Abstract
Implementation of terrestrial laser scanning creates new opportunities in the analysis of processes occurring in the environment. Terrestrial laser scanning (TLS) enables fast, precise mapping of land relief and dimensions of technical structures. TLS allows moving from traditional methods, such as point measurement, to differential analyses of a relief model. TLS allows accurate analyses of land relief changes and erosion phenomena, both in terms of the processes intensity and range, and the initial and intensifying events. The paper presents the authors’ experiment with the use of the TLS technology in erosion processes monitoring and analyses. The authors present the methodology of using TLS which contributed to the acquisition of reliable results by providing optimal conditions for the research. The paper shows the effects of laser scanning in order to analyse the intensity of soil erosion in mountainous forest area with a large number of measurement stations, which resulted in a dense points cloud. Differential model method allowed to evaluate erosion phenomena in the form of rills resulting from surface flushing, which is impossible to assess by traditional methods of erosion measurements. In the paper, we showed the necessity to choose the proper coordinate system (object or scanner) for the analysis of the landslide, depending on structure and direction of the soil masses movement. The application of the object coordinate system showed greater erosion phenomena range (ca 12%) on buttress stabilizing landslide and ca 44% on natural landslide, in which the application of the object coordinate system increases the number of observations by 40%.
Public Interest Statement
We would like to share our experience with using terrestrial laser scanning (TLS) in monitoring the land relief changes and qualitative and spatial assessment of the water erosion processes. The paper presents the conditions of using the TLS technology in erosion phenomena research. We were studied the erosion processes both occurring in natural areas locally on forest skid trails and in the large scale as a landslide, and also on technical structures. Research indicates the importance of choosing the right equipment, coordinate system and spatial resolution, which affects on the quality of measurements, time and costs, and research results.
1. Introduction
The term erosion means the processes of destruction of the top layer of the ground by forces of nature, such as wind, water and gravity or by anthropogenic factors. The processes of the degrading effects include mainly the transformation of the soil and terrain and a change of water relations. Erosion can be observed and evaluated by the movements of particles and masses of soil, caused by water and wind, as well as by mass movement understood as movement of large volumes of soil materials (Prochal, Citation1987). All types and forms of erosion processes lead to levelling of the ground surface.
Analysis of water erosion processes requires knowledge of the intensity of the process under evaluation, the volume of transported material, and the location of erosion. In the case of spatial phenomena, such as surface erosion and mass movements such as soil creep, it is justified to determine where the erosion processes have occurred.
Traditional methods of surveying used in the assessment of erosion phenomena (Prochal, Citation1987; Prochal, Maślanka, & Koreleski, Citation2005) are based on levelling, typically in order to analyse changes in the thickness of topsoil or to determine the volume of sediment in streams and reservoirs. The use of conventional surveying tools, such as the leveller, allows only to execute point measurements. In the case of spatial phenomena, it is associated with the interpolation between the measured positions results. That leads to averaging the intensity of the phenomena. Using traditional methods for large areas is possible, but also involves a significant investment of time, work, and increases the risk of measurement errors.
Another spatial distribution analysis method is assessment by determining the amount of sediment deposition in the runoff receivers and transported erosion material (Jała & Cieślakiewicz, Citation2004; Pierzgalski, Janek, Kucharska, Tyszka, & Wróbel, Citation2007). Similar studies were conducted in the context of sediment accumulation in reservoirs (Czamara, Citation1992). However, one of the most common methods for measuring surface erosion (sheet erosion) and mass movements (soil creep) is to measure the volume of eroded material retained in the grippers (catchers)—these are temporary structures, usually made of impermeable synthetic material mounted on a rack, or in septic tanks, whose task is to capture the erosion material from the run-off. This methodology allows to perform an analysis of eroded material in terms of its weight and volume.
Field studies and analyses of erosion processes resulting from natural precipitation are carried out in many countries. These types of researches are similar not only in terms of the causative factor being natural, but also in the methodology of measurement and design of equipment for measuring the intensity of run-off and erosion. However, there are different reasons for undertaking the researches. Benavides-Solorio and MacDonald (Citation2005) and Larsen, MacDonald, Brown, Rough, and Welsh (Citation2009) conducted studies on the Colorado Front Rage (USA), where catchers were used to analyse the intensity of surface erosion in the area of a fire and the factors that cause erosion in these specific circumstances. Black and Luce (Citation2013) undertook the analysis of erosion on forest roads, by draining run-off from the road surface with transported debris to septic tanks. Furthermore, the correlation of intensity of precipitation, runoff intensity, and volume of sediment were analysed in relation to the road area and its structure. Similar studies have also been conducted in Poland. One of them was realised by Smolska (Citation2002) in the Suwałki Lake District. Evaluation of spatial erosion processes may also be carried out using representative experimental plots. Studies on those dedicated test stands—plots - may be conducted in the laboratory as a result of simulated precipitation, as well as in the field under natural conditions (Rejman & Usowicz, Citation2002; Ruiz-Sinoga, Romero-Diaz, Ferre-Bueno, & Martínez-Murillo, Citation2010). Martínez-Zavala, Jordán López, and Bellinfante (Citation2008) developed the impact of topography and vegetation on soil erosion intensity using simulated rainfall on several plots in forest areas by measuring the amount of run-off and eroded material. Similar studies were also carried out in Spain by Jordán and Martínez-Zavala (Citation2008).
