Abstract
Background: Methicillin resistant Staphylococcus aureus (MRSA) is an important Gram positive pathogen that has raised concerns due to its increasing prevalence despite pharmaceutical and technological advances. It does not only cause infections in humans but it can also be zoonotic in nature. The aim of this study is to investigate the prevalence of MRSA from presumably healthy shelter animals, in particular, canines and felines. Methods: Fifty-two faecal samples from canines and felines were collected from an animal shelter for the isolation of MRSA. This was carried out using the ChromMRSA (Oxoid, United Kingdom) media, followed by antibiotic susceptibility testing using the Kirby–Bauer disk diffusion test in accordance to the Clinical and Laboratory Standards Institute (CLSI) for ceftazidime, enrofloxacin, methicillin, oxacillin and vancomycin. Principal component analysis (PCA) was then employed to identify the variables in antibiotic sensitivity and emphasise any patterns between isolates and the antibiotic profile from both animal samples types. This was then summarised using descriptive statistics. Results: 283 and 169 S.aureus isolates were obtained respectively from the canine and feline samples on selective media. Of this, 33/283 (11.66%) and 13/169 (7.70%) were MRSA when grown on ChromMRSA. Canine MRSA isolates exhibited resistance in decreasing order of methicillin (100%), ceftazidime (81.82%), enrofloxacin (78.79%), oxacillin (60.61%) and vancomycin (0%). On the same note feline MRSA isolates indicated resistance to methicillin (100%), ceftazidime (100%), enrofloxacin (92.31%), oxacillin (84.62%) and vancomycin (0%). 51.51% of the canine and 84.62% of feline MRSA isolates indicated resistance to four out of five antibiotics tested. PCA of antibiotic resistance profiles revealed that canine and feline formed distinct groups. However, one of the feline MRSA isolates resembled more of the canine group; although likelihood of cross-transmission between the animals may be low due to separate enclosures for the canines and felines: cross-transmission may have occurred when animals are brought into the main building for vaccination, neutering and consulting procedures. Conclusion: The majority of MRSA isolates obtained were not only resistant to methicillin alone but to other antibiotics too. Vancomycin proved to be the only effective antibiotic. This will pose a greater risk of resistance developing in empirical antibiotics in the future if proper antibiotic stewardship practices are not in place.
Public Interest Statement
This research looks into the prevalence of MRSA, which is a type of bacteria that is resistant to many commonly used antibiotics. The research involves taking stool samples and isolates MRSA from these samples. The MRSA isolates are then further analysed to differentiate the bacterial strains. The zoonotic nature of MRSA is a matter of high concern due to which it can spread from animals to humans and animal shelters act as a potential hub where this contact can take place. We hope that this research can provide a public health awareness about the importance of biosecurity and sewage or waste management from animal shelters.
Competing Interests
The authors declare no competing interest.
1. Introduction
The discovery of antibiotics in 1928 by Sir Alexander Fleming revolutionised the treatment of infectious diseases and the quality of life of many patients, such as those suffering from Tuberculosis, Lyme disease, etc. Penicillin for instance, saved approximately 12–15% of the lives of Allied soldiers during the second World War and reduced the numbers of amputations as it prevented septicaemia from bacterial infections (Aldridge, Parascandola, & Sturchio, Citation1999). In tandem with this development, however, is the emergence of antibiotic-resistant bacteria. Healthcare-related problems such as failure to respond to treatment, increase in morbidity and mortality, increase in incidence of complications and increased treatment costs due to lengthened hospital stay and higher use of novel antibacterial drugs are repercussions of this resistance phenomenon (Livermore, Citation2003). This phenomenon, however in part, is caused by the inappropriate and rampant use of antibiotics by medical practitioners, both in the clinical and agricultural settings.
Overprescription of antibiotics exerts selective pressure on bacteria and those that can resist the harmful effects will be favoured for survival, culminating to the emergence of the fittest strain. The most common multidrug resistant bacteria includes methicillin resistant Staphylococcus aureus (MRSA); they are ubiquitous, often present asymptomatically in domestic animals such as canines and felines despite being known as a nosocomial pathogen for the past 30 years (Gardam, Citation2000). Recent studies show that MRSA development in companion animals is increasing (Loeffler et al., Citation2005; Strommenger et al., Citation2006) which can be a public health concern especially in situation where owners are immunocompromised.
