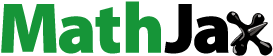
Abstract
The use of synthetic chemicals as herbicides for crop protection is a big threat due to toxicity, non-degradability, and negative impact on environment. Onosma bracteatum leaves and flowers, and Commiphora stocksiana Engl bark ethanolic extracts are evaluated for allelopathic potential against Brassica napus, a model plant. Complete allelopathic tendency was depicted by crude extract of O. bracteatum leaves and partial trend by flower and C. stocksiana extracts. B. napus seed germination efficiency and plant architecture is adversely influenced by the presence of plants extracts. The antioxidative analysis of Brassica plants depicts that extracts in the growth environment produces oxidative stress that eventually increased free radical scavenging activity, total antioxidative potential, and reducing power capability. Though Brassica plants produced phenolics and flavonoids to combat the oxidative stress but at insufficient concentration. Based on the findings, it can be concluded that the plants extracts produce oxidative stress to the seedlings and plants that eventually results in toxicity and allelopathic effect. Furthermore O. bracteatum can be a good candidate for natural herbicide either in form of extracts or the allelopathic compounds isolated from this plant species, which can be used as replacement of expensive and harmful synthetic herbicide.
Public Interest Statement
The use of herbicides in crop cultivation is cause of toxicology to environment and food chain. Plants or their extract may act as agent that inhibit germination of weeds or kill them. This manuscript describes allelopathic potential of two plant, which shows significant inhibition of target specie. Furthermore, how the plants extract inhibited seed germination or growth of target species is also proposed to evaluate the mechanism.
Competing Interests
The authors declare no competing interest.
1. Introduction
There is an alarming increase in weed distribution as they possess faster colonization and survive under adverse situations (Colautti, Grigorovich, & MacIsaac, Citation2006; Hamilton et al., Citation2005). They are also a major threat to plant species due to their abundance mainly caused by low regulation of competing plant species and natural predators (Callaway, DeLuca, & Belliveau, Citation1999). Consequently, in the areas under cultivation synthetic herbicides are used due to their affectivity at low concentration and cost (Dayan, Cantrell, & Duke, Citation2009). However, extensive use of synthetic herbicides leads to an increased risk of herbicide resistant weeds (Heap, Citation2014) and have toxic effect to other plants (Macías, Oliveros-Bastidas, & Marín, Citation2007). The indiscriminate use of synthetic herbicides is also hazardous to human and other biological communities (Peres & Moreira, Citation2007), environment (Akhtar, Sengupta, & Chowdhury, Citation2009) and even deplete nutrients from soil (Batish et al., Citation2007). Therefore an alternate source of natural herbicide apart from biodegradability, affectivity, low contamination (Souza Filho et al., Citation2006), and ecofriendly is a glittering option. Medicinal plants can be a promising tool to overcome the problems caused by synthetic herbicides (Gilani et al., Citation2010) as they possess versatile properties due to presence of bioactive compounds (Fujii, Parvez, Parvez, Ohmae, & Iida, Citation2003). In phytotoxicity, phytochemicals affect the growth, development, and functioning of the target specie (Mallik, Citation2008; Rice, Citation1984) as cover crop (Mallik, Citation2008) or the chemical compounds released (Macías, López, Varela, Torres, & Molinillo, Citation2008; Macías et al., Citation2001). Pelargonic acid (Copping & Duke, Citation2007), glufosinate (Duke, Dayan, Romagni, & Rimando, Citation2000), sorgoleone (Einhellig & Souza, Citation1992), and arteether (Bagchi, Jain, & Kumar, Citation1997) are few examples of herbicides isolated from plant species. The natural herbicides possess numerous advantages i.e. water solubility, less halogenated, ecofriendly, and novel mode of action (Duke et al., Citation2000; Macías et al., Citation2008). These compounds function by interrupting the basic procedures of respiration, enzyme activities, phytohormone level, water availability, mineral relation, permeability of cell wall, and cell division (Cruz-Ortega, Anaya, Hernández-Bautista, & Laguna-Hernández, Citation1998; Ferreira & Aquila, Citation2000). The phytotoxic ability of medicinal plant extract can be easily explored by analyzing the effect on seed germination, growth, and developmental parameters (Duke et al., Citation2000; Vyvyan, Citation2002).