TLS technology has changed the way of analysing the natural phenomena like erosion processes. Geodesy surveying has evolved into 3D models analyses (Barbarella & Fiani, Citation2013; Barbarella, Fiani, & Lugli, Citation2015; Coppa, Guarnieri, Pirotti, Tarolli, & Vettore, Citation2013; Denora, Romano, & Cecaro, Citation2013; Guzzetti et al., Citation2012; Vosselman & Maas, Citation2010). The use of laser technology in the research of erosion phenomena is becoming more and more common in the scientific community. Such research was conducted by Dąbek in terms of surface flushing on forest roads in the Sudetes, Poland (Dąbek, Żmuda, Ćmielewski, & Szczepański, Citation2014), Ballesteros-Cánovas in terms of influence exposed roots on sheet erosion in experimental approach in Barranca de los Pinos, Spain (Ballesteros-Cánovas, Corona, Stoffel, Lucia-Vela, & Bodoque, Citation2015), Stenberg in terms of erosion of peatland forest ditches, Finland (Stenberg et al., Citation2016), Eltner in terms of several years soil erosion research on small, unpaved fields in Spain and Germany simultaneously showing DTM comparison obtained from TLS and photogrammetry from UAV (Eltner, Mulsow, & Maas, Citation2013) and many others. No homogeneous or unambiguous procedures and techniques have been developed for the investigation and monitoring of erosion processes on technical structures or in the natural environment. Such guidelines could be necessary in terms of determining the optimal density of the point cloud, the number of scanner stations relative to the research area, application the coordinate system (object or scanner), methods for interpolating the point cloud to DTM and others. The analysis of the topic and the research we conducted were the reason for preparing such a study based on our experience in the use of TLS in the research of various forms of erosion phenomena.
The authors’ aim was to demonstrate the usability of modern and rapidly evolving technology—terrestrial laser scanning (TLS)—for monitoring eroded areas. The main aim of the paper was to determine the conditions for the using TLS in research of the qualitative and quantitative assessment of erosion processes, measurement intensity and range of erosion and monitoring of these phenomena. We analyse the conditions of using TLS in the terms of the adopted measurement accuracy, density of point cloud, application the coordinate system, interpolation to DTM and adopting the differential model method for further analysis. We conducted analyses in terms of erosion processes in the form of landslides on a technical structure and in the natural environment, as well as in the form of sheet and rill erosion on the example of forest areas of operation trials.
2. Methods and materials
Laser scanners allow to take inventory of an area with a high resolution. The results are presented as a point cloud (Afana, Solé-Benet, Pérez, Gilkes, & Prakongkep, Citation2010). This way, the collected data allow to perform quantitative and qualitative analysis of the occurring phenomenon. The economic criteria have been also discussed, such as the time and ease of measurement (a single measurement session does not exceed a few hours of field work), as well as the possibility of stepping into previously inaccessible areas of analyses.
2.1. Types of laser scanners
Two technologically different scanners are used to obtain data (Vosselman & Maas, Citation2010). A pulse scanner (like Leica ScanStation2 that was used in our researches) and a phase scanner (like Faro Focus3D).
The phase laser scanner measurements are based on comparing the phase shift of the emitted and returned laser light. This type of scanner usually uses a signal with a sinusoidal modulation. This system is at risk of losing one full cycle. In order to avoid this error, a plurality of frequencies is used. The low frequency of the long wavelength determines the scope, while the shortness of the wavelength determines the accuracy that can be obtained.
Measurement using a phase scanner is easy because the scanner size allows to transport the device. Due to measurement noise, the data are automatically filtered during the measurement. Consequently, many points of the object cloud are removed by filtration algorithms, making this type of scanner less useful in the measurement. Because of that there are places for which the data are inaccurate or missing. However, in other areas, it is possible to determine the areas of erosion processes.
Distance measurement method used in pulsed scanners is based on recording the time of emitting and returning reflected laser light wave from the object. Knowing this time, as well as the speed of light and atmospheric factors, it is possible to count twice the distance from the object to the scanner. Therefore, there is a need to use high-precision timers.
The way the two instruments measure the distance entails different work parameters of the devices. A phase scanner achieves a much higher measurement speed (more than one million points per second), but its range is usually twice shorter, while a pulse scanner is characterised by a much lower measurement noise (Vosselman & Maas, Citation2010).
2.2. Methodology of analysis
The data acquired from a terrestrial laser scanner must be filtered, especially in natural areas where vegetation can be very dense. To clean point clouds form noise, it is possible to use several algorithms. Points representing the terrain surface are located the lowest in the cloud. In this work, a programme designed to filter data from a laser scanner was used. TerraScan used the active algorithm TIN (Brodowska, Citation2012).
After reducing the noise, a set of points representing the surface of the terrain was analysed using geostatistical tools—Surfer v10. Algorithms implemented in the software create digital terrain models (DTM) using different interpolation methods such as kriging, triangulation, nearest neighbour method and others, allowing a thorough analysis of the data-set. The study is based on kriging because it reflects the terrain best (Chaplot et al., Citation2006; Czamara, Citation1992; Oliver & Webster, Citation1990). Using the primary measurement filtered data and the control one, a DTM for the study objects was developed. The primary DTM was subtracted from the control DTM, resulting in a differential model of the terrain. The obtained model allows to see the changes that have been taking place around the facility, as well as their extent. It should be reminded that the laser scanning method is one of quantitative methods and as such it determines the location of the effects of the phenomena on the entire object area. An in-depth analysis of the area models can provide data concerning phenomena intensity.
