved by the urease inhibition studies of all the synthesized compounds. The whole array of unique synthesized compounds was found to be very effective and excellent inhibitor of urease enzyme. The high % inhibition and low IC50 values confirmed the high activity potential of these compounds. The results were compared with thiourea and have been represented in Table . All the discussed compared studies made us able to state that the standard was highly less effective as compared to the synthesized compounds which can efficiently replace the standard and could be utilized for inhibition of urease activity with less cost and no side effect of these green synthetic bio-organic compounds (Table ).
Table 3. Anti-urease activity
2.3. Molecular docking
To validate the surflex docking procedure, ligand donepezil was extracted from AChE-donepezil complex (crystal structure, PDBID: 4EY7) and re-docked to the binding site of AChE. The docked poses were compared with the experimentally determined binding mode of donepezil in AChE. Molecular docking results of the top three ligand poses were found to be almost overlapped to the crystallographic conformation of donepezil in AChE, with root-mean-square deviation (RMSD) between predicted and experimental confirmation not more than 0.67 Å. This indicates that our docking procedure is reliable.
Furthermore, three oxadiazoles 6p, 6a, and 6e (Figure ), were scrutinized based on their inhibitory potency for AChE. 6p and 6a are highly and moderately active, whereas, 6e exhibits lowest inhibitory potency for AChE, while it differs from 6p only by the absence of NO2 group at ortho positon.
Figure 1. Obtained binding modes of ligands in the main binding site of Acetylcholinesterase. (A) Superimposing of compounds 6p (Purple), 6a (Green) and 6e (Yellow) docked to AChE. (B) Binding mode of compound 6p in AChE main binding site. (C) Binding mode of compound 6e in AChE main binding site. (D) Binding mode of compound 6a in AChE main binding site.
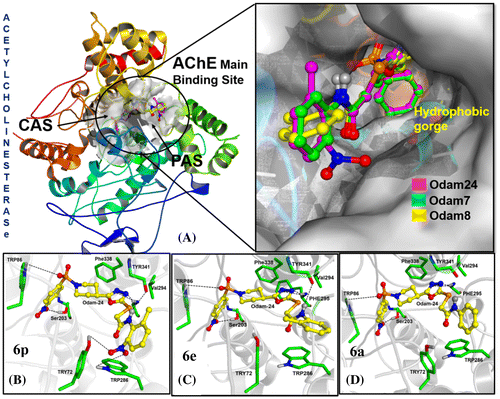
The top ranked poses for each of ligands were selected based on the CS core. The selected (Surflex’s) docked poses ware then graphically visualized using MOLCAD (SYBYL-X) to analyze ligand–protein interactions and to inspect whether the docked ligands are in correct orientation or not. Interestingly, all compounds attained similar geometrical conformations in AChE main active site to the experimentally determined ligand conformation in 4EY7 (Figure (A)). All three ligands were found to establish H-bond interactions with Phe295 near gorge region. As shown in Figure (B), the most active compound 6p extends deeply into the two main binding sites of AChE. The CAS and PAS are connected by a deep, hydrophobic gorge. The piperidine ring of G1 enables hydrophobic interactions with Phe338 and sulfonyl oxygen accept an H-bond from TRP86 in the CAS. Meanwhile, the NH2 of 6p was able to establish H-bond interaction backbone carbonyl oxygen atom of TYR341 in PAS region. Moreover, the oxygen atom at NO2 group in 6-methyl-nitrobenzen ring can form additional hydrogen bond with the –OH of TYR72 and amino group of TRP286, which is consistent with experimentally determined biological activity.
Both compounds 6e and 6a, Figure (C) and (D), respectively, acquired a similar binding pattern to 6p with exception of H-bond with Tyr72 and TRP286 due to absence of NO2 group. The graphical (Figure (C)) analysis illustrate that replacement of NO2 group of 6p with methyl group in 6e is not well accommodated in solvent exposed region and TYR72 is propelled to upward direction. These drastic conformational changes in 6e–AChE complex may results in weakening of overall binding interactions in 6e–AChE complex.
2.4. BSA binding studies
Proteins are primary molecules to be targeted by the drugs for their transportation as well as effectiveness. Understandings about the drugs-BSA binding is very useful to understand the effectiveness of the particular drug as the blood protein play very authentic role in manners of drugs pharmacology, pharmacokinetics, efficiency, and distribution in the cell along with metabolic studies. The effectiveness of the drug depends upon the balance between bound and unbound drug. The binding of drugs with plasma proteins may depends upon the number of binding sites because the proteins molecules are flexible in nature and drugs are transported as complex with serum albumin. The current studies designed for the evaluation of binding of synthesized compounds with bovine serum albumin to know the effects of various substituents on binding. The binding efficiency was measured by flourometric titration of BSA along with different concentrations of synthesized compounds. A few compounds 6a, 6k, 6l, 6p, and 6m were evaluated for their BSA binding studies. All the tested compounds showed the binding with BSA as calculated by their binding constant (Table ). The numbers of binding sites were also calculated. In the current series of synthesized compounds, the compound 6a was found to display the highest binding constant as 3.23 × 107 which was might be due to the presence of un-substituted ring of amide group. Presence of ethyl and methyl at ortho position and presence of ethyl group at para position in the N-substituted aromatic ring of compound 6m and 6k might be responsible to cause a decrease in the binding constant such as 1.62 × 105, 1.05 × 104, respectively. The presence of a nitro and methyl group at ortho position of N-substituted aromatic ring of compound 6p was found to decrease its binding ability (Figure and Scheme ).
Table 4. Binding constant and number of binding site of compounds warfarin and ibuprofen with BSA at 298 K
Figure 2. Fluorescence spectra of BSA in presence of different amounts of selected compounds (6k, 6l, 6m, and 6p) at 295 nm and 298 K (inset graph corresponding to the Stern-Volmer plot).
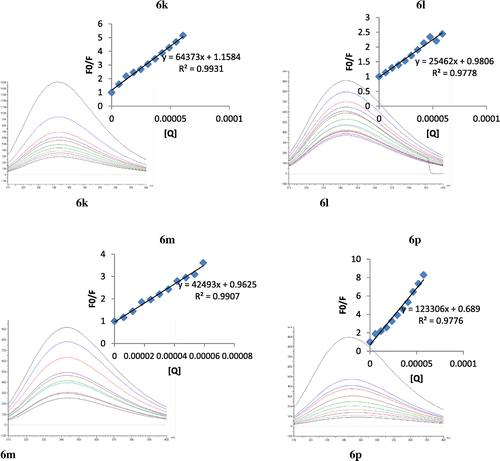
Scheme 1. General scheme for the synthesis of 2-(5-(1-(4-nitrophenylsulfonyl)piperidin-4-yl)-1,3,4-oxadiazol-2-ylthio)-N-(substituted)acetamide (6a–p). (A) 15% Na2CO3 solution, H2O, stir for 8 h. (B) N2H4.H2O, EtOH, reflux for 5 h. (C) KOH (s), EtOH, reflux for 6 h. (D) BrCH2COBr, 15% Na2CO3 solution, H2O, stir for 2 h. (E) DMF, NaH, stir for 24 h.