The plants Onosma bracteatum Wall and Commiphora stocksiana Engl are traditionally well known in ayurvedic medicines for medicinal properties. O. bracteatum Wall locally known as Gaozaban is used as an antibacterial (Ahmad et al., Citation2009), anti-dandruff (Khan et al., Citation2013), and anti-inflammatory agent (Binzet & Akcin, Citation2012). It is also effective against respiratory (Kirtikar & Basu, Citation1999) and gastrointestinal problems (Ata, Faiza, & Shabnam, Citation2011). C. stocksiana Engl also known as Balsamodendron pubescens Stock and locally known by its gums Guggal famous for its medicinal properties that dates back to its ancient folkloric use. “Guggal” is related with the cure of laryngitis, rheumatism, inflammations, pneumonia, chronic dyspepsia, diarrhea, gum complaints, chronic endometritis, bronchitis, indolent ulcers, tonsillitis, leucorrhea piles, and whooping cough (Ishnava, & Mohan, Citation2008; Sharma & Kumar, Citation2012). The purpose of this study was to evaluate the allelopathic potential of O. bracteatum and C. stocksiana collected from natural habitat. The target plant species (Brassica) is also evaluated for antioxidative response and non-enzymatic antioxidative molecules to define possible mechanism of phytotoxicity by extracts.
2. Material and methods
2.1. Plant materials and extraction procedures
Fresh plant materials of O. bracteatum Wall was collected from Chaman and C. stocksiana Engl of Turbat region Baluchistan, Pakistan during July 2013 and were identified by Division of Plant Protection, Baluchistan Agricultural College, Quetta. The leaves and flowers of O. Bracteatum and the woody part of C. stocksiana were washed with tap water to remove dirt and debris. The plants material was shade dried at room temperature for two weeks and then dried in an incubator at 61°C.
2.2. Extraction procedure
Plant materials were cut into small pieces and pulverized in grinder and 100 g was dipped in ethanol (3:1). After three days, the extract was filtered using muslin cloth and Whatmann filter paper. The residue was dipped again in ethanol for additional three days and filtered thereafter. The filtrates were combined and concentrated by rotary evaporator (Rotovapor R 200 Buchi, Flawil Switzerland) at 40°C. The extracts were kept at 4°C until further analysis.
2.3. Phytotoxicity assay
The phytotoxic activity of the extracts is evaluated following the method of Attarad, Phull, Zia, Shah, and Haq (Citation2015), with minor modifications. Brassica napus, a dicotyledonous specie is used as a target specie due to its sensitivity to allelopathic extracts and growth pattern. The extracts were diluted at three concentrations i.e. 10,000, 1,000, and 100 ppm and poured in 28 mm Petri plates containing doubled layered Whatmann filter paper. The plates were placed in draft chamber till the solvent evaporated. Under aseptic conditions, B. napus seeds were sterilized by sodium hypochlorite solution for three min and rinsed with autoclaved distilled water. Twenty seeds were placed in each Petri plate. 2,4-D (2,4 Dichlorophenoxy acetic acid; an auxin homology well-known herbicide) was used as positive control while distilled water was used as negative control. The experiment was performed in triplicate. All the plates were rapped with parafilm to avoid the loss of moisture and kept in dark at room temperature i.e. 25 ± 1°C. Seed germination efficiency was monitored on daily basis until germination prevalence i.e. the emergence of radical by rupturing the seed coat. The germination indices to evaluate the phytotoxic potential are;
(1) | Final germination (FG) percentage is calculated as; | ||||
(2) | Rate of Germination (RG) = Σ Ni/Di | ||||
(3) | Mean period of final germination (MPFG) = Σ (NiDi)/S where N is the daily increase in the seedling number, D is the number of days from seed placement, and S is the total number of seeds germinated. | ||||
(4) | Germination index (GI) = (% Germination against sample)/(% Germination in the control) | ||||
(5) | Percentage inhibition (%) = 1 − [FG in extract (%)/FG in control (%)] × 100 |
Fifteen days old plantlets were examined for fresh and dry weight. The percentage seedling weight loss and relative dry weight percent is observed using following formula;
Germination percentage depicts the percent germination of a seeds after a specific period of time until it became constant as delayed germination cannot be explained using germination percentage. For this purpose RG and germination index is measured. Seedling weight loss (%Wt. loss) and relative dry weight percent (RDW %) are used to monitor the weight associated parameters.