2.3. Study areas
2.3.1. Forest skid trail in a mountain area
Human influence on the natural balance of forests, including establishing networks of forest roads and trails or logging, and the effects of these treatments, negatively affects the forest soil protection function (Chang, Citation2003; Kusiak & Jaszczak, Citation2009). Changing the land use, the landform (Burley, Evans, & Youngquist, Citation2004; Prochal et al., Citation2005), and the soil’s shear strength and the ability of soil to resist force working parallel to the soil surface which is the result of using the land for transport, leads to intensification of soil erosion processes. Erosion phenomena are a result of concentration run-off on forest roads and trails. Chang (Citation2003) shows that the roads and trails network is essential for the management of forests, but as much as 90% of the erosion material deposited in streams is derived from road surface. In areas with heavy relief, erosion occurring on road scarps located on slopes could generate 70–90% soil loss from the entire network in the area. Also Croke, Hairsine, and Fogarty (Citation2001) showed that the erosion on skid trails (trails used to transport timber from a harvest area to a storage place) is approximately 30-fold greater than erosion from the surface of harvesting. The most differences are observed 1–2 years after the logging.
Therefore, identifying the erosion and hydrologic processes and the intensity of these phenomena in the context of skid trails, as well as the effects of anthropogenic activities, is a legitimate subject. To perform such field measurements TLS technology was used (Figure ). TLS allows the analysis of changes in local land relief in relation to the entire experimental plot, taking into account changes in the structure of the terrain in the vertical axis (depth of rills—the effect of flushing the surface and rills erosion) and in the horizontal axis (width and length of rills), as well as the spatial arrangement. This allows to analyse all the formed rills network and changes in the thickness of the topsoil. TLS also allows to analyse the line of the runoff concentration and to perform quantitative evaluation of water erosion.
Figure 1. Terrestrial laser scanning on land relief analyses. The Szklarska Poręba Forestry District.
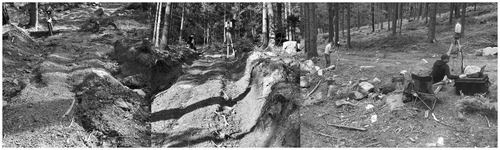
The experimental area was located in the western part of the Sudetes, in the Izera Mts., Poland, on the slope of Ciemniak (GPS N: 50° 51′ 33″; E: 15° 34′ 45″). The area under study is a forest area managed by the Szklarska Poręba Forest District (The State Forests National Forest Holding). In the analysed area, timber logging was conducted until the end of 2010 according to the planned activities. For proper and efficient conduct of logging, skid trails have been designated and located in accordance with the State Forests regulations. Setting and using the trails causes topsoil degradation, changes in local land relief, and thus contributes to the intensification of soil erosion processes on the forest slopes. The increase in erosion phenomena on the trails changed the stability of the topsoil (Chang, Citation2003). With the end of the logging, a selected section of the trail was chosen and an experimental area was created. The change of local land relief of the trail (Figure ) was documented from the skidding time until the end of the research, focusing on the erosion effects emerging after the works.
2.3.2. Anthropogenically transformed area
The next example are erosion processes occurring on land transformed by human activity—buttress stabilising landslide (Figure ) on the rail trail in Mazurowice, Poland (GPS N 51° 12′ 53,94″, E 16° 27′ 43,44″). Deep cuttings and high embankments favour the occurrence of mass movements, especially if they are located on cohesive soils (Figure ).
The railway track in this area is situated on ground built with cohesive soils, including mostly Tertiary clays. The terrain forced the location of the E-30 railway line on high embankments, h > 6.0 m, and in a deep excavation, which creates a greater risk of landslides. The research area is located on the edge of the plateau of Malczyce. In the upper part of the slope, drilled geotechnical bores (3.0–7.0 m depth) revealed the Quaternary formations of Middle Polish Glaciation, in the deeper parts - sand over Tertiary formations. Cohesive soils occurring in the area, with numerous interbeds of silt, were classified as silty clays and loess-like sand over Tertiary formations. The soils in the subsurface layers (0.6–3.8 m depth) occur in a firm state (IL = 0.3), and deeper in a stiff state (IL = 0.2). Deposits (to 2.3 m depth) were classified as embankment material due to a variety of drilled material and numerous interbeds. In cohesive soils (to 4.3 m depth), numerous interbeddings in the form of hydrated medium sands were observed. The ceiling of Tertiary clays was observed at 4.2–4.3 m depth on ~121.00 m a.s.l. These deposits are in a stiff (IL = 0.2), semi-hard (IL = 0.0) and locally in a firm state (IL = 0.3). Tertiary formations included also soils in the form of medium sand, sandy gravel, sandy clay and silt which formed local interbeddings. The landslides colluvium and the direction of rainwater runoff were determined.