The mechanism of resistance of MRSA is associated with the methicillin resistance gene (mecA) which codes for a protein that resists binding by methicillin, preventing the drug from exerting its effects. This gene is carried on the mobile genetic element known as staphylococcal chromosomal cassette mec (SCCmec) enabling them to become resistant to all β-lactam antibiotics by coding for the penicillin binding protein PBP2a (Hiramatsu, Cui, Kuroda, & Ito, Citation2001; Hiramatsu, Katayama, Yuzawa, & Ito, Citation2002). MRSA is involved in the development of clinical syndromes such as bacteraemia, pneumonia, cellulitis, osteomyelitis, endocarditis and septic shock (Klevens et al., Citation2007).
Several research papers have shown that canine faeces contain gastrointestinal tract pathogens that can cause diarrhoea in humans such as Campylobacter, Salmonella, Yersinia and E. coli (Beutin, Citation1999; Chaban, Ngeleka, & Hill, Citation2010; Lefebvre, Reid-Smith, Boerlin, & Weese, Citation2008). Moreover, the faeces may facilitate the diffusion of protozoa including Giarda and Crytosporidium and roundworms such as Toxocara canis (El-Tras, Holt, & Tayel, Citation2011). Furthermore, there is evidence to suggest that the stool samples of pets may have the potential to harbour antibiotic-resistant bacteria such as MRSA (Rich & Roberts, Citation2004). In addition, MRSA strains found in canine faeces and infected pets have been shown to be similar to those isolated from the faecal samples of human subjects with nosocomial infections in hospital settings (Cefai, Ashurst, & Owens, Citation1994). This could be a result of the direct transfer of bacteria between these two hosts, further amplifying the development of antibiotic resistance in the host gut that facilitates the hidden selection of resistant bacteria, manifesting in critical illnesses and nosocomial infections caused by resistant bacteria. Furthermore, cross contamination as a direct cause of this transfer is becoming increasingly rampant, adding to the complexity of the problem (Carlet, Citation2012).
Human–animal relationships shared a long history from the time when humans first used animals such as buffaloes in the agricultural sector in rural settings to modern day context wherein surveillance dogs assist the police to sniff out illegal substances and explosives, etc. Other examples of human–animal interaction in non-clinical settings can further be expanded to pet shop owners, veterinarians and animal shelter workers. Cases of animal-to-human transmissions of MRSA were reported and MRSA is gradually emerging as an important veterinary pathogen (Weese et al., Citation2006). The focus of this work is primarily on the prevalence of MRSA from shelter animals in nonclinical settings.
2. Methods
2.1. Sample origin and collection
The study was approved by the Institutional Review Board at Perdana University (PUIRB0055). A total of 52 faecal samples obtained from 28 canines and 24 felines of different breeds were collected from a local animal shelter in the Klang Valley, Malaysia during the months of May and June 2014 based on an inclusion criteria such as healthy, vaccinated and neutered animals that were ready for adoption. The samples were placed in faecal containers labelled with details of the animal, before being stored at −20 °C in the freezer at the Virology Laboratory of the Institute of Bioscience, Universiti Putra Malaysia (UPM) until further use.
2.2. Isolation and enumeration of bacteria
0.1 g of each faecal sample was weighed and placed into Eppendorf tubes followed by addition of 900 μL of 0.9% sodium chloride, NaCl (Kollin, USA). The mixture was homogenised briefly using a vortex mixer. Ten-fold serial dilutions were carried out up to dilution of 10−9. Then 100 μL of each dilution was spread onto Tryptone Soya Agar, TSA (Oxoid, United Kingdom) in triplicates. This was done within 30 min of the preparation of the dilutions. The plates were incubated for 48 h at 37°C. Countable number of colonies between 30 and 300 were proceeded to replica plating onto Mannitol Salt Agar, (Oxoid, United Kingdom) for selection of S. aureus. To isolate MRSA another set of replica plating was performed onto ChromMRSA, (Chromagar, Oxoid, United Kingdom). The number of isolates on Mannitol Salt Agar and ChromMRSA were enumerated after incubation at 37°C for 24 h. S. aureus ATCC 25,923 was used as a control.