2.4. Phytochemical and biochemical evaluation
The plantlets obtained after phytotoxic assay are analyzed for phytochemical and antioxidant potential to evaluate stress on germinated plantlets.
2.5. Sample preparation
The dried powdered plants were suspended in DMSO at 4 mg/ml. After 24 h the suspension was centrifuged at 4,000 rpm for 5 min and the supernatant is used for biochemical and antioxidative response. Total flavonoid content was determined by the aluminum chloride colorimetric method described by Almajano, Carbó, Jiménez, and Gordon (Citation2008). The absorbance was measured at 450 nm using an Agilent Spectrophotometer (DAD, 8453, Agilent Technologies, Waldbronn Analytical Division, Germany). The contents are determined as Quercetin equivalents (μg QE/mg D.W).
Total phenolics were determined according to the method of Astill, Birch, Dacombe, Humphrey, and Martin (Citation2001). The absorbance was measured at 630 nm. The total phenolic contents are determined as Gallic acid equivalents (μg GAE/mg D.W).
Antioxidant activities of the test samples is calculated using three different methods i.e. DDPH-based free radical scavenging activity, total antioxidant activity, and total reducing power.
The free radical quenching activity of the test samples against 2,2-diphenyl-1-picrylhydrazyl reagent (DPPH) is determined using procedure modified by Fatima et al. (Citation2015). The absorbance was measured at 515 nm. Percent inhibition is calculated for each sample using formula:
where Abs is the absorbance of DPPH solution with sample and Abc indicates the absorbance of negative control (containing the reagent except the sample).
Ascorbic acid is used as a standard and values above 50% are considered significant.
Total antioxidant activity of extracts is evaluated by method described by Clarke, Heather, Gregory, and Daniel (Citation2013). The absorbance was measured at 630 nm and Ascorbic acid is used as standard at final concentration of 50 μg/ml. The total antioxidant activity is determined as Ascorbic acid equivalents (μg AAE/mg D.W).
The reduction potential of the extracts is investigated according to the procedure described by Jafri, Saleem, Haq, Ullah, and Mirza (Citation2014). The absorbance was measured at 630 nm and Ascorbic acid is used as standard at final concentration of 100 μg/ml. The reducing power capacity is determined as Ascorbic acid equivalents (μg AAE/mg D.W).
2.6. Statistical analysis
All the experiments were conducted in triplicates. For allelopathy assay three Petri plates were seeded with 60 seeds (20 seeds in each) against each concentration. The selection of plants extract concentration for assay was completely randomized. The plant parameters were studied after 15 days of seed germination. Each plant was considered as unit. The data is presented as mean ± standard deviation. The means are further analyzed for least significant difference (LSD) at p ≤ 0.05 after analysis of variance.
3. Results
Phytotoxic (allelopathic) effect of crude extracts of C. stocksiana and O. bracteatum evaluated against seeds of B. napus depicts complete to partial allelopathic potential (Tables and ). Application of low concentration (100 ppm) of all extracts did not show any significant effect on B. napus seed germination. FG, RG, germination index, fresh weight, relative dry weight percent, and dry weight are found to decrease with increase in concentration of the extracts. While MPFG, percent inhibition and percent weight loss increase with increase in concentration of the extracts (Tables and ). The results are in correspondence to the positive control and constituents followed a similar pattern as that of 2,4-D, in fact few parameters (FG, MPFG, RG, GI, and RDW %) showed much better results than 2,4-D.
Table 1. Phytotoxic effect of crude extracts of O. bracteatum Wall and C. stocksiana Engl with germination associated parameters
Table 2. Phytotoxic effect of crude extracts of O. bracteatum Wall and C. stocksiana Engl with weight associated parameters
The phytochemical and biochemical evaluation of the Brassica plantlets is performed to observe the impact of stress on phytochemical profile. For 2,4-D at 10,000 ppm there were not sufficient plantlets, due to its potent allelopathic capacity, to conduct any of the assay as it completely prevented germination of the target plant species.