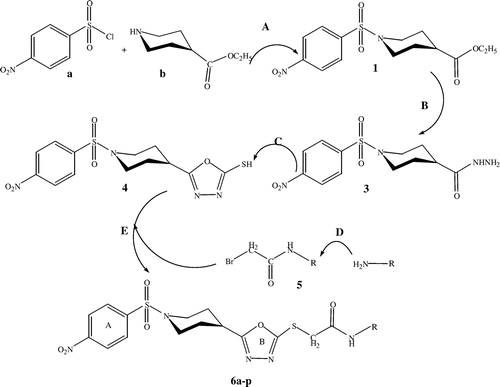
3. Conclusion
Herein we report the successful multistage synthesis of compounds, starting with robustly designed pharmacological based searching of unique compounds in conjunction with structural characterization followed by molecular docking and BSA binding studies. Molecular docking and BSA binding studies were made in aimed to justify the active parts of compounds and effect of various substituents on binding abilities of synthesized compounds responsible for effective pharmacological applications. All the synthesized compounds were employed for acetyl cholinesterase inhibition, and urease inhibition studies. All the compounds demonstrated the outstanding profile as active inhibitor of acetyl cholinesterase, with exceptions of few compounds. But more and most efficiently these compounds can be used as drugs for urease inhibition as they all compounds were found to possess more inhibition character against the urease enzyme as compared to the standard used. The position of nitrogen and oxygen atoms in all of the synthesized compounds based on heterocyclic systems submerged in single unit are optimal to ensure the inhibition efficacy and further recommended for their in vivo studies in future.
4. Materials and methods
4.1. Materials
Jasco-320-A spectrometer was used to record the IR spectra to identify the basic functional group present the in the compounds. Electron impact mass spectroscopic studies were carried by JMS-HX-110 spectrometer. 1H-NMR and 13C-NMR studies were done by using Bruker spectrometer operating at 600 & 150 MHz. Griffin and George melting point apparatus was employed to identify he melting points of synthesized compound with help of open capillary tube. All the compounds were synthesized in good yield and the purity was monitored and confirmed by thin layer chromatography coated with silica gel G-25-UV254 aluminum plates.
4.2. Synthesis of ethyl 1-(4-nitrophenylsulfonyl)piperidin-4-carboxylate (1)
4-Nitrobenzene sulfonyl chloride (0.390 mol; a) and equimolar ethyl isonipecotate (b) were subjected to react in aqueous medium in the presence of sodium carbonate to yield maximum amount of ethyl 1-(4-nitrophenylsulfonyl)piperidin-4-carboxylate at room temperature. The reaction thoroughly monitored by TLC to confirm the completion of reaction and assure the purity synthesized compound. After the completion of reaction the pH of reaction mixture was maintained at neutral by adding dil. HCl. In order to obtained compound in the form of precipitates, chilled distilled water was added in the reaction mixture and aged for three hours. The obtained precipitates were filtered washed and dried for further utilization.
4.3. Synthesis of 1-(4-nitrophenylsulfonyl)piperidine-4-carbohydrazide (2)
Compound 2 was synthesized by refluxed reaction between hydrazinium hydroxide (5 mL) and compound 1 (0.015 mol) in methanol solvent. Product formation was confirmed by thin layer chromatographic analysis. As the reaction completed, the excess of methnol was evaporated followed by the addition of chilled water drop wise after the methanol evaporation. After addition of 10 mL water into the reaction flask, it was aged into refrigerator for maximum precipitation and finally filtered, washed, and dried the precipitates for upcoming stage of synthesis.
4.4. Synthesis of 5-(1-(4-nitrophenylsulfonyl)piperidin-4-yl)-1,3,4-oxadiazol-2-thiol (3)
Equimolar amounts of KOH (0.0304 mol) and compound 2 (0.0304 mol) were dissolved in 10 mL of ethanol followed by the addition of CS2 (0.0608 mol) and refluxed for 5 h. Thin layer chromatography was used to analyze the completion of reaction. As the reaction completed excess chilled distilled water was added into the reaction mixture. Then the pH of reaction mixture was maintained at 5 by the addition of Dil. HCl for protonation at sulfur atom directly attached with oxdiazole ring, so that maximum amount of target compound obtained. Finally, the precipitates were filtered washed and dried.
4.5. Synthesis of N-(substituted)-2-bromoacetamides (5a–p)
Aralkyl/aryl amines (4a–p; 0.02 mol) were stirred with 2-bromoacetyl bromide (0.02 mol) in distilled water for 1 h. The pH was adjusted to 9–10 by 15% Na2CO3 solutions during the reaction. The formed precipitates were filtered, washed with distilled water, and dried.
4.6. General procedure for the synthesis of target compounds (6a–p)
A series of 2-(5-(1-(4-nitrophenylsulfonyl)piperidin-4-yl)-1,3,4-oxadiazol-2-ylthio)-N-(substituted)acetamide (6a–p) in the presence LiH and 10 mL of N,N-dimethyl form amide as reaction medium 20 h. TLC supported to confirm the purity and completion of reaction. After completion of reaction, chilled water was added with continuous stirring to obtain maximum product. The obtained product in the form of precipitates was filtered washed and dried and a few were extracted in the presence of chloroform and obtained in the form of extracts.
4.7. Acetyl cholinesterase inhibition assay
The pre-reported method was adopted to measure the inhibition of acetyl cholinesterase enzyme responsible for the breakdown of acetylcholine (Citation13). Test compound (0.5 mM well−1), a buffer solution of Na2HPO4 buffer (pH 7.7) and acetyl cholinesterase (0.005 mM well−1) were mixed and absorption was measured at 405 nm. Then the whole mixture was incubated for 20 min at 30°C. As 10 μL of 0.5 mM well−1 acetylthiocholine iodide and 10 μL DTNB (0.5 mM well−1) were added to the reaction mixture, reaction was started. After incubation period the absorption was again measured at 405 nm by using 96-well plate reader Synergy HT, Biotek, USA. Eserine (0.5 mM well-1) as a standard and percent inhibition was calculated by following the formula given below:
The IC50 was calculated was calculated with the assistance of EZ-Fit Enzyme kinetics software (Perrella Scientific Inc. Amherst, USA).
4.8. Urease inhibition assay
A volume of 985 microliter mixture having test compound (0 to 100 μL), urea (850 μL), and phosphate buffer (100 mM, pH 7.4) was made to access the anti-urease activity by the reported method (Citation14). The reaction started by the addition of 15 μL of urease enzyme. 500 μL of solution A having 0.5 g phenol and 2.5 mg of sodium nitro prusside in 50 mL of distilled water and equi-volume solution B (contained of 250 mg sodium hydroxide and 820 μL of sodium hypochlorite 5% in 50 mL of distilled water) were used to measure the extent of reaction after 60 min at 37°C for 30 min. Before the inhibition of urease activity was taken as 100% control activity. The similar formula was employed to calculate the % inhibition as for acetyl cholinesterase enzyme. GraphPad Prism 5 software was used to calculate the IC50 values.