2.3. Disk diffusion testing
Positively tested MRSA isolates from ChromMRSA were then tested for antibiotic resistance using a range of antibiotic classes which included penicillins, cephalosporins, glycopeptides and fluoroquinolones namely methicillin, oxacillin, ceftazidime, vancomycin and enrofloxacin. All antibiotics are soluble in water. Volume of 20 μL of varying amounts of antibiotics as shown in Table were impregnated into the 6 mm disks (Oxoid, United Kingdom). The size classification of average inhibition zones (Cockerill et al., Citation2012) is also given. Antibiotic susceptibility was carried out using Mueller-Hinton Agar which had been spread with putative MRSA isolates adjusted to 0.5 MacFarland (1.5 × 108 CFU/ML) prior to placement of the disks. The inhibition zones for each antibiotic were measured after 24 h of incubation at 37°C. Distilled water was used as a negative control and the experiment was carried out in triplicates.
Table 1. Antibiotics, solvent and amount impregnated per disk
2.4. Statistical analysis
Descriptive statistics that are used to summarise the raw data were done using Microsoft Excel and R (Team R.C, Citation2012). Principal component analysis (PCA) was done using the prcomp() function of the R stats package and visualization of the plot were calculated using the ggbiplot package.
3. Results
3.1. ChromMRSA and antibiotic susceptibility results
Not all collected faecal samples yield S. aureus. In the search of S. aureus, 283 isolates in canines and 169 isolates in felines were obtained. 33 out of 283 (11.66%) isolate for canines and 13 out of 169 (7.70%) isolate for felines were confirmed to be MRSA. These 46 isolates were then subjected to antibiotic susceptibility testing using disk diffusion and the results are listed in Tables and .
Table 2. The resistance classification for 33 MRSA isolated from canines
Table 3. The resistance classification for 13 MRSA isolated from felines
Feline resistance to ceftazidime, enrofloxacin and oxacillin is higher compared to canine resistance. Canine MRSA isolates exhibited resistance in decreasing order of methicillin (100%), ceftazidime (81.82%), enrofloxacin (78.79%), oxacillin (60.61%) and vancomycin (0%) (Figure ). Feline MRSA isolates’ resistance are as follows, methicillin (100%), ceftazidime (100%), enrofloxacin (92.31%), oxacillin (84.62%) and vancomycin (0%) (Figure ). 51.51% of the canine and 84.62% of feline MRSA isolates indicated resistance to four out of five antibiotics tested (Figure ).
Figure 1. Antibiotic resistance profile for 33 canine (A) and 13 feline (B) MRSA isolates based on level of resistance towards different antibiotics in percentage.
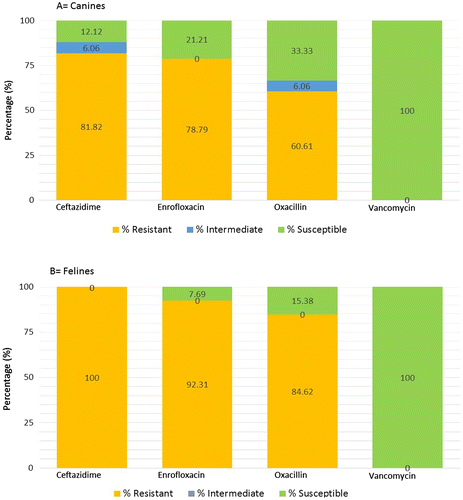
The distribution of the raw data of antibiotic profiling of each MRSA isolate was summarised in boxplots comparing canine and feline groups (Figure ). From the boxplots, clear differences in the distribution of MRSA isolate of canine vs. feline could be observed and there was more variation amongst canine isolates when compared to feline. From the PCA analysis, the cumulative proportion of variance explained by the first two components was 83%. There was a clear demarcation between the antibiotic profile of the canine and feline isolates (Figure ). Interestingly, one of the feline isolates indicated an antibiotic profile that was more similar to the canine group.
Figure 3. Boxplots of antibiotics resistance profiles, which are measured by inhibition zone (mm), of canine and feline isolates.