The presence of extracts in growth media caused oxidative stress on Brassica plants confirmed by variation in different activities. DPPH-based free radical scavenging activity decreased in plants due to the presence of 2,4-D in the environment. Likewise, extracts also induced oxidative stress at different concentrations making the free radical scavenging activity variable. The samples, however, show no significant DPPH scavenging activity (Figures and ). The free radical scavenging activity is linked with TAC and TRP. Variation in TAC and TRP in Brassica plants show that the metabolites in extracts make the plants under stress resulting in increase in these activities. However, the extent of stress makes the activities variable. The highest antioxidant capacity (88.5 μg AAE/mg D.W) is observed for bark extract of C. stocksiana at 1,000 ppm (Figure ) and the flower extract of O. bracteatum at 10,000 ppm i.e. 101.8 μg AAE/mg D.W (Figure ). Highest value of reducing power was observed among the leaf extract of O. bracteatum (148.2 μg AAE/mg D.W) at 1,000 ppm and for C. stocksiana (115.7 μg AAE/mg D.W) at 10,000 ppm of bark extracts (Figures and ). Although extracts constituents may stress the plants leading to death but the natural mechanism to combat the stress may assist the plant to survive. Enzymatic and non-enzymatic systems are present for this purpose and non-enzymatic system is more powerful because during stress the excessive reactive oxygen species (ROS) activates the natural defense mechanism. Secondary metabolites, meantime, produce to overcome the cells oxidative stress preventing alarming deadly accumulation of ROS. For this reason phenolics and flavonoids concentrations are analyzed but the varying trends of phenolics and flavonoids may be due to stress caused by the phytotoxic extracts that masked the production of these antioxidative components. The highest phenolic content are also observed in plantlets exposed to bark extracts of C. stocksiana at 100 ppm i.e. 55.1 μg GAE/mg D.W (Figure ) while highest flavonoid content (22.4 μg QE/mg D.W) was observed at 10,000 ppm. In case of O. bracteatum, highest phenolic content was observed by the ones exposed to leaf extracts at 1,000 ppm i.e. 52.4 μg GAE/mg D.W (Figure ) and highest flavonoid content (24.8 μg QE/mg D.W) was observed in flower extracts of O. bracteatum at 1,000 ppm. The results depict oxidative stress in the model plants that was higher as compared to positive and negative control with few exceptions. The phenolic and flavonoid content are affected by the oxidative stress caused by the extracts as lower values of phenolic content and higher values of flavonoid content as compared to 2,4-D suggest the unusual inhibition and enhancement of these natural antioxidative molecules.
Figure 1. DPPH radical scavenging activity (% inhibition), total antioxidant capacity (TAC μg AAE/mg D.W), and reducing power (TRP μg AAE/mg D.W) of allelopathic plantlets exposed to C. stocksiana Engl bark at varying concentration along with 2,4-D and dH2O.
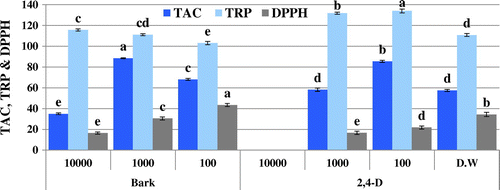
Figure 2. DPPH radical scavenging activity (% inhibition), total antioxidant capacity (TAC μg AAE/mg D.W), and reducing power (TRP μg AAE/mg D.W) of allelopathic plantlets exposed to O. bracteatum Wall leaves and flowers at varying concentration along with 2,4-D and dH2O.
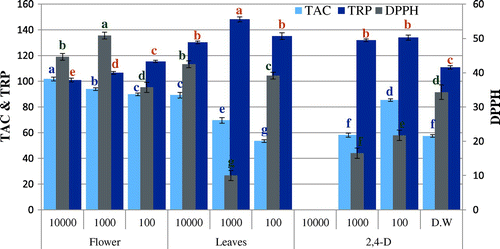
Figure 3. Total phenolic content (TPC μg GAE/mg D.W) and total flavonoid content (TFC μg QE/mg D.W) of allelopathic plantlets exposed to C. stocksiana Engl bark extract at varying concentration along with 2,4-D and dH2O.
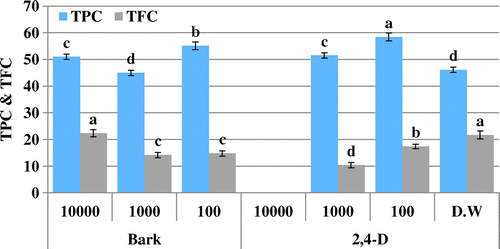
Figure 4. Total phenolic content (TPC μg GAE/mg D.W) and total flavonoid content (TFC μg QE/mg D.W) of allelopathic plantlets exposed to O. bracteatum Wall leaves and flowers extracts at varying concentration along with 2,4-D and dH2O.