4.9. Molecular docking simulations
The 3D-structures of the studied inhibitors were constructed by Sybyl-X1.3/ SKETCH module (Citation15), and then minimized by Tripos force field with GasteigereHückel atomic charge. To investigate the differences in the binding modes of most active and least active inhibitors of studied enzymes, the Surflex-Dock module included in the SYBYL-X 1.3 (Citation16) was used to generate putative poses of docked ligands. Crystal structures of recombinant human AChE in complex with donepezil (PDB entry: 4EY7 (Citation17) retrieved from the RCSB Protein Data Bank (PDB), was used as a starting point for further calculations. The complex was then carefully analyzed to confirm the chemical accuracy using structure preparation tools implemented in biopolymer module of SYBYL-X 1.3. Missing hydrogens were added, charges were applied, and atom types were assigned according to AMBER 7 FF99 force field. Consequently, the energy was minimized to prevent steric clashes using the Powell algorithm with a convergence gradient of 0.5 kcal (mol Å)−1 for 1000 cycles. The in silico build structures of most active compound 6p was inserted into the active site of AChE (4EY7) to replace donepezil. The resulting conformation of ligand 6p was used as starting point for protomol generation (an idealized active site). All ligands were then individually docked into the binding pocket of AChE, using Surflex-Dock module included in the SYBYL-X 1.3. Default parameters were used to determine the extent of protomol (threshold = 0.50 and bloat = 0). C-score was turned on and all other parameters were kept at default. The best pose was ranked on the basis of C-score and the ligand–protein interactions.
4.10. BSA-synthesized compounds interactions using fluorescence measurements
In order to calculate the BSA quenching constant, a solution of BSA (3 mL, 3 μM) was titrated with solution of synthesized compounds of different concentrations ranging from 1–3 mg/mL followed by their fluorescence determination. The solutions were excited at 295 nm. The intensity of BSA solution was recorded at 336 nm at 298 K with and without synthesized compounds (Citation18).
4.11. Spectral data of synthesized compounds
4.11.1. 2-(5-(1-(4-Nitrophenylsulfonyl)piperidin-4-yl)-1,3,4-oxadiazol-2-ylthio)-N-phenylacetamide (6a)
Light brown amorphous solid; Yield: 83%; M.P.: 187–1186°C; Molecular formula: C21H21N5O6S2; Molecular mass: 503.55 gmol−1;IR (KBr, υmax, cm−1): 3350 (N–H), 3071 (Ar C–H), 1674 (C=N), 1662 (C=O), 1599 (Ar C=C), 1523 (N=O), 1432 (S=O), 1075 (C–N), 605 (C–S); 1H-NMR (CDCl3, 600 MHz, δ (ppm)): 10.36 (s, 1H, N–H), 7.56 (d, J = 7.6 Hz, 2H, H-3″, H-5″), 7.36 (d, J = 8.7 Hz, 2H, H-2‴, H-6‴), 7.31 (t, J = 8.3 Hz, 1H, H-4‴), 7.07 (t, J = 7.4 Hz, 2H, H-3‴, H-5‴), 6.65 (d, J = 8.7 Hz, 2H, H-2″, H-6″), 6.06 (s, 2H, H-1″″), 3.47–3.45 (m, 2H, Heq-2′, Heq-6′), 3.00–2.96 (m, 1H, H-4′), 2.39–2.35 (m, 2H, Hax-2′, Hax-6′), 2.05–2.00 (m, 2H, Heq-3′, Heq-5′), 1.72–1.66 (m, 2H, Hax-3′, Hax-5′); 13C-NMR (150 MHz, DMSO): δ 169.22 (C-2), 164.77 (C-5), 162.77 (C-2″″), 153.14 (C-4″), 138.59 (C-1″), 129.42 (C-1‴), 128.84 (C-3″, C-5″), 123.62 (C-2‴, C-6‴), 119.85 (C-4‴), 119.09 (C-3‴, C-5‴), 129.8 (C-2″, C-6″), 44.85 (C-2′, C-6′), 36.65 (C-4′), 31.29 (C-1″″), 27.86 (C-3′, C-5′); EIMS (m/z): 503 [M]+, 370 [C13H14N4O5S2]+, 338 [C13H14N4O5S]+, 295 [C12H13N3O4S]+, 270 [C11H14N2O4S]+, 165 [C8H7NOS]+, 134 [C8H8NO]+, 123 [C6H5NO2]+, 78 [C6H6]+, 66 [C5H6]+, 52 [C4H4]+.
4.11.2. N-(2,4-dimethylphenyl)-2-(5-(1-(4-nitrophenylsulfonyl)piperidin-4-yl)-1,3,4-oxadiazol-2-ylthio)acetamide (6b)
Radish sticky product; Yield: 77%; Molecular formula: C23H25N5O6S2; Molecular mass: 531.60 gmol−1; IR (KBr, υmax, cm−1): 3341 (N–H), 3074 (Ar C–H), 1677 (C=N), 1664 (C=O), 1595 (Ar C=C), 1522 (N=O), 1434 (S=O), 1075 (C–N), 604 (C–S); 1H-NMR (CDCl3, 600 MHz, δ (ppm)): 9.24 (s, 1H, N–H), 7.52 (d, J = 8.04 Hz, 1H, H-6‴), 7.36 (d, J = 7.4 Hz, 2H, H-3″, H-5″), 7.19 (d, J = 7.8 Hz, 1H, H-5‴), 7.08 (s, 1H, H-3‴), 6.65 (d, J = 7.5 Hz, 2H, H-2″, H-6″), 6.07 (s, 2H, H-1″″), 3.51–3.49 (m, 2H, Heq-2′, Heq-6′), 2.89 (s, 3H, H-3″″), 2.73 (s, 3H, H-4″″), 2.38–2.33 (m, 1H, H-4′), 2.29–2.26 (m, 2H, Hax-2′, Hax-6′), 2.16–2.02 (m, 2H, Heq-3′, Heq-5′), 1.70–1.68 (m, 2H, Hax-3′, Hax-5′); EIMS (m/z): 531 [M]+, 370 [C13H14N4O5S2]+, 338 [C13H14N4O5S]+, 295 [C12H13N3O4S]+, 270 [C11H14N2O4S]+, 193 [C10H11NOS]+, 162 [C10H12NO]+, 123 [C6H5NO2]+, 106 [C8H10]+, 78 [C6H6]+, 52 [C4H4]+.