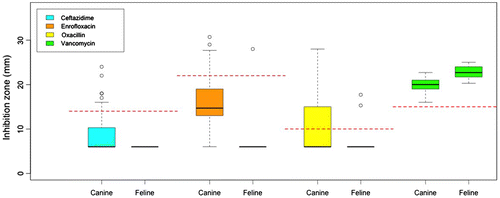
Figure 4. Principal components analysis plot of canine and feline isolates as groups and the four antibiotics, ceftazidime, enrofloxacin, oxacillin and vancomycin, as variables.
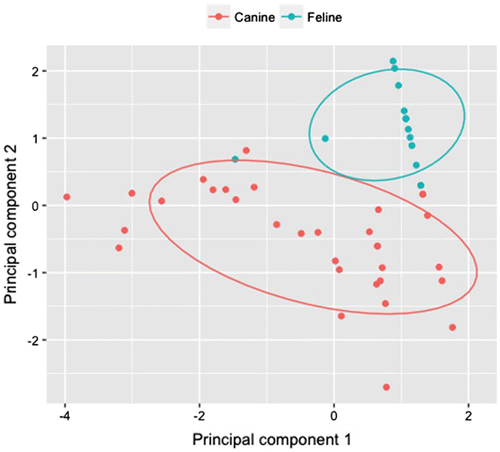
4. Discussion
4.1. Handling of shelter animals infected with MRSA
An animal shelter is potentially a major reservoir of MRSA as there is an influx and efflux of large number of animals. The nature of animal shelters that keep large number of animals in a confined space increases the possibility of bacterial transmission. Furthermore, in Malaysia, many animal shelters double up their holding area for excess of stray animals. Generally, these stray animals are immunocompromised and needed to be kept for a limited time before they can be humanely euthanised. This type of management enhances the spreading of pathogens such as MRSA in the healthy animals too. Proper preventive care remains a challenge not only to the resident animals but also to the people working and visiting there. In addition to excretions, bacteria festering in the anterior nares or open wounds of animals can cause boils, impetigo and rashes.
The control and prevention of infectious animals being taken into the shelters is difficult to mitigate. Although many shelters have stringent adoption policies, infectious animals which are in their clinically latent incubation phase may not be identified and housed anyway. MRSA is a commonly found microbe in shelter animals and is becoming more resistant towards available antibiotics (Tacconelli, De Angelis, Cataldo, Pozzi, & Cauda, Citation2008). Overcrowding and lack of isolation units in shelters cause MRSA to spread to previously uninfected animals thus becoming an important source of community acquired MRSA (CA-MRSA) today (Steneroden, Hill, & Salman, Citation2011).
4.2. Use of antibiotics to counter MRSA
In our study, 11.66% isolates for canines and 7.70% for felines were identified to be MRSA. Methicillin was initially used to treat penicillin-resistant S. aureus and by 1961, there were already reports of the emergence of methicillin resistant strains (Enright et al., Citation2002). MRSA is an emerging medical and veterinary problem as it can cause complications in immunocompromised shelter animals such as surgical site infection, blood stream infection and pneumonia (Oztoprak et al., Citation2006). In addition, compared to S. aureus, MRSA is not only more difficult to eradicate, but also it is associated with higher mortality due to the higher increased virulence of MRSA, the reduced effectiveness of vancomycin and the delay in administering appropriate antimicrobial therapy (Cosgrove et al., Citation2003).
Ceftazidime is more effective against MRSA isolates in felines than canines. Ceftazidime is a third generation cephalosporin and generally used for Gram-negative microbes (Tambekar, Dhanorkar, Gulhane, & Dudhane, Citation2007). β-lactamase enzyme can degrade Ceftazidime, hence MRSA which express this enzyme are resistant to Ceftazidime. It explains the relatively high rates of resistance found in the tested canines and felines. Overall, feline resistance to ceftazidime, enrofloxacin and oxacillin is higher compared to canine resistance. The higher resistance for enrofloxacin in felines may be due to mutation in the DNA gyrase (Lysnyansky et al., Citation2013). Enrofloxacin, a synthetic antibacterial from the fluoroquinolone class works by preventing the synthesis and supercoiling of the bacteria DNA. This is done by inhibiting DNA gyrase, a type 2 topoisomerase (Ramli, Neoh, Aziz, & Hussin, Citation2012). Vancomycin is commonly used clinically in MRSA infections by inhibiting the synthesis of peptidoglycan, a major component in the bacterial cell wall by forming complexes with D-Ala-D-ala in various phases of cell wall synthesis (Perkins & Nieto, Citation1973, Citation1974). There were no MRSA isolates in this study that were resistant or intermediately resistant to vancomycin. This confirms that there are no vancomycin resistant or intermediate S. aureus yet detected in animals in Malaysia (Ng et al., Citation2011), although they have been reported in many countries worldwide (Tiwari & Sen, Citation2006).