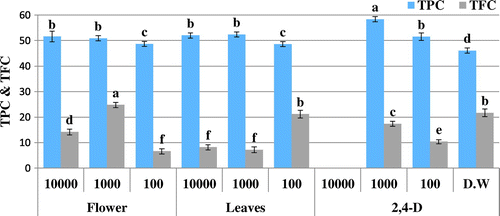
4. Discussion
Phytomedicines are well known for numerous advantages and are considered highly valuable in terms of affectivity against different diseases (Jiang et al., Citation2006). Along with these characteristics, they also tend to be effective allelopathic agents. The current study proposed a complete phytotoxic tendency of leaves extract of O. bracteatum and partial allelopathic trend of C. stocksiana. The seed germination inhibition potential might be associated with the presence of allelochemicals that affect germination and growth of plants (Evenari, Citation1949; Gressel & Holm, Citation1964). Khanh, Chung, Xuan, and Tawata (Citation2005) reported that maximum allelopathic suppression may occur when allelochemicals interfere with early growth stages of plant. Although details of the biochemical mechanism through which allelochemicals exert a toxic effect on germination and growth of the respective plants is still unknown (Zhou & Yu, Citation2006) but dos Santos et al. (Citation2008) suggested that allelochemicals might affect enzymatic activity or cause depolarization of the root cell membrane or lipid peroxidation blocking. The extracts affected germination and weight associated parameters in a concentration dependent manner similar to one observed by Inderjit and Keating (Citation1999). FG, RG, relative dry weight percent, germination index, fresh weight, and dry weight decreased with increase in concentration of the sample. The variations if fresh weight and dry weight depict that the plants extracts varied water holding capacity of Brassica plantlets. Furthermore it indicates that presence of different concentrations of extracts stress the plants to produce biomass. Previous studies also suggest that the effect of phytotoxins on the plants is species dependent both in biochemical and physiological characteristics (Kobayashi, Citation2004; Prati & Bossdorf, Citation2004; Xuan, Shinkichi, Khanh, & Chung, Citation2005). Germination inhibition due to allelochemicals is known to be associated to lipid mobilization and low levels of isothiocynates (Baleroni, Ferrarese, Souza, & Ferrarese-Filho, Citation2000; Petersen, Belz, Walker, & Hurle, Citation2001). The identification of compounds responsible for causing allelopathy is of great interest as it can be used as an effective alternative method for weed control. Allelochemicals mainly function by affecting cell division and elongation or by suppressing enzymes responsible for the movement of nutrients, important for germination (Batlang & Shushu, Citation2007). The current focus of agriculture industry is to find a safe and biological solution to reduce foreseen hazardous impacts of herbicides and insecticides (Khanh & Chung, Citation2004).
The significant increase in phenolic and flavonoid contents shows varying trend that might be linked to the mediocre point where the effect of phytotoxins get masked. The high phytochemical content can be associated to the stress and to the allelopathic treatment as previous studies depict a strong relation of phenolics and flavonoids with the allelopathic potential (Macías et al., Citation2008). The antioxidative evaluation of the plantlets shows that stress increases production of secondary metabolites suggesting that not only medicinal plants can be used as an herbicide but it might also help in the enhancement of plants antioxidative potential. Along with that the phytochemical and antioxidant assays help to identify the nature of allelochemicals involved in causing a specific effect. These effects may be associated to the phenolic, saponins, tannins, alkaloids, or terpenoids present in the extract being used (Einhellig, Citation1995; Inderjit & Nilsen, Citation2003) and later help out in isolation of compounds that can be used as potent herbicide with lesser side effects and more effective mode of action in comparison to synthetic ones.
5. Conclusion
The allelopathic tendency depicted by the crude extract of two plants and their effect on different parameters suggests the isolation of an important compound that can act as potent herbicide for future with lesser side effects to the environment. The phytochemical and antioxidant evaluation of the plantlets also depict that the extract cause oxidative stress leading to cell/plant death. Furthermore, the ROS and oxidative stress are one of the biochemical concerns that govern allelopathic effect.
Additional information
Funding
Notes on contributors
Joham Sarfraz Ali
Muhammad Zia and his group is working on different aspects of plant biotechnology. They are interested in phytochemistry of plants and their possible use/role in different aspects of life including agriculture, food, medicine, and environment.