4.11.3. N-(3,4-dimethylphenyl)-2-(5-(1-(4-nitrophenylsulfonyl)piperidin-4-yl)-1,3,4-oxadiazol-2-ylthio)acetamide (6c)
Off white amorphous solid; Yield: 86%; M.P.: 153–154°C;Molecular formula: C23H25N5O6S2; Molecular mass: 531.60 gmol−1; IR (KBr, υmax, cm−1): 3344 (N–H), 3076 (Ar C–H), 1679 (C=N), 1667 (C=O), 1597 (Ar C=C), 1525 (N=O), 1438 (S=O), 1077 (C–N), 606 (C–S); 1H-NMR (CDCl3, 600 MHz, δ (ppm)): 10.20 (s, 1H, N–H), 7.35 (d, J = 8.6 Hz, 2H, H-3″, H-5″), 7.32 (s, 1H, H-2‴), 7.26 (d, J = 7.8 Hz, 1H, H-6‴), 7.06 (d, J = 8.0 Hz, 1H, H-5‴), 6.65 (d, J = 8.6 Hz, 2H, H-2″, H-6″), 6.06 (s, 2H, H-1″″), 3.48–3.46 (m, 2H, Heq-2′, Heq-6′), 2.36–2.34 (m, 1H, H-4′), 2.23–2.20 (m, 2H, Hax-2′,Hax-6′), 2.17 (s, 3H, H-3″″), 2.16 (s, 3H, H-4″″), 2.05–2.03 (m, 4H, H-3′, H-5′); EIMS (m/z): 531 [M]+, 370 [C13H14N4O5S2]+, 338 [C13H14N4O5S]+, 295 [C12H13N3O4S]+, 270 [C11H14N2O4S]+, 193 [C10H11NOS]+, 162 [C10H12NO]+, 123 [C6H5NO2]+, 106 [C8H10]+, 78 [C6H6]+, 52 [C4H4]+.
4.11.4. N-(3,5-dimethylphenyl)-2-(5-(1-(4-nitrophenylsulfonyl)piperidin-4-yl)-1,3,4-oxadiazol-2-ylthio)acetamide (6d)
Dark brown sticky product; Yield: 79%; Molecular formula: C23H25N5O6S2; Molecular mass: 531.60 gmol−1; IR (KBr, υmax, cm−1): 3349 (N–H), 3078 (Ar C–H), 1678 (C=N), 1661 (C=O), 1598 (Ar C=C), 1527 (N=O), 1435 (S=O), 1072 (C–N), 602 (C–S); 1H-NMR (CDCl3, 600 MHz, δ (ppm)): 9.26 (s, 1H, N–H), 7.36 (d, J = 8.7 Hz, 2H, H-3″, H-5″), 7.09 (s, 2H, H-2‴, H-6‴), 7.07 (s, 1H, H-4‴), 6.65 (d, J = 8.7 Hz, 2H, H-2″, H-6″), 6.06 (s, 2H, H-1″″), 3.51–3.49 (m, 2H, Heq-2′, Heq-6′), 2.39–2.37 (m, 1H, H-4′), 2.31–2.28 (m, 2H, Hax-2′,Hax-6′), 2.24 (s, 3H, H-3″″), 2.22 (s, 3H, H-4″″), 2.12–2.11 (m, 2H, Heq-3′, Heq-5′), 2.03–1.98 (m, 2H, Hax-3′, Hax-5′); EIMS (m/z): 531 [M]+, 370 [C13H14N4O5S2]+, 338 [C13H14N4O5S]+, 295 [C12H13N3O4S]+, 270 [C11H14N2O4S]+, 193 [C10H11NOS]+, 162 [C10H12NO]+, 123 [C6H5NO2]+, 106 [C8H10]+, 78 [C6H6]+, 52 [C4H4]+.
4.11.5. 2-(5-(1-(4-Nitrophenylsulfonyl)piperidin-4-yl)-1,3,4-oxadiazol-2-ylthio)-N-o-tolylacetamide (6e)
Oily extract; Yield: 80%; Molecular formula: C22H23N5O6S2; Molecular mass: 517.58 gmol−1;IR (KBr, υmax, cm−1): 3344 (N–H), 3082 (Ar C–H), 1675 (C=N), 1671 (C=O), 1587 (Ar C=C), 1526 (N=O), 1431 (S=O), 1072 (C–N), 601 (C–S); 1H-NMR (CDCl3, 600 MHz, δ (ppm)): 9.33 (s, 1H, N–H), 7.36 (d, J = 8.7 Hz, 2H, H-3″, H-5″), 7.19 (d, J = 7.4 Hz, 1H, H-5‴), 7.15 (t, J = 7.7 Hz, 1H, H-5‴), 7.02 (d, J = 7.3 Hz, 1H, H-3‴), 6.99 (t, J = 7.3 Hz, 1H, H-4‴), 6.65 (d, J = 8.7 Hz, 2H, H-2″, H-6″), 6.05 (s, 2H, H-1″″), 3.51–3.39 (m, 2H, Heq-2′, Heq-6′), 2.73 (s, 3H, H-3″″), 2.41–2.38 (m, 1H, H-4′), 2.17–2.16 (m, 2H, Hax-2′,Hax-6′), 2.05–2.04 (m, 2H, Heq-3′, Heq-5′), 1.73–1.70 (m[‘, 2H, Hax-3′, Hax-5′); 13C-NMR (150 MHz, DMSO): δ 162.30 (C-2), 161.62 (C-5), 160.77 (C-2″″), 153.12 (C-4″), 138.56 (C-1″), 130.51 (C-1‴), 129.81 (C-2‴), 129.40 (C-3″, C-5″), 126.70 (C-6‴), 125.50 (C-5‴), 123.42 (C-4‴), 116.70 (C-3‴), 112.76 (C-2″, C-6″), 44.90 (C-2′, C-6′), 35.75 (C-4′), 31.23 (C-1″″), 28.07 (C-3′, C-5′), 17.81 (C-3″″); EIMS (m/z): 517 [M]+, 370 [C13H14N4O5S2]+, 338 [C13H14N4O5S]+, 295 [C12H13N3O4S]+, 270 [C11H14N2O4S]+, 179 [C9H9NOS]+, 148 [C9H10NO]+, 123 [C6H5NO2]+, 92 [C7H8]+, 78 [C6H6]+, 52 [C4H4]+.
4.11.6. 2-(5-(1-(4-Nitrophenylsulfonyl)piperidin-4-yl)-1,3,4-oxadiazol-2-ylthio)-N-m-tolylacetamide (6f)
Off white amorphous solid; Yield: 85%; M.P.: 164–163°C; Molecular formula: C22H23N5O6S2; Molecular mass: 517.58 gmol−1;IR (KBr, υmax, cm−1): 3352 (N–H), 3085 (Ar C–H), 1670 (C=N), 1672 (C=O), 1585 (Ar C=C), 1525 (N=O), 1432 (S=O), 1078 (C–N), 603 (C–S); 1H-NMR (CDCl3, 600 MHz, δ (ppm)): 10.82 (s, 1H, N–H), 7.49 (s, 1H, H-2‴), 7.46 (d, J = 8.7 Hz, 2H, H-3″, H-5″), 7.22 (t, J = 7.8 Hz, 1H, H-5‴), 7.09–7.07 (m, 1H, H-6‴), 6.89 (d, J = 7.7 Hz, 1H, H-4‴), 6.54 (d, J = 7.2 Hz, 2H, H-2″, H-6″), 6.06 (s, 2H, H-1″″), 3.81–3.80 (m, 4H, H-2′, H-6′), 2.28–2.26 (m, 1H, H-4′), 2.24 (s, 3H, H-3″″), 2.20–2.08 (m, 4H, H-3′,H-5′); EIMS (m/z): 517 [M]+, 370 [C13H14N4O5S2]+, 338 [C13H14N4O5S]+, 295 [C12H13N3O4S]+, 270 [C11H14N2O4S]+, 179 [C9H9NOS]+, 148 [C9H10NO]+, 123 [C6H5NO2]+, 92 [C7H8]+, 78 [C6H6]+, 52 [C4H4]+.