2.3.3. Landslide area
In 1997, after the July flood in the Sudetes region, a landslide occurred in the area of Janowiec village, Poland, on the slope of Kurzyniec Mt. (GPS N 50° 30′ 18,53″, E 16° 44′ 57,50″). The landslide area is located on Quaternary formations, in particular on deluvial clay with a content of rubble. The oldest rocks in the area are revealed in the erosion indentations as mudstones, clays, greywacke sandstones assigned to Lower Carboniferous formation from Opolnica (Cwojdziński & Pacuła, Citation2009; Oberc, Citation1987). The development and geological position of Lower Carboniferous deposits in the Bardzkie Mts. is shown in numerous studies (Chorowska & Radlicz, Citation1994; Chorowska & Wajsprych, Citation1995; Finckh, Citation1932; Oberc, Citation1987; Wajsprych, Citation1995; Wajsprych, Citation1978, Citation1986).
The landslides of Quaternary formations situated directly on the Carboniferous formation created a phenomenon. The main slope is clearly defined in the morphology, numerous secondary slopes and crevices are located in the middle part of the landslide, and the tongue of the landslide is poorly formatted (Figure ). The study area is bounded by deep erosion valleys oriented transversely to the slope (up to 8 m depth). At the bottom, strongly fractured Carboniferous shales are exposed. In the eastern canyon, due to backward erosion, a sub-notch is developing in the SW-NE direction. This direction is analogous to the slopes. It may reflect the neotectonic movements related with a system of the Sudetes Marginal Fault that occur along the sub-block faults (Badura et al., Citation2003; Krzyszkowski, Migoń, & Sroka, Citation1995).
The landslide moves directly to the bottom of the Nysa Kłodzka valley (Figure ). The area of the landslide is about 1.1 ha (130 m length, 120 m width), and it reaches about 20–25 m above the level of the river. However, this did not result in flow limitation in river Nysa Kłodzka, due to the small volume of moving mass. The landslide is located from 295 m a.s.l. to the bottom of the Nysa Kłodzka valley (225 m a.s.l.). The landslide was founded on the northern slope of the Kurzyniec Mt. In the upper part of the investigated area the slope does not exceed 5°. On the edge of the Holocene valley of the Nysa Kłodzka, following the Sudetes fault, the slope attains even 30°. The area of the landslide forehead is covered mostly with deciduous trees, the upper part of the landslide is agriculturally used (Figure ).
One of important elements in research on landslides is to determine the area and micromorphology of the phenomenon. Several methods can be used for surface measurements, like tachymetry, GNSS, hand-held GPS loggers. It is also important that the data are intended to be used at a later stage of deformation research. One of the methods—modern and relatively fast allowing for the accurate reproduction of micromorphology as well as allowing for deformation analyses is Airborne laser scanning (ALS) (Pawłuszek & Borkowski, Citation2016) or close range photogrammetry from unmanned aerial vehicle (UAV). Landslides located in the mountainous region are situated in areas usually covered dense vegetation where those methods do not allow for capture occurring changes of terrain morphology because of occurrence a large covering of high vegetation. With TLS method we can work below the vegetation, directly from the ground, we also obtain lots of additional information while scanning, like vegetation. Using filtration data can be classified into classes of vegetation (low, medium and high) and the ground class.
3. Results
3.1. Forest skid trail in the mountain area
Applying TLS made it possible to automatically measure and to produce measurable and fully objective models of the terrain in a short time. Scanning resolution was scheduled and set as a grid of 3 × 4 mm at a distance of 10 m. The accuracy of scan registration and adjustment for a single measurement campaign did not exceed ±3 mm. The average density of the point cloud after filtration in different sectors of skid trail on 1 m2 is shown in Figure . Data prepared in such a way were imported into Surfer10, where the interpolations of the point cloud and the basic analyses were done. GRID with a density 1 × 1 cm was created. As a result of the measurements and conducted analyses, the areas of soil erosion occurrence and the areas of eroded material deposition were determined. Analysis of DTM created from laser scanning also allowed to calculate precisely the intensity of the erosion processes. Interpolation of point cloud (obtained from the scanning) to resolution of 1 × 1 cm allowed accurate assessment of erosion rills, even for their minimal depth. In comparison, data interpolation with lower resolution or using traditional methods such as levelling would not show such small changes in local land relief. As a result of inaccurate interpolation or measurement errors, the magnitude of erosion processes may be underestimated or excessively averaged.
To present the research results, the lower section of the analysed trail, which ends with an anti-erosion barrier, was chosen. The area of the selected section is 131 m2 in total, with an average width of the trail at about 2 m. The section is characterised by an average decrease of about 20%. The density of the point cloud from TLS for the selected trail section is above 33,000 points per m2 (Figure ). This accuracy of data allowed to create DTMs of this area, to carry out the analyses and to interpret the results.
The analysis of changes in local land relief was obtained by comparing the subsequent models. The data from scanning were acquired in June, August and November, 2011. The effects of water erosion during that period are shown in Figure .
Figure 9. Contour map of the differential model of the skid trail section (the scanner coordinate system), model of June–August.