References
- Ahmad, B., Ali, N., Bashir, S., Choudhary, M. I., Azam, S., & Khan, I. (2009). Parasiticidal, antifungal and antibacterial activities of Onsomagriffithi. African Journal of Botany, 8, 5084–5087.
- Akhtar, W., Sengupta, D., & Chowdhury, A. (2009). Impact of pesticides use in agriculture: Their benefits and hazards. Interdisciplinary Toxicology, 2(1), 1–12.10.2478/v10102-009-0001-7
- Almajano, M. P., Carbó, R., Jiménez, J., & Gordon, M. H. (2008). Antioxidant and antimicrobial activities of tea infusions. Food Chemistry, 108, 55–63.10.1016/j.foodchem.2007.10.040
- Astill, C., Birch, M. R., Dacombe, C., Humphrey, P. G., & Martin, P. T. (2001). Factors affecting the caffeine and polyphenol contents of black and green tea infusions. Journal of Agricultural Food Chemistry, 49, 5340–5347.10.1021/jf010759+
- Ata, S., Faiza, F., & Shabnam, J. (2011). Elemental profile of 24 common medicinal plants of Pakistan and its direct link with traditional uses. Journal of Medicinal Plant Research, 5, 6164–6168.
- Attarad, A., Phull, A. R., Zia, M., Shah, A. M. A., & Haq, I. U. (2015). Phytotoxicity of River Chenab sediments: In vitro morphological and biochemical response of Brassica napus L. Environmental Nanotechnology, Monitoring & Management, 4, 74–84.
- Bagchi, G. D., Jain, D. C., & Kumar, S. (1997). Arteether: A potent plant growth inhibitor from Artemisia annua. Phytochemistry, 45, 1131–1133.10.1016/S0031-9422(97)00126-X
- Baleroni, C. R. S., Ferrarese, M. L. L., Souza, N. E., & Ferrarese-Filho, O. (2000). Lipid accumulation during canola seed germination in response to cinnamic acid derivatives. Biologia Plantarum, 43, 313–316.10.1023/A:1002789218415
- Batish, D., Singh, H., Kohli, R., Kaur, S., Saxena, D., & Yadav, S. (2007). Assessment of parthenin against some weeds. ZeitschriftfürNaturforschung, 62, 367–372.
- Batlang, U., & Shushu, D. D. (2007). Allelopathic activity of sunflower (Helianthus annuus L.) on growth and nodulation of bambara groundnut (Vigna subterranean (L.) Verdc.). Journal of Agronomy, 6, 541–547.
- Binzet, R., & Akcin, O. E. (2012). The anatomical properties of two Onosma L. (Boraginaceae) species from Turkey. Journal of Medicinal Plant Research, 6, 3288–3294.
- Callaway, R. M., DeLuca, T. H., & Belliveau, W. M. (1999). Biological-control herbivores may increase competitive ability of the noxious weed Centaurea maculosa. Ecology, 80, 1196–1201.10.1890/0012-9658(1999)080[1196:BCHMIC]2.0.CO;2
- Clarke, D., Heather, W., Gregory, S., & Daniel, R. (2013). Detection and learning of floral electric fields by bumblebees. Journal of Inorganic Biochemistry, 53, 573–583.
- Colautti, R. I., Grigorovich, I. A., & MacIsaac, H. J. (2006). Propagule pressure: A null model for biological invasions. Biological Invasions, 8, 1023–1037.10.1007/s10530-005-3735-y
- Copping, L. G., & Duke, S. O. (2007). Natural products that have been used commercially as crop protection agents. Pest Management Science, 63, 524–554.10.1002/(ISSN)1526-4998
- Cruz-Ortega, R., Anaya, A. L., Hernández-Bautista, B. E., & Laguna-Hernández, G. (1998). Effescts of allelochemical stress produced by Sicyos deppei on seedling root ultraestrucutre of Phaseolus vulgaris and curcubita ficifolia. Journal of Chemical Ecology, 24, 2039–2057.10.1023/A:1020733625727
- Dayan, F. E., Cantrell, C. L., & Duke, S. O. (2009). Natural products in crop protection. Bioorganic and Medicinal Chemistry, 17, 4022–4034.10.1016/j.bmc.2009.01.046
- dos Santos, W. D., Ferrarese, M. L., Nakamura, C. V., Mourão, K. S., Mangolin, C. A., & Ferrarese-Filho, O. (2008). Soybean (Glycine max) root lignification induced by ferulic acid. The possible mode of action. Journal of Chemical Ecology, 34, 1230–1241.10.1007/s10886-008-9522-3
- Duke, S. O., Dayan, F. E., Romagni, J. G., & Rimando, A. M. (2000). Natural products as sources of herbicides: Current status and future trends. Weed Research, 40, 99–111.10.1046/j.1365-3180.2000.00161.x
- Einhellig, F. A. (1995). Allelopathy: Organisms, processes and applications. In Inderjit, K. M. M. Dakshini, F. A. Einhellig, (Eds). Journal of American Chemical Society (Vol. 582, pp. 96–116). Washington, DC: American Chemical Society.