4.11.7. 2-(5-(1-(4-Nitrophenylsulfonyl)piperidin-4-yl)-1,3,4-oxadiazol-2-ylthio)-N-p-tolylacetamide (6g)
Radish extract; Yield: 84%; Molecular formula: C22H23N5O6S2; Molecular mass: 517.58 gmol−1; IR (KBr, υmax, cm−1): 3356 (N–H), 3081 (Ar C–H), 1679 (C=N), 1678 (C=O), 1586 (Ar C=C), 1528 (N=O), 1430 (S=O), 1076 (C–N), 604 (C–S); 1H-NMR (CDCl3, 600 MHz, δ (ppm)): 10.24 (s, 1H, N–H), 7.41 (d, J = 8.4 Hz, 2H, H-3″, H-5″), 7.37 (d, J = 9.0 Hz, 2H, H-2‴, H-6‴), 7.12 (d, J = 8.3 Hz, 2H, H-3‴, H-5‴), 6.65 (d, J = 8.7 Hz, 2H, H-2″, H-6″), 6.06 (s, 2H, H-1″″), 3.55–3.49 (m, 2H, Heq-2′, Heq-6′), 2.28 (s, 3H, H-3″″), 2.41–2.39 (m, 1H, H-4′), 2.14–2.05 (m, 2H, Hax-2′,Hax-6′), 1.90–1.86 (m, 2H, Heq-3′, Heq-5′), 1.73–1.70 (m, 2H, Hax-3′, Hax-5′); 13C-NMR (150 MHz, DMSO): δ 161.18 (C-2), 159.68 (C-5), 158.14 (C-2″″), 153.14 (C-4″), 138.51 (C-1″), 136.29 (C-1‴), 130.38 (C-4‴), 129.41 (C-3″, C-5″), 129.34 (C-2‴, C-6‴), 112.74 (C-2″, C-6″), 116.81 (C-3‴, C-5‴), 45.00 (C-2′, C-6′), 36.64 (C-4′), 31.15 (C-1″″), 28.07 (C-3′, C-5′), 20.25 (C-3″″); EIMS (m/z): 517 [M]+, 370 [C13H14N4O5S2]+, 338 [C13H14N4O5S]+, 295 [C12H13N3O4S]+, 270 [C11H14N2O4S]+, 179 [C9H9NOS]+, 148 [C9H10NO]+, 123 [C6H5NO2]+, 92 [C7H8]+, 78 [C6H6]+, 52 [C4H4]+.
4.11.8. Methyl 2-(2-(5-(1-(4-nitrophenylsulfonyl)piperidin-4-yl)-1,3,4-oxadiazol-2 ylthio)acetamido)benzoate (6h)
Dark brown; Yield: 87%; M.P.: 137–136°C; Molecular formula: C23H23N5O8S2; Molecular mass: 561.59 gmol−1; IR (KBr, υmax, cm−1): 3358 (N–H), 3084 (Ar C–H), 1681 (C=N), 1674 (C=O), 1588 (Ar C=C), 1527 (N=O), 1433 (S=O), 1074 (C–N), 608 (C–S); 1H-NMR (CDCl3, 600 MHz, δ (ppm)): 11.01 (s, 1H, N–H), 8.20 (d, J = 7.8 Hz, 1H, H-3‴), 7.92 (d, J = 7.8 Hz, 1H, H-6‴), 7.61 (t, J = 8.7 Hz, 1H, H-4‴), 7.36 (d, J = 9.1 Hz, 2H, H-3″, H-5″), 7.24 (t, J = 8.1 Hz, 1H, H-5‴), 6.64 (d, J = 8.7 Hz, 2H, H-2″, H-6″), 6.05 (s, 2H, H-1″″), 4.26 (s, 3H, H-3″″), 3.47–3.46 (m, 2H, Heq-2′, Heq-6′), 2.47–2.37 (m, 1H, H-4′), 2.37–2.35 (m, 2H, Hax-2′,Hax-6′), 2.03–2.01 (m, 2H, Heq-3′, Heq-5′), 1.90–1.70 (m, 2H, Hax-3′, Hax-5′); EIMS (m/z): 561 [M]+, 370 [C13H14N4O5S2]+, 338 [C13H14N4O5S]+, 295 [C12H13N3O4S]+, 270 [C11H14N2O4S]+, 223 [C10H9NO3S]+, 192 [C10H10NO3]+, 136 [C8H8O2]+, 123 [C6H5NO2]+, 92 [C7H8]+, 78 [C6H6]+, 52 [C4H4]+.
4.11.9. N-(2-ethoxyphenyl)-2-(5-(1-(4-nitrophenylsulfonyl)piperidin-4-yl)-1,3,4-oxadiazol-2-ylthio)acetamide (6i)
Dark brown amorphous solid; Yield: 83%; M.P.: 143–142°C; Molecular formula: C22H23N5O7S2; Molecular mass: 533.58 gmol−1; IR (KBr, υmax, cm−1): 3357 (N–H), 3087 (Ar C–H), 1684 (C=N), 1679 (C=O), 1585 (Ar C=C), 1525 (N=O), 1434 (S=O), 1079 (C–N), 605 (C–S); 1H-NMR (CDCl3, 600 MHz, δ (ppm)): 9.76 (s, 1H, N–H), 8.08 (d, J = 7.5 Hz, 1H, H-3‴), 7.37 (d, J = 8.7 Hz, 2H, H-3″, H-5″), 7.08 (d, J = 8.8 Hz, 1H, H-6‴), 6.65 (d, J = 8.7 Hz, 2H, H-2″, H-6″), 6.06 (s, 2H, H-1″″), 4.30 (q, J = 6.2 Hz, 2H, H-3″″), 4.30 (t, J = 5.6 Hz, 3H, H-4″″), 3.54–3.49 (m, 2H, Heq-2′, Heq-6′), 2.71–2.69 (m, 1H, H-4′), 2.47–2.43 (m, 2H, Hax-2′,Hax-6′), 2.06–2.01 (m, 2H, Heq-3′, Heq-5′), 1.89–1.80 (m, 2H, Hax-3′, Hax-5′); EIMS (m/z): 533 [M]+, 370 [C13H14N4O5S2]+, 338 [C13H14N4O5S]+, 295 [C12H13N3O4S]+, 270 [C11H14N2O4S]+, 195 [C9H9NO2S]+, 164 [C9H10NO2]+, 123 [C6H5NO2]+, 108 [C7H8O]+, 78 [C6H6]+, 52 [C4H4]+.