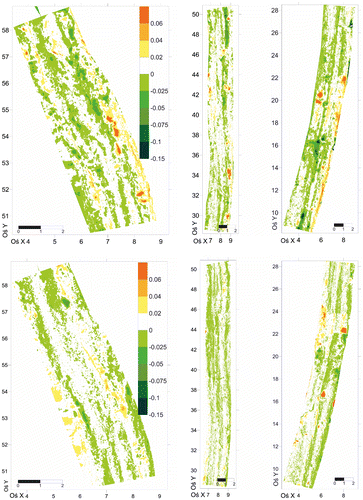
Local land relief changes, resulting from erosion processes, occurred in more than 38% of the analysed section between June and August, and in about 33% between August and November, 2011. Run-off concentration lines were formed in the ruts, caused by the movement of heavy forestry tractors and timber hauling. Erosion rills, with an average depth of 1–10 cm, were formed in those lines. At the same time, terrain elevation outside the ruts was reduced by approximately 15 cm. Analysis of the DTMs also shows intensive erosion occurring on trail scarps. The dimensions of the formed rills and their location on the trail area indicate that the intensity of erosion depends on local land relief of the trail, local slope, and varying topsoil stability. During the research period, the estimated volume of eroded material in the analysed area was approximately 0.9 m3. A half of this volume was eroded in the first part of the study. This value was calculated as the volume of all the rills formed on the analysed trail section.
3.2. Anthropogenically transformed area
Two measurement campaigns were performed, with an interval of about one year. Both measurements referred to the local survey matrix, located outside of the object impact. The result of the inferred overlapping point clouds is shown in Figure . Registration of the objects in a common spatial arrangement and graphical presentation shows the scale of the phenomenon. The sectors of evenly coloured penetrations (point cloud) suggest a low intensity of the phenomenon. Intensification of one colour indicates greater intensity of erosion.
Figure 10. Point cloud of output (green colour) and control measurement (red colour) of the Mazurowice landslide.
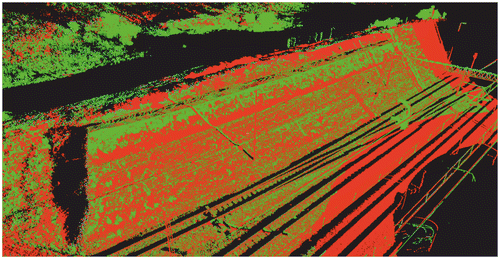
The analysis of the deformation processes was done in two ways: in the scanner coordinate system (Z-axis directed along the vector of gravity but in the opposite direction) and in the object coordinate system (Z-axis directed perpendicularly to the plane fitted to the research object). The aim of this was to improve the accuracy of the analysis by better interpolation in connection with the extension of the object area (Figure ).
As a result of the calculations the DTMs were developed. Next, the differential models were created (control DTM was subtracted from the output DTM). This procedure was performed for the two cases—the scanner and the object coordinate system (Figure ). The results are presented in Figure .
Figure 12. Escarpment of excavation—the object coordinate system: DTM from the control measurement (top, January 2012), DTM from the output measurement (middle).
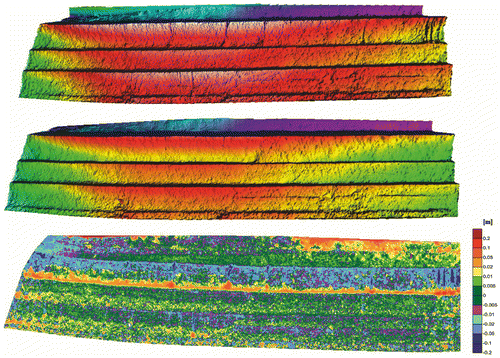
Figure 13. Comparison of differential models from the scanner coordinate system (top) and the object coordinate system (bottom).
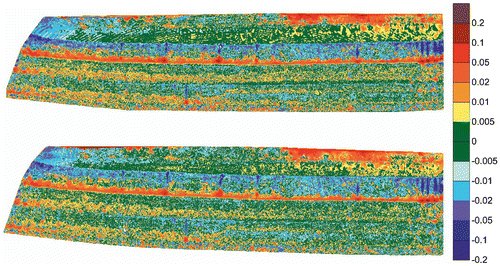
Both the differential models show object deformation, however, it can be assumed that displacements are not associated with the object movements but with erosion processes—ablation. The differential model coordinate systems show (Figure ) that the largest changes of land relief occur in the upper part of the object, which is due to the design of the buttresses—in the lower parts it is made of crushed stones and the upper part is made of sand. The sand was eroded and deposited on the shelf formed by the first layer of the crushed stone.
To examine the distribution of displacements occurring on the object (Figure ), two histograms were created. While comparing the two histograms, the greater frequency of erosion phenomena (about 12%) can be observed in the object coordinate system. Such a manner of presentation of the changes taking place on the area allows a more detailed analysis of local land relief, especially on very steep slopes.
3.3. Landslide
In the opinion of the authors, the best way to inventory such an area is to perform a large number of scans at various positions, with a short measurement time. That reduces the amount of shade, does not take a lot of time, and allows to represent the terrain micromorphology accurately. After filtration of the data obtained from TLS, the points of the vegetation class are ca 75% and those of the ground class are ca 25% of the total acquired information. Using TLS allows to develop a digital terrain model representing both the area of the landslide and its micromorphology (Figure ). Analysis of the deformation of the landslide obtained from TLS is possible after control measurement. The results of the measurement campaigns should be compared with each other by performing the differential model. In our presentation of the method, we focus primarily on the head of the landslide as representing the best the occurring transformation of the landslide.