- Einhellig, F. A., & Souza, I. F. (1992). Phytotoxicity of sorgoleone found in grain Sorghum root exudates. Journal of Chemical Ecology, 18, 1–11.10.1007/BF00997160
- Evenari, M. (1949). Germination inhibitors. The Botanical Review, 15, 153–194.10.1007/BF02861721
- Fatima, H., Khan, K., Zia, M., Ur-Rehman, T., Mirza, B., & Haq, I.-U. (2015). Extraction optimization of medicinally important metabolites from Daturainnoxia Mill: An in vitro biological and phytochemical investigation. BMC Complementary and Alternative Medicine, 15, 1.
- Ferreira, A. G., & Aquila, M. E. A. (2000). Alelopatia: Umaáreaemergente de ecofisiologia [Alellopathy: An emerging topic in ecophysiology]. RevistaBrasileira de Fisiologia Vegetal/Brazilian Journal of Plant Physiology, 12, 175–204.
- Fujii, Y., Parvez, S. S., Parvez, M. M., Ohmae, Y., & Iida, O. (2003). Screening of 239 medicinal plant species for allelopathic activity using the sandwich method. Weed Biology and Management, 3, 233–241.10.1046/j.1444-6162.2003.00111.x
- Gilani, S. A., Fujii, Y., Shinwari, Z. K., Adnan, M., Kikuchi, A., & Watanabe, K. N. (2010). Phytotoxic studies of medicinal plant species of Pakistan. Pakistan Journal of Botany, 42, 987–996.
- Gressel, J. B., & Holm, L. G. (1964). Chemical inhibition of crop germination by weed seeds and the nature of inhibition by Abutilon theophrasti. Weed Research, 4, 44–53.10.1111/wre.1964.4.issue-1
- Hamilton, M. A., Murray, B. R., Cadotte, M. W., Hose, G. C., Baker, A. C., Harris, C. J., & Licari, D. (2005). Life-history correlates of plant invasiveness at regional and continental scales. Ecology Letters, 8, 1066–1074.10.1111/j.1461-0248.2005.00809.x
- Heap, I. (2014). The international survey of herbicide resistant weeds. Retrieved fromhttp://www.weedscience.org
- Inderjit, & Keating, K. I. (1999). Allelopathy: Principles, procedures, processes, and promises for biological control. Advances in Agronomy, 67, 141–231.10.1016/S0065-2113(08)60515-5
- Inderjit, & Nilsen, E. (2003). Bioassays and field studies for allelopathy in terrestrial plants: Progress and problems. Critical Reviews in Plant Sciences, 22, 221–238.10.1080/713610857
- Jafri, L., Saleem, S., Haq, I.U., Ullah, N., & Mirza, B. (2014). In vitro assessment of antioxidant potential and determination of polyphenolic compounds of Hedera nepalensis K. Koch. Arab Journal of Chemistry. doi:10.1016/j.arabjc.2014.05.002
- Jiang, C., Chang, M., Wen, C., Lin, Y., Hsu, F., & Lee, M. (2006). Natural products of cosmetics: Analysis of extracts of plants endemic to Taiwan for the presence of tyrosinese inhibitory, melanin reducing and free radical scavenging activities. Journal of Food and Drug Analysis, 14, 346–352.
- Ishnava, K. B., & Mohan, J. S. S. (2008). Intraspecific isozymes variation in Commiphora wightii (Arn.) Bhandari: A traditional hypocholesteremic medicinal shrub from Gujarat, India. Journal of Herbs, Spices and Medicinal Plants, 13, 25–40.