4.11.10. N-(2-ethylphenyl)-2-(5-(1-(4-nitrophenylsulfonyl)piperidin-4-yl)-1,3,4-oxadiazol-2-ylthio)acetamide (6j)
Dark brown amorphous solid: 89%; M.P.: 124–125°C; Molecular formula: C22H25N5O6S2; Molecular mass: 531.60 gmol−1; IR (KBr, υmax, cm−1): 3362 (N–H), 3090 (Ar C–H), 1680 (C=N), 1669 (C=O), 1582 (Ar C=C), 1529 (N=O), 1437 (S=O), 1070 (C–N), 602 (C–S); 1H-NMR (CDCl3, 600 MHz, δ (ppm)): 10.08 (s, 1H, N–H), 7.38 (d, J = 7.7 Hz, 2H, H-3″, H-5″), 7.35–7.32 (m, 1H, H-3‴), 7.19 (d, J = 7.8 Hz, 1H, H-6‴), 7.11 (t, J = 7.4 Hz, 1H, H-5‴), 6.76 (d, J = 8.7 Hz, 2H, H-2″, H-6″), 6.64 (t, J = 8.4 Hz, 1H, H-4‴), 6.05 (s, 2H, H-1″″), 3.35 (q, J = 3.4 Hz, 2H, H-3″″), 3.34–3.33 (m, 2H, Heq-2′, Heq-6′), 2.48–2.46 (m, 1H, H-4′), 2.43 (t, J = 7.5 Hz, 3H, H-4″″), 2.25–2.24 (m, 2H, Hax-2′,Hax-6′), 1.72–1.71 (m, 2H, Heq-3′, Heq-5′), 1.69–1.68 (m, 2H, Hax-3′, Hax-5′); EIMS (m/z): 531 [M]+, 370 [C13H14N4O5S2]+, 338 [C13H14N4O5S]+, 295 [C12H13N3O4S]+, 270 [C11H14N2O4S]+, 193 [C10H11NOS]+, 162 [C10H12NO]+, 123 [C6H5NO2]+, 106 [C8H10]+, 78 [C6H6]+, 52 [C4H4]+.
4.11.11. N-(4-ethylphenyl)-2-(5-(1-(4-nitrophenylsulfonyl)piperidin-4-yl)-1,3,4-oxadiazol-2-ylthio)acetamide (6k)
Yellow amorphous solid; Yield: 81%; M.P.: 161–162°C; Molecular formula: C23H25N5O6S2; Molecular mass: 531.60 gmol−1; IR (KBr, υmax, cm−1): 3364 (N–H), 3089 (Ar C–H), 1690 (C=N), 1671 (C=O), 1577 (Ar C=C), 1531 (N=O), 1439 (S=O), 1078 (C–N), 609 (C–S); 1H-NMR (CDCl3, 600 MHz, δ (ppm)): 10.27 (s, 1H, N–H), 7.45 (d, J = 7.7 Hz, 2H, H-3″, H-5″), 7.34 (d, J = 8.7 Hz, 2H, H-2‴, H-6‴), 7.15 (d, J = 8.4 Hz, 2H, H-3‴, H-5‴), 6.64 (d, J = 8.4 Hz, 2H, H-2″, H-6″), 6.05 (s, 2H, H-1″″), 3.51–3.46 (m, 2H, Heq-2′, Heq-6′), 3.05–2.96 (m, 1H, H-4′), 2.55 (q, J = 7.5 Hz, 2H, H-3″″), 2.38–2.37 (m, 2H, Hax-2′, Hax-6′), 2.03–2.00 (m, 2H, Heq-3′, Heq-5′), 1.70–1.67 (m, 2H, Hax-3′, Hax-5′); EIMS (m/z): 531 [M]+, 370 [C13H14N4O5S2]+, 338 [C13H14N4O5S]+, 295 [C12H13N3O4S]+, 270 [C11H14N2O4S]+, 193 [C10H11NOS]+, 162 [C10H12NO]+, 123 [C6H5NO2]+, 106 [C8H10]+, 78 [C6H6]+, 52 [C4H4]+.
4.11.12. N-(4-ethoxyphenyl)-2-(5-(1-(4-nitrophenylsulfonyl)piperidin-4-yl)-1,3,4-oxadiazol-2-ylthio)acetamide (6l)
Dark brown amorphous solid; Yield: 89%; M.P.: 155–156°C; Molecular formula: C23H25N5O7S2; Molecular mass: 547.60 gmol−1; IR (KBr, υmax, cm−1): 3363 (N–H), 3088 (Ar C–H), 1691 (C=N), 1672 (C=O), 1576 (Ar C=C), 1523 (N=O), 1438 (S=O), 1081 (C–N), 601 (C–S); 1H-NMR (CDCl3, 600 MHz, δ (ppm)): 10.21 (s, 1H, N–H), 7.45 (d, J = 7.7 Hz, 2H, H-3″, H-5″), 7.35 (d, J = 8.7 Hz, 2H, H-2‴, H-6‴), 6.88 (d, J = 9.0 Hz, 2H, H-3‴, H-5‴), 6.65 (d, J = 8.7 Hz, 2H, H-2″, H-6″), 6.06 (s, 2H, H-1″″), 3.39 (q, J = 6.9 Hz, 2H, H-3″″), 3.47–3.45 (m, 2H, Heq-2′, Heq-6′), 2.98 (br.s, 1H, H-4′), 2.39–2.35 (m, 2H, Hax-2′, Hax-6′), 2.03–2.01 (m, 2H, Heq-3′, Heq-5′), 1.72–1.68 (m, 2H, Hax-3′, Hax-5′), 1.31 (t, J = 6.9 Hz, 3H, H-4″″); 13C-NMR (150 MHz, DMSO): δ 169.21 (C-2), 164.20 (C-5), 162.80 (C-2″″), 154.70 (C-4″), 153.14 (C-1″), 140.22 (C-4‴), 131.63 (C-1‴), 129.93 (C-3″, C-5″), 120.64 (C-2‴, C-6‴), 114.43 (C-3‴, C-5‴), 112.73 (C-2″, C-6″), 63.06 (C-3″″), 44.86 (C-2′, C-6′), 36.56 (C-4′), 31.28 (C-1″″), 27.87 (C-3′, C-5′), 31.28 (C-4″″); EIMS (m/z): 547 [M]+, 370 [C13H14N4O5S2]+, 338 [C13H14N4O5S]+, 295 [C12H13N3O4S]+, 270 [C11H14N2O4S]+, 209 [C10H11NO2S]+, 178 [C10H12NO2]+, 123 [C6H5NO2]+, 122 [C8H10O]+, 78 [C6H6]+, 52 [C4H4]+.