As a result of subsequent measurements which were filtered and oriented to a common reference system, we obtained DTMs of the scanner and the object coordinate system (Figures and ). As a result it was possible to determine the areas where the mass movement phenomenon is intensified, and to compute the volume of the eroding material.
Figure 16. Forehead of the landslide—the object coordinate system: DTM from the control measurement (top, 2013), DTM from the output measurement (middle, 2010).
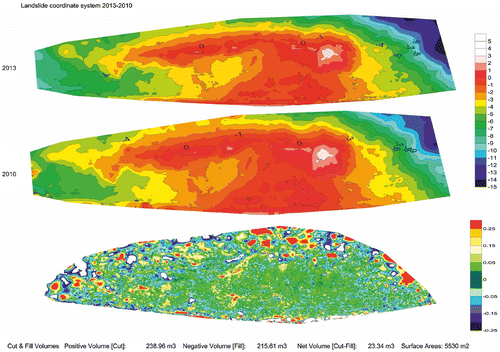
Figure 17. Forehead of the landslide—the scanner coordinate system: DTM from the control measurement (top, 2013), DTM from the output measurement (middle, 2010).
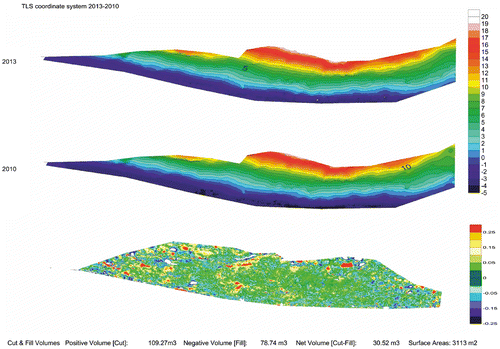
Comparing these two representations of occurring phenomenon it can be seen that using the object coordinate system the area of deformation increases by ca 44%. Analysing the histograms (Figure ) of the differential models, the number of observations also increases (ca 40%). In both approaches the net volume of eroding material is positive, which suggests bloating of the landslide. In the authors’ opinion, more reliable results are obtained using the object coordinate system, because the observed landslide area is more perpendicular to the Z axis. The use of the object coordinate system improves the quality of interpretation of the occurring phenomenon, which significantly affects the reliability of the method.
4. Conclusions
The use of modern techniques and technologies for measuring and processing data in research on erosion phenomena now seems to be natural, easy, faster and giving better and more accurate results than traditional methods. The use of measurement techniques such as TLS, ALS or photogrammetry in combination with geostatistical programmes and GIS gives almost unlimited possibilities, as well as better recognition of the characteristic of erosion processes, their assessment and monitoring. However, the use of modern technologies, measurement techniques and data processing methods requires the adoption of certain assumptions and conditions that need to be defined.
The application of terrestrial laser scanning allows to perform accurate evaluation of the terrain condition, in natural areas (landslide) as well as in anthropogenically transformed ones, such as forest trails and roads or railway embankments and cuttings. The area of erosion processes may be accurately located by means of control measurements. The adopted method allowed rapid assessment of the intensity of these processes and the calculation of the erosion material volume. In comparison with the traditional methods, field measurements are relatively quick and easy to conduct. The methodology has another important advantage—it is possible to identify precisely the areas where intensive erosion processes occur. Changing the scanner coordinate system to the object one increases the accuracy of determining the area of the phenomenon and its intensity on steep surfaces.
The applied automatic laser measurement technology allows to perform modelling of the erosion phenomena that take place on skid trails in mountain forest areas. The traditional measuring method based on catchers allows to evaluate the volume of erosion material. However, this method limits the trails usability. The TLS method does not limit the functionality of the terrain, and the applied resolution gives very accurate results of the erosion processes in terms of volume, variability in time and spatial range of the phenomenon. The study results concerning water erosion in forests are crucial for managing such areas, including damage in forests and small-scale retention, as well as for the cognitive and scientific aspects of erosion.
Erosion processes of landslide (on anthropogenically transformed and natural objects) in the top layer of buttresses built of loose soil are well represented. On the rest of the analysed object, possible processes of water erosion are within the range of scanner measurement error. By transformation of the scanner coordinate system to the object coordinate system, the number of observations increased, making it possible to accurately identify the areas of occurrence of intensive erosion processes.
Funding
The authors received no direct funding for this research.
Additional information
Notes on contributors
Paweł B. Dąbek
Paweł Dąbek has been awarded with a PhD degree in specialty of Water Management, Water Erosion of Soil and Forest Engineering for thesis “The water erosion processes of Soils in forest areas in the mountain catchment” in 2017 from Wrocław University of Environmental and Life Sciences, Poland, where he is currently working as a research and teaching assistant. His research interests are GIS, forest engineering, small scale water retention and soil erosion.
References
- Afana, A., Solé-Benet, A., Pérez, J. C., Gilkes, R. J., & Prakongkep, N. (2010, August 1–6). Determination of soil erosion using laser scanners. In Proceedings of the 19th World Congress of Soil Science: Soil solutions for a changing world (pp. 39–42). Brisbane. Published on DVD.
- Badura, J., Zuchiewicz, W., Górecki, A., Sroka, W., Przybylski, B., & Żyszkowska, M. (2003). Morphotectonic properties of the Sudetic Marginal Fault, SW Poland. Acta Montana A, 24(131), 21–49.