- Khan, S. M., Page, S., Ahmad, H., Shaheen, H., Ullah, Zahid, Ahmad, M., & Harper, D. M. (2013). Medicinal flora and ethnoecological knowledge in the Naran Valley, Western Himalaya, Pakistan. Journal of Ethnobiology and Ethnomedicine, 9, 4.10.1186/1746-4269-9-4
- Khanh, T., & Chung, I. (2004). Weed-suppressing potential of dodder (Cuscuta hygrophilae) and its phytotoxic constituents. Weed Sciences, 56, 119–127.
- Khanh, T. D., Chung, M. I., Xuan, T. D., & Tawata, S. (2005). The exploitation of crop allelopathy in sustainable agricultural production. Journal of Agronomy and Crop Science, 191, 172–184.10.1111/j.1439-037X.2005.00172.x
- Kirtikar, K. R., & Basu, B. D. (1999). Indian medicinal plants (Vol. 3, 2nd ed., p. 1699). New Delhi: International Book Distributors.
- Kobayashi, K. (2004). Factors affecting phytotoxic activity of allelochemicals in soil. Weed Biology Management, 4, 1–7.10.1111/wbm.2004.4.issue-1
- Macías, F. A., López, A., Varela, R. M., Torres, A., & Molinillo, J. M. G. (2008). Helikauranoside A, a new bioactive diterpene. Journal of Chemical Ecology, 34, 65–69.10.1007/s10886-007-9400-4
- Macías, F. A., Molinillo, J. M. G., Galindo, J. C. G., Varela, R. M., Simonet, A. M., & Castellano, D. (2001). The use of allelopathic studies in the search for natural herbicides. Journal of Crop Production, 4, 237–255.10.1300/J144v04n02_08
- Macías, F. A., Oliveros-Bastidas, A., & Marín, D. (2007). Plant biocommunicators: Their phytotoxicity, degradation studies and potential use as herbicide models. Phytochemistry Reviews, 7, 179–194.10.1007/s11101-007-9062-4
- Mallik, A. U. (2008). Allelopathy: Advances, challenges and opportunities. In R. S. Zeng, A. U. Mallik, & S. M. Luo (Eds.), Allelopathy in Sustainable Agriculture and Forestry (pp. 25–38). New York, NY: Springer Science, Business Midia.10.1007/978-0-387-77337-7
- Peres, F., & Moreira, J. C. (2007). Saúde e ambiente e sua relação com o consumo de agrotóxicos em um pólo agrícola do estado do Rio de Janeiro, Brasil [Health and environment and its relation with the consumption of agrochemicals in an agricultural center in the state of Rio de Janeiro, Brazil]. Rio deJaneiro: Caderno de Saúde Pública.
- Petersen, J., Belz, R., Walker, F., & Hurle, K. (2001). Weed suppression by release of isothiocyanates from turnip-rape mulch. Agronomy Journal, 93, 37–43.10.2134/agronj2001.93137x
- Prati, D., & Bossdorf, O. (2004). Allelopathic inhibition of germination by Alliaria petiolata (Brassicaceae). American Journal of Botany, 91, 285–288.10.3732/ajb.91.2.285
- Rice, E. L. (1984). Allelopathy. Orlando, FL: Academic Press.
- Sharma, S., & Kumar, A. (2012). Traditional uses of herbal medicinal plants of Rajashthan. International Journal of Life Science Pharma Research, 2, 77–82.
- Souza Filho, A. P. S., Santos, R. A., Santos, L. S., Guilhon, G. M. P., Santos, A. S., Arruda, M. S. P., … Arruda, A. C. (2006). Potencial alelopático de Myrcia guianensis. Planta Daninha, 24, 649–656.10.1590/S0100-83582006000400005
- Vyvyan, J. R. (2002). Allelochemicals as leads for new herbicides and agrochemicals. Tetrahedron, 58, 1631–1646.10.1016/S0040-4020(02)00052-2
- Xuan, T. D., Shinkichi, T., Khanh, T. D., & Chung, I. M. (2005). Biological control of weeds and plant pathogens in paddy rice by exploiting plant allelopathy: an overview. Crop Protection, 24, 197–206.10.1016/j.cropro.2004.08.004
- Zhou, Y. H., & Yu, J. Q. (2006). Allelochemicals and photosynthesis. In M. J. Reigosa, N. Pedrol, & L. Gonzalez (Eds.), Allelopathy: A Physiological Process with Ecological Implications (pp. 127–139). Dordrecht: Springer.