4.11.13. N-(2-ethyl-6-methylphenyl)-2-(5-(1-(4-nitrophenylsulfonyl)piperidin-4-yl)-1,3,4-oxadiazol-2-ylthio)acetamide (6m)
Yellow amorphous solid; Yield: 85%; M.P.: 167–168°C; Molecular formula: C24H27N5O6S2; Molecular mass: 545.63 gmol−1; IR (KBr, υmax, cm−1): 3368 (N–H), 3086 (Ar C–H), 1690 (C=N), 1671 (C=O), 1573 (Ar C=C), 1524 (N=O), 1437 (S=O), 1083 (C–N), 605 (C–S); 1H-NMR (CDCl3, 600 MHz, δ (ppm)): 9.64 (s, 1H, N–H), 7.36 (d, J = 8.7 Hz, 2H, H-3″, H-5″), 7.12 (t, J = 7.4 Hz, 1H, H-4‴), 7.06 (d, J = 8.4 Hz, 2H, H-3‴, H-5‴), 6.65 (d, J = 8.7 Hz, 2H, H-2″, H-6″), 6.06 (s, 2H, H-1″″), 3.47–3.43 (m, 2H, Heq-2′, Heq-6′), 3.01 (br.s, 1H, H-4′), 2.48 (q, J = 7.6 Hz, 2H, H-4″″), 2.45–2.40 (m, 2H, Hax-2′, Hax-6′), 2.08 (s, 3H, H-3″″), 2.06–2.04 (m, 2H, Heq-3′, Heq-5′), 1.75–1.69 (m, 2H, Hax-3′, Hax-5′), 1.03 (t, J = 7.5 Hz, 3H, H-5″″); EIMS (m/z): 545 [M]+, 370 [C13H14N4O5S2]+, 338 [C13H14N4O5S]+, 295 [C12H13N3O4S]+, 270 [C11H14N2O4S]+, 207 [C11H13NOS]+, 176 [C11H14NO]+, 123 [C6H5NO2]+, 120 [C9H12]+, 78 [C6H6]+, 52 [C4H4]+.
4.11.14. N-(2-ethoxyphenyl)-2-(5-(1-(4-nitrophenylsulfonyl)piperidin-4-yl)-1,3,4-oxadiazol-2-ylthio)acetamide (6n)
Dark brown amorphous solid; Yield: 83%; M.P.: 143–142°C; Molecular formula: C22H23N5O7S2; Molecular mass: 533.58 gmol−1; IR (KBr, υmax, cm−1): 3357 (N–H), 3087 (Ar C–H), 1684 (C=N), 1679 (C=O), 1585 (Ar C=C), 1525 (N=O), 1434 (S=O), 1079 (C–N), 605 (C–S); 1H-NMR (CDCl3, 600 MHz, δ (ppm)): 9.76 (s, 1H, N–H), 8.08 (d, J = 7.5 Hz, 1H, H-3‴), 7.37 (d, J = 8.7 Hz, 2H, H-3″, H-5″), 7.08 (d, J = 8.8 Hz, 1H, H-6‴), 6.65 (d, J = 8.7 Hz, 2H, H-2″, H-6″), 6.06 (s, 2H, H-1″″), 4.30 (q, J = 6.2 Hz, 2H, H-3″″), 4.30 (t, J = 5.6 Hz, 3H, H-4″″), 3.54–3.49 (m, 2H, Heq-2′, Heq-6′), 2.71–2.69 (m, 1H, H-4′), 2.47–2.43 (m, 2H, Hax-2′,Hax-6′), 2.06–2.01 (m, 2H, Heq-3′, Heq-5′), 1.89–1.80 (m, 2H, Hax-3′, Hax-5′); 13C-NMR (150 MHz, DMSO): δ 170.2 (C-2), 164.2 (C-5), 162.8 (C-2″″), 154.7 (C-4″), 153.1 (C-1″), 140.2 (C-2‴), 131.6 (C-1‴), 129.9 (C-3″, C-5″), 121.6 (C-6‴), 120.6 (C-3‴), 119.4 (C-4‴), 117.9 (C-5‴), 113.7 (C-2″, C-6″), 63.6 (C-3″″), 44.6 (C-2′, C-6′), 36.5 (C-4′), 31.2 (C-1″″), 27.8 (C-3′, C-5′), 14.6 (C-4″″); EIMS (m/z): 533 [M]+, 370 [C13H14N4O5S2]+, 338 [C13H14N4O5S]+, 295 [C12H13N3O4S]+, 270 [C11H14N2O4S]+, 195 [C9H9NO2S]+, 164 [C9H10NO2]+, 123 [C6H5NO2]+, 108 [C7H8O]+, 78 [C6H6]+, 52 [C4H4]+.
4.11.15. N-benzyl-2-(5-(1-(4-nitrophenylsulfonyl)piperidin-4-yl)-1,3,4-oxadiazol-2-ylthio)acetamide (6o)
Reddish sticky product; Yield: 78%; Molecular formula: C22H23N5O6S2; Molecular mass: 517.58 gmol−1; IR (KBr, υmax, cm−1): 3366 (N–H), 3088 (Ar C–H), 1692 (C=N), 1673 (C=O), 1572 (Ar C=C), 1523 (N=O), 1433 (S=O), 1082 (C–N), 607 (C–S); 1H-NMR (CDCl3, 600 MHz, δ (ppm)): 7.98 (t, J = 12.4 Hz, 1H, N–H), 7.37 (d, J = 9.2 Hz, 2H, H-3″, H-5″), 7.34 (d, J = 8.6 Hz, 2H, H-2‴, H-6‴), 7.32 (t, J = 8.2 Hz, 2H, H-3‴, H-5‴), 7.25–7.23 (m, 1H, H-4‴), 6.64 (d, J = 8.6 Hz, 2H, H-2″, H-6″), 6.06 (s, 2H, H-1″″), 4.32 (d, J = 6.18 Hz, 2H, H-3″″), 3.49–3.46 (m, 2H, Heq-2′, Heq-6′), 2.38–2.36 (m, 1H, H-4′), 2.24–2.22 (m, 2H, Hax-2′,Hax-6′), 1.98–1.95 (m, 2H, Heq-3′, Heq-5′), 1.72–1.64 (m, 2H, Hax-3′, Hax-5′); EIMS (m/z): 517 [M]+, 370 [C13H14N4O5S2]+, 338 [C13H14N4O5S]+, 295 [C12H13N3O4S]+, 270 [C11H14N2O4S]+, 179 [C9H9NOS]+, 148 [C9H10NO]+, 123 [C6H5NO2]+, 79 [C6H7]+, 66 [C5H6]+, 52 [C4H4]+.
4.11.16. N-(2-methyl-6-nitrophenyl)-2-(5-(1-(4-nitrophenylsulfonyl)piperidin-4-yl)-1,3,4-oxadiazol-2-ylthio)acetamide (6p)
Yellow amorphous solid; Yield: 86%; M.P.: 182–181°C; Molecular formula: C22H22N6O8S2; Molecular mass: 562.58 gmol−1; IR (KBr, υmax, cm−1): 3369 (N–H), 3086 (Ar C–H), 1691 (C=N), 1672 (C=O), 1575 (Ar C=C), 1527 (N=O), 1433 (S=O), 1087 (C–N), 608 (C–S); 1H-NMR (CDCl3, 600 MHz, δ (ppm)): 10.28 (s, 1H, N–H), 7.90 (d, J = 8.1 Hz, 1H, H-3‴), 7.75–7.72 (m, 1H, H-4‴), 7.62–7.60 (m, 1H, H-5‴), 7.55 (d, J = 7.3 Hz, 1H, H-6‴), 7.39 (d, J = 8.0 Hz, 2H, H-3″, H-5″), 6.66 (d, J = 8.7 Hz, 2H, H-2″, H-6″), 6.06 (s, 2H, H-1″″), 3.48–3.46 (m, 2H, Heq-2′, Heq-6′), 2.53–2.52 (m, 1H, H-4′), 2.42–2.39 (m, 2H, Hax-2′, Hax-6′), 2.04–2.01 (m, 2H, Heq-3′, Heq-5′), 1.71–1.70 (m, 2H, Hax-3′, Hax-5′); EIMS (m/z): 562 [M]+, 370 [C13H14N4O5S2]+, 338 [C13H14N4O5S]+, 295 [C12H13N3O4S]+, 270 [C11H14N2O4S]+, 224 [C9H8N2O3S]+, 193 [C9H9N2O3]+, 137 [C7H7NO2]+, 123 [C6H5NO2]+, 78 [C6H6]+, 52 [C4H4]+.