4.3. Preventive measures against MRSA
It was also noted that most of the isolates were resistant to four of the five antibiotics tested. It is widely accepted that MRSA can colonise healthy animals but do not cause any harm or disease. However, when an animal is immunocompromised or if there is an underlying pathological condition, the organism can cause persistent infections. It is important to note that MRSA cannot be eradicated and thus proper personal hygiene practice remains fundamental. Proper containment measures such as stricter antibiotic policies should be adopted to minimise the spread and development of these resistant strains. Proper aseptic and antisepsis procedures must be employed by all who work with these animals. The use of gloves is especially pertinent when there is a risk of contact with body fluids. Infected animals should be placed in singly housed enclosures.
4.4. Main findings of this study
It is interesting to note that PCA analysis is distinguishing the canine group from the feline group on the basis of antibiotic resistance profile. It suggests that there is no widespread cross-transmission of MRSA isolates between the two different animal specimens. Separate enclosures of the tested animals may be a reason for this finding. Another possible explanation for this observation is that different MRSA isolates may adapt to specific hosts and if cross-transmission occurs, the new host is not suitable for the growth of the bacteria. It is noteworthy that one feline isolate is similar to the canine group in the PCA analysis for the antibiotic resistance profile, suggesting the possibility of feline-canine transmission. However, since evidence of cross-transmission is based on a single isolate, it is inconclusive to measure the rate of cross-transfer between species. Future work should include testing of larger sample sizes from both animal species to better elucidate the possibility of cross-transmission.
5. Conclusion
The close contact between humans and animals have led to the zoonotic spread of community acquired MRSA (CA-MRSA). From the study, it is clear that MRSA was present in both animals. However, the pattern of colonisation may vary in both animals. There were slightly higher MRSA isolates detected in canines compared to the felines. A difference in host enterotype may result in different antibiotic profiling of the isolates. Majority of the isolates were resistant to four antibiotics of the five tested. Furthermore, MRSA colonisation in animals is a cause of concern as they have become established in various animal species other than canines and felines such as pigs, horses and cattle. In addition to careful administration of antibiotics, newer drugs must be developed and more effective molecular methods should be employed to detect these microorganisms earlier in animals and humans.
Funding
The authors are grateful to the Malaysian Medical Association Foundation for funding this work and to Perdana University as well as Universiti Putra Malaysia for providing research facilities.
Additional information
Notes on contributors
Rajwin Raja Kanagarajah
This work is the core research interest of Animal Neighbours, an initiative led by Sharmini Julita Paramasivam, focusing on microbiological and behavioral zooanthroponotic and anthropozoonotic transmissions of antimicrobial resistant bacteria in non-clinical settings. In this particular work, the prevalence of methicillin resistant Staphylococcus aureus (MRSA) among canines and felines housed in animal shelters in Malaysia and the antibiotic profiling of these isolates relating to their host types were carried out. As there is limited data on the prevalence of MRSA among stray animals in South-East Asia in non-conventional, non-clinical settings, this research aims to reveal the prevalence of MRSA amongst these animals in the region, hence, the sample collection from mainly local breeds of canines and felines in Malaysia, which may differ in prevalence compared to other regions of the world due to varied prescribing practices and different existing guidelines for shelters as well as adoption processes. This research also aims to highlight the importance of practising antibiotic stewardship when treating companion animals.
References
- Aldridge, S., Parascandola, J., & Sturchio, J. (1999). The discovery and development of penicillin 1928–1945. Washington, DC: American Chemical Society.