- Ballesteros-Cánovas, J. A., Corona, C., Stoffel, M., Lucia-Vela, A., & Bodoque, J. M. (2015). Combining terrestrial laser scanning and root exposure to estimate erosion rates. Plant and Soil, 394(1–2), 127–137. doi:10.1007/s11104-015-2516-3
- Barbarella, M., & Fiani, M. (2013). Monitoring of large landslides by Terrestrial Laser Scanning techniques: Field data collection and processing. European Journal of Remote Sensing, 45, 126–151. doi:10.5721/EuJRS20134608
- Barbarella, M., Fiani, M., & Lugli, A. (2015). Landslide monitoring using multitemporal terrestrial laser scanning for ground displacement analysis. Geomatics, Natural Hazards and Risk, 6(5–7), 398–418. doi:10.1080/19475705.2013.863808
- Benavides-Solorio, J. D. D., & MacDonald, L. H. (2005). Measurement and prediction of post-fire erosion at the hillslope scale, Colorado Front Range. International Journal of Wildland Fire, 14(4), 457–474. doi:10.1071/WF05042
- Black, T. A., & Luce, C. H. (2013). Measuring water and sediment discharge from a road plot with a settling basin and tipping bucket (Gen. Tech. Rep. RMRS-GTR-287, 38 pp.). Fort Collins, CO: U.S. Department of Agriculture, Forest Service, Rocky Mountain Research Station.10.2737/RMRS-GTR-287
- Brodowska, P. (2012). Comparison of TIN active model and prediction of linear algorithms for terrain points segmentation. Archiwum Fotogrametrii, Kartografii i Teledetekcji, 24, 63–71 (in Polish).
- Burley, J., Evans, J., & Youngquist, J. A. (Eds.). (2004). Encyclopedia of forest sciences (2061 pp). Cambridge, MA: Academic Press.
- Chang, M. (2003). Forest hydrology: An introduction to water and forests (p. 373). Boca Raton, FL: CRC Press LLC.
- Chaplot, V., Darboux, F., Bourennane, H., Leguédois, S., Silvera, N., & Phachomphon, K. (2006). Accuracy of interpolation techniques for the derivation of digital elevation models in relation to landform types and data density. Geomorphology, 77(1–2), 126–141. doi:10.1016/j.geomorph.2005.12.010
- Chorowska, M., & Radlicz, K. (1994). Revision of the age of the Lower Carboniferous deposits of the northern part of the Góry Bardzkie (Sudetes). Geological Quarterly, 38(2), 249–288.
- Chorowska, M., & Wajsprych, B. (1995). Góry Bardzkie Mts. Unit. In: The Carboniferous System in Poland. Prace Pafstwowego Instytutu Geologicznego, 148, 137–139.
- Coppa, U., Guarnieri, A., Pirotti, F., Tarolli, P., & Vettore, A. (2013). Comparing data acquisition methodologies for DTM production. International Archives of the Photogrammetry, Remote Sensing and Spatial Information Sciences - ISPRS Archives, 40(5W3), 59–62. doi:10.5194/isprsarchives-XL-5-W3-59-2013
- Croke, J., Hairsine, P., & Fogarty, P. (2001). Soil recovery from track construction and harvesting changes in surface infiltration, erosion and delivery rates with time. Forest Ecology and Management, 143, 3–12. doi:10.1016/S0378-1127(00)00500-4
- Cwojdziński, S., & Pacuła, J. (2009). Objaśnienia do arkusza Ząbkowice Śląskie (869) Szczegółowej mapy geologicznej Polski. Warszawa: Narodowe Archiwum Geologiczne (in Polish).
- Czamara, W. (1992). Prognoza erozji powierzchniowej w zlewniach zbiorników wodnych zlokalizowanych na przedgórzu sudeckim. Zeszyty Naukowe Akademii Rolniczej w Krakowie, 271, 79–89 (in Polish).
- Dąbek, P., Żmuda, R., Ćmielewski, B., & Szczepański, J. (2014). Analysis of water erosion processes using terrestrial laser scanning. Acta Geodynamica et Geomaterialia, 11(1, 173), 45–52. doi:10.13168/AGG.2013.0054
- Denora, D., Romano, L., & Cecaro, G. (2013). Terrestrial laser scanning for the Montaguto landslide (Southern Italy). In C. Margottini, P. Canuti, & K. Sassa (Eds.), Landslide science and practice (Vol. 2, pp. 33–38). doi:10.1007/978-3-642-31445-2_4
- Eltner, A., Mulsow, C., & Maas, H. G. (2013). Quantitative measurement of soil erosion from tls and uav data. ISPRS - International Archives of the Photogrammetry, Remote Sensing and Spatial Information Sciences, XL-1/W2(2), 119–124.10.5194/isprsarchives-XL-1-W2-119-2013
- Finckh, L. (1932). Erlaeuterungen zur geologischen Karte von Preussen und benachbarten deutschen Laendern 1:25 000. Blatt Frankenstein. Preussische Geologische Landesanstalt, 343.