Funding
The authors received no direct funding for this research.
Acknowledgement
Authors are thankful to Higher Education Commission (HEC) of Pakistan for financial assistance regarding spectral analysis of synthesized compounds.
Additional information
Notes on contributors
Aziz-ur Rehman
Aziz-ur-Rehman, the corresponding author, is working as Associate Professor in GC University, Lahore, Pakistan. He has published more than 200 research papers in peer-reviewed national and international journals. He has been awarded national research productivity award three times. He has guided six PhD students and five are working under him. The main focus of our research group is to develop simple and efficient synthetic strategies for the synthesis of new bioactive organic heterocyclic compounds under Organo-Pharmaceutical Research. Hira Khalid and Sabina Jhaumeer Laulloo are co-authors who conducted the BSA binding analysis of the synthesized compounds. The structural corroboration of synthesized compounds was conducted through spectral data provided by Syed Adnan Ali Shah.
References
- Omar, F.A.; Mahfouz, N.M.; Rahman, M.A. Synthesis and Antimicrobial Activity of 2-substituted [4-(1,3,4-oxadiazol-2-yl methyl)] phthalazin-1(2 H)-one Derivatives. Eur. J. Med. Chem. 1996, 31, 819–825.
- Kishore, K.; Jha, A.; Samad, Y.; Kumar, M.; Shaharyar, R.L.; Jain, K.J. Design, Synthesis and Biological Evaluation of 1, 3, 4-oxadiazole Derivatives. Eur. J. Med. Chem. 2010, 45, 4963–4967.
- Musad, E.A.; Mohamed, R.; Ali Saeed, B.; Vishwanath, B.S.; LokanathaRai, K.M. Synthesis and Evaluation of Antioxidant and Antibacterial Activities of New Substituted Bis (1,3,4-oxadiazoles), 3,5-bis (substituted) Pyrazoles and Isoxazoles. Bioorg. Med. Chem. Lett. 2011, 21, 3536–3540.
- Rollas, S.; Gulerman, N.; Erdeniz II, H. Antimicrobial Activity and a SAR Study of Some Novel Benzimidazole Derivatives Bearing Hydrazone Moiety. Eur. J. Med. Chem. 2002, 57, 171–174.
- Sangshetti, J.N.; Chabukswar, A.R.; Shinde, D.B. Microwave Assisted One Pot Synthesis of Some Novel 2,5-disubstituted 1,3,4-oxadiazoles as Antifungal Agents. Bioorg. Med. Chem. Lett. 2011, 21, 444–448.
- Ramaprasad, G.C.; Kalluraya, B.; Sunil Kumar, B.; Hunnur, R.K. Synthesis, Characterization and Pharmacological Activities of 2-[4-cyano-(3-trifluoromethyl) phenyl amino)]-4-(4-quinoline/coumarin-4-yloxy)-6-(fluoropiperazinyl)-s-triazines. Eur. J. Med. Chem. 2010, 45, 4587–4593.
- Rashid, M.; Husain, A.; Mishra, R. Synthesis of Benzimidazoles Bearing Oxadiazole Nucleus as Anticancer Agents. Eur. J. Med. Chem. 2012, 54, 855–866.
- Pushpan, P.; Poojary, B.; Chikkanna, C.; Sunil Kumar, B. Oxadiazole a Nucleus with Versatile Biological Behavior. Eur. J. Med. Chem. 2012, 53, 203–210.
- Bondock, S.; Adel, S.; Etman, H.A.; Badria, F.A. Synthesis and Antitumor Evaluation of Some New 1,3,4-oxadiazole-based Heterocycles. Eur. J. Med. Chem. 2012, 48, 192–199.
- Macaaev, F.; Rusu, V.; Pogreboni, S.; Gudima, A.; Stingaci, E.; Vlad, I.; Shvets, N.; Kandemirli, F.; Dimogloa, A.; Reynolds, R. Synthesis of Novel 5-aryl-2-thio-1, 3, 4-oxadiazoles and the Study of Their Structure–anti-Mycobacterial Activities. Bioorg. Med. Chem. 2005, 13, 4842–4850.
- Sun, S.F.; Xiang, G.Y.; Hou, H.N.; Liu, Y. Studies on Interaction Between 4-(4-Hydroxybut-2-ynyloxy)-3-(phenylsulfonyl)-1,2,5-oxadiazole-2-oxide and Bovine Serum Albumin by Spectroscopic Method. Chin. J. Chem. 2006, 24, 1050–1053.
- Chun, Q.M.; Ke, A.L.; Wen, T.H.; Shen, Y.T. Fluorescence Enhancement of Oxadiazole by Albumin and the Microdetermination of Albumin by Fluorospectrophotometry. Microchem. J. 1998, 59, 417–426.
- Ellman, G.L.; Courtney, K.D.; Andres, V.; Featherstone, R.M. A New and Rapid Colorimetric Determination of Acetylcholinesterase Activity. Biochem. Pharm. 1961, 7, 88–95.
- Mahernia, S.; Bagherzadeh, K.; Mojab, F.; Amanlou, M. Urease Inhibitory Activities of some Commonly Consumed Herbal Medicines. Iran. J. Pharmaceuti. Res. 2015, 14, 943–947.
- Peters, T.J. All about Albumin: Biochemistry, Genetics, and Medical Applications; Academic Press, San Diego, CA, 1995.
- Valstar, A.; Almgren, M.; Brown, W. The Interaction of Bovine Serum Albumin with Surfactants Studied by Light Scattering. Langmuir 2000, 16, 922–927.
- Wang, H.; Jiang, X.; Zhou, L.; Cheng, Z.; Yin, W.; Duan, M.; Liu, P. Interaction of NAEn-s-n Gemini Surfactants with Bovine Serum Albumin: A Structure-activity Probe. J. Luminesc. 2013, 134, 138–147.
- Chmara, H.; Andruszkiewicz, R.; Borowski, E. Synthetic Derivatives of N3-fumaroyl-L-2,3-diaminopropanoic Acid Inactivate Glucosamine Synthesis from Candida albicans. Biochim. Biophys. Acta 1985, 870, 357–366.