- Attili, A., Preziusoa, S., Ngu, V. N., Cantalamessaa, A., Moriconia, M., & Cuteri, V. (2016). Clinical evaluation of the use of enrofloxacin against Staphylococcus aureus clinical mastitis in sheep. Small Ruminant Research, 136, 72–77.10.1016/j.smallrumres.2016.01.004
- Beutin, L. (1999). Escherichia coli as a pathogen in dogs and cats. Veterinary Research, 30(2–3), 285–298.
- Carlet, J. (2012). The gut is the epicentre of antibiotic resistance. Antimicrobial Resistance and Infection Control, 1(1), 39.10.1186/2047-2994-1-39
- Cefai, C., Ashurst, S., & Owens, C. (1994). Human carriage of methicillin-resistant Staphylococcus aureus linked with pet dog. The Lancet, 344(8921), 539–540.10.1016/S0140-6736(94)91926-7
- Chaban, B., Ngeleka, M., & Hill, J. E. (2010). Detection and quantification of 14 Campylobacter species in pet dogs reveals an increase in species richness in feces of diarrheic animals. BMC Microbiology, 10, 73.10.1186/1471-2180-10-73
- Cockerill, F., Wikler, M., Alder, J., Dudley, M., Eliopoulos, G., Ferraro, M., … Zimmer, B. (2012). Performance standards for antimicrobial disk susceptibility tests. Approved standard (Vol. 32, 11th ed., p. 58). Wayne, PA: CLSI (Clinical and Laboratory Standards Institute).
- Cosgrove, S., Sakoulas, G., Perencevich, E. N., Schwaber, M. J., Karchmer, A. W., & Carmeli, Y. (2003). Comparison of mortality associated with methicillin‐resistant and methicillin‐susceptible Staphylococcus aureus Bacteremia: A meta‐analysis. Clinical Infectious Diseases, 36, 53–59.10.1086/cid.2003.36.issue-1
- El-Tras, W. F., Holt, H. R., & Tayel, A. A. (2011). Risk of Toxocara canis eggs in stray and domestic dog hair in Egypt. Veterinary Parasitology, 178(3–4), 319–323.10.1016/j.vetpar.2010.12.051
- Enright, M. C., Robinson, D. A., Randle, G., Feil, E. J., Grundmann, H., & Spratt, B. G. (2002). The evolutionary history of methicillin-resistant Staphylococcus aureus (MRSA). Proceedings of the National Academy of Sciences, 99(11), 7687–7692.10.1073/pnas.122108599
- Gardam, M. A. (2000). Is methicillin-resistant Staphylococcus aureus an emerging community pathogen? A review of the literature. Canadian Journal of Infectious Diseases, 11(4), 202–211.10.1155/2000/424359
- Hiramatsu, K., Cui, L., Kuroda, M., & Ito, T. (2001). The emergence and evolution of methicillin-resistant Staphylococcus aureus. Trends in Microbiology, 9(10), 486–493.10.1016/S0966-842X(01)02175-8
- Hiramatsu, K., Katayama, Y., Yuzawa, H., & Ito, T. (2002). Molecular genetics of methicillin-resistant Staphylococcus aureus. International Journal of Medical Microbiology, 292(2), 67–74.10.1078/1438-4221-00192
- Klevens, R. M., Morrison, M. A., Nadle, J., Petit, S., Gershman, K., Ray, S., … Fridkin, S. K. (2007). Invasive methicillin-resistant Staphylococcus aureus infections in the United States. JAMA, 298(15), 1763–1771.10.1001/jama.298.15.1763
- Lefebvre, S. L., Reid-Smith, R., Boerlin, P., & Weese, J. S. (2008). Evaluation of the risks of shedding salmonellae and other potential pathogens by therapy dogs fed raw diets in Ontario and Alberta. Zoonoses Public Health, 55(8–10), 470–480.10.1111/jvb.2008.55.issue-8-10
- Livermore, D. M. (2003). Bacterial resistance: Origins, epidemiology, and impact. Clinical Infectious Diseases, 36(Suppl 1), S11–S23.10.1086/344654
- Loeffler, A., Boag, A. K., Sung, J., Lindsay, J. A., Guardabassi, L., Dalsgaard, A., … Lloyd, D. H. (2005). Prevalence of methicillin-resistant Staphylococcus aureus among staff and pets in a small animal referral hospital in the UK. Journal of Antimicrobial Chemotherapy, 56(4), 692–697.10.1093/jac/dki312
- Lysnyansky, I., Gerchman, I., Mikula, I., Gobbo, F., Catania, S., & Levisohn, S. (2013). Molecular characterization of acquired enrofloxacin resistance in Mycoplasma synoviae field isolates. Antimicrobial Agents and Chemotherapy, 57(7), 3072–3077.10.1128/AAC.00203-13
- National Committee for Clinical Laboratory Standard. (2001). Performance standards for antimicrobial disc susceptibility tests (M2-A7 and M7-A5) (6th ed., Vol. 21, No. 1). 11th informational supplement 2001 (M100-S11), 1997.