- Guzzetti, F., Mondini, A. C., Cardinali, M., Fiorucci, F., Santangelo, M., & Chang, K.-T. (2012). Landslide inventory maps: New tools for an old problem. Earth-Science Reviews, 112(1–2), 42–66. doi:10.1016/j.earscirev.2012.02.001
- Jała, Z., & Cieślakiewicz, D. (2004). Potential erosion of soils in the Karkonosze National Park. In Geoekologicke problemy Krkonoš. Mez. Věd. Konf. November 2003, Szklarska Poręba. Opera Corcontica, 41, 66–73 (in Polish).
- Jordán, A., & Martínez-Zavala, L. (2008). Soil loss and runoff rates on unpaved forest roads in southern Spain after simulated rainfall. Forest Ecology and Management, 255(3–4), 913–919. doi:10.1016/j.foreco.2007.10.002
- Krzyszkowski, D., Migoń, P., & Sroka, W. (1995). Neotectonic quaternary history of the Sudetic marginal fault, SW Poland. Folia Quartern, 66, 73–98.
- Kusiak, W., & Jaszczak, R. (2009). Propedeutyka leśnictwa (143 pp.). Wyd. Uniwersytetu Przyrodniczego w Poznaniu (in Polish).
- Larsen, I. J., MacDonald, L. H., Brown, E., Rough, D., & Welsh, M. J. (2009). Causes of post-fire runoff and erosion: Water repellency, cover, or soil sealing? Soil Science Society of America Journal, 73(4), 1393–1407. doi:10.2136/sssaj2007.0432
- Martínez-Zavala, L., Jordán López, A., & Bellinfante, N. (2008). Seasonal variability of runoff and soil loss on forest road backslopes under simulated rainfall. Catena, 74(1), 73–79. doi:10.1016/j.catena.2008.03.006
- Oberc, J. (1987). Struktura bardzka jako reper rozwoju waryscydów wschodniej części Sudetów Zachodnich i ich przedpola. Przew, 58, 165–180 (in Polish).
- Oliver, M. A., & Webster, R. (1990). Kriging: A method of interpolation for geographical information systems. International journal of geographical information systems, 4(3), 313–332. doi:10.1080/02693799008941549
- Pawłuszek, K., & Borkowski, A. (2016, July 12–19). Landslides identification using airborne laser scanning data derived topographic terrain attributes and support vector machine classification. In The International Archives of the Photogrammetry, Remote Sensing and Spatial Information Sciences, XXIII ISPRS Congress (Volume XLI-B8). Prague. doi:10.5194/isprsarchives-XLI-B8-145-2016
- Pierzgalski, E., Janek, M., Kucharska, K., Tyszka, J., & Wróbel, M. (2007). Badania hydrologiczne w leśnych zlewniach sudeckich (84 pp.). Sękocin: IBL (in Polish).
- Prochal, P. (Eds.). (1987). Podstawy melioracji rolnych. PWRiL, Warszawa, 2, 419 (in Polish).
- Prochal, P., Maślanka, K., & Koreleski, K. (2005). Ochrona środowiska przed erozją wodną (126 pp). Kraków (in Polish).
- Rejman, J., & Usowicz, B. (2002, October). Ocena erozji wodnej gleb lessowych w oparciu o pomiary poletkowe. In Proccedings of conference: “Erozja gleb i transport rumowiska rzecznego”. Zakopane (in Polish).
- Ruiz-Sinoga, J. D., Romero-Diaz, A., Ferre-Bueno, E., & Martínez-Murillo, J. F. (2010). The role of soil surface conditions in regulating runoff and erosion processes on a metamorphic hillslope (Southern Spain). Catena, 80(2), 131–139. doi:10.1016/j.catena.2009.09.007
- Smolska, E. (2002, October). Erozja powierzchniowa gleb na Pojezierzu Suwalskim i niektóre jej uwarunkowania klimatyczno-topograficzne. In Proccedings of conference “Erozja gleb i transport rumowiska rzecznego”. Zakopane (in Polish).
- Stenberg, L., Tuukkanen, T., Finér, L., Marttila, H., Piirainen, S., Kløve, B., & Koivusalo, H. (2016). Evaluation of erosion and surface roughness in peatland forest ditches using pin meter measurements and terrestrial laser scanning. Earth Surface Processes and Landforms, 41, 1299–1311. doi:10.1002/esp.3897
- Vosselman, G., & Maas, H. G. (2010). Airborne and terrestrial laser scanning (p. 336). Dunbeath, Scotland: Whittles Publishing.
- Wajsprych, B. (1978). Allochthonous Paleozoic rocks in the Visean of the Bardzkie Mts. (Sudetes). Rocz Pol Tow Geol, 38(1), 99–128 (in Polish).
- Wajsprych, B. (1986). Sedimentary record of tectonic activity on a Devonian—Carboniferous continental margin (Sudetes). In IAS 7th European Meeting. Kraków – Poland. (pp. 141–164). Wrocław: Excursion Guide Book.
- Wajsprych, B. (1995). The Bardo Mts. rock complex: The Famennian – Lower Carboniferous preflysch (platform)-to-flysch (foreland) basin succession, the Sudetes. In O. Kumpera, M. Paszkowski, B. Wajsprych, J. Foldyna, J. Malec, & H. Zakowa (Eds.), Transition of the early carboniferous pelagic sedimentation into synorogenic flysch. An example from the Silesian-Moravian zone and adjacent areas. XIII International Geological Congress on Carboniferous-Permian (Vol. B2, pp. 23–42). Kraków: Guide to Excursion.