- Ng, S. T., Lim, C. Y., Tan, C. S., Karim, A. A., Haron, H., Ahmad, N. S., & Murugaiyah, V. (2011). Emergence of vancomycin-resistant Staphylococcus aureus (VRSA). WebMed Central, 1–11.
- Oztoprak, N., Cevik, M. A., Akinci, E., Korkmaz, M., Erbay, A., Eren, S. S., … Bodur, H. (2006). Risk factors for ICU-acquired methicillin-resistant Staphylococcus aureus infections. American Journal of Infection Control, 34(1), 1–5.10.1016/j.ajic.2005.07.005
- Perkins, H. R., & Nieto, M. A. N. U. E. L. (1973). The significance of D-alanyl-D-alanine termini in the biosynthesis of bacterial cell walls and the action of penicillin, vancomycin and ristocetin. Pure and Applied Chemistry, 35(4), 371–381.
- Perkins, H. R., & Nieto, M. (1974). The chemical basis for the action of the vancomycin group of antibiotics. Annals of the New York Academy of Sciences, 235(1), 348–363.10.1111/nyas.1974.235.issue-1
- Ramli, S. R., Neoh, H. M., Aziz, M. N., & Hussin, S. (2012). Screening and detection of heterogenous vancomycin intermediate Staphylococcus aureus in Hospital Kuala Lumpur Malaysia, using the glycopeptide resistance detection etest and population analysis profiling. Infectious Disease Reports, 4(1), 20.10.4081/idr.2012.3836
- Rich, M., & Roberts, L. (2004). Methicillin-resistant Staphylococcus aureus isolates from companion animals. Veterinary Record, 45, 591–597.
- Steneroden, K. K., Hill, A. E., & Salman, M. D. (2011). Zoonotic disease awareness in animal shelter workers and volunteers and the effect of training. Zoonoses Public Health, 58(7), 449–453.10.1111/jvb.2011.58.issue-7
- Strommenger, B., Kehrenberg, C., Kettlitz, C., Cuny, C., Verspohl, J., Witte, W., & Schwarz, S. (2006). Molecular characterization of methicillin-resistant Staphylococcus aureus strains from pet animals and their relationship to human isolates. Journal of Antimicrobial Chemotherapy, 57(3), 461–465.10.1093/jac/dki471
- Tacconelli, E., De Angelis, G., Cataldo, M., Pozzi, E., & Cauda, R. (2008). Does antibiotic exposure increase the risk of methicillin-resistant Staphylococcus aureus (MRSA) isolation? A systematic review and meta-analysis. Journal of Antimicrobial Chemotherapy, 61(1), 26–38.
- Tambekar, D., Dhanorkar, D., Gulhane, S., & Dudhane, M. (2007). Prevalence and antimicrobial susceptibility pattern of methicillin resistant Staphylococcus aureus from healthcare and community associated sources. African Journal of Infectious Disease, 1(1), 52–56.
- Team R.C. (2012). R: A language and environment for statistical computing. Vienna: Author.
- Tiwari, H. K., & Sen, M. R. (2006). Emergence of vancomycin resistant Staphylococcus aureus (VRSA) from a tertiary care hospital from northern part of India. BMC Infectious Diseases, 6, 520.10.1186/1471-2334-6-156
- Weese, J., Dick, H., Wiley, B. M., Mcgeer, A., Kreisweirth, B. N., Innis, B., & Low, D. E. (2006). Suspected transmission of methicillin-resistant Staphylococcus aureus between domestic pets and humans in veterinary clinics and in the household. Veterinary Microbiology, 115(1–3), 148–155.10.1016/j.vetmic.2006.01.004