Abstract
We investigated behavioral thermoregulatory function and acquired heat tolerance of β-amyloid (Aβ)-infused rats. Male Wistar rats were anesthetized and implanted in the intraperitoneal cavity with a temperature transmitter. Aβ peptide (4.9–5.5 nmol) was dissolved in a solvent of 35% acetonitrile and 0.1% trifluoroacetic acid (pH 2.0). The solvent was used as the vehicle. An osmotic pump contained 234 ± 13.9 μl of Aβ solution was subcutaneously implanted in the back and was cannulated into the left cerebral ventricle. Moreover, 0.5 µg of AlCl3 was injected into the right cerebral ventricle with a micro syringe pump (Aβ-infused rats). The solvent-infused rats were used as control rats (CN rats). After 2 weeks, rats were placed in a thermal gradient and their intra-abdominal temperature (Tab) and their ambient temperatures (Ta) selected (Ts) were measured for 3 consecutive days. In an additional study, rats were kept at a Ta of 32°C for 4 weeks to attain heat acclimation. Then, rats were subjected to a heat tolerance test, i.e. they were exposed to a Ta of 36°C for 160 min. Although there were clear day-night variations of Ts and Tab in CN rats, patterns were significantly abolished in Aβ-infused rats. Moreover, heat tolerance obtained by heat acclimation was attenuated in Aβ-infused rats. These results suggest that Aβ-infusion in the lateral ventricle modifies behavioral thermoregulation and lowers an ability to acclimate to heat in rats.
Abbreviations
AD | = | Alzheimer disease |
Aβ | = | β-amyloid |
Ta | = | ambient temperature |
Tab | = | abdominal temperature |
Ts | = | selected ambient temperature |
i.c.v. | = | intracerebroventricular |
PO/AH | = | preoptic area/anterior hypothalamus |
Dcx | = | doublecortin |
PCNA | = | proliferating cell nuclear antigen. |
Introduction
Alzheimer disease (AD) is a progressive neurodegenerative disorder characterized by cognitive and memory deterioration and a major social and clinical burden in the elderly. There is accumulating evidence that the production and deposition of β-amyloid (Aβ) is central to the pathogenesis of AD (Selkoe Citation1991, Hardy, Selkoe Citation2002). Aβ has been shown to cause synaptic dysfunction and neuronal cell death by some mechanisms, e.g. increased oxidative stress, disruption of cellular calcium homeostasis and induces neuronal cell death (Mattson, 1997). Aβ also suppresses neuronal progenitor cell proliferation and differentiation in the hippocampus and/or cerebral cortex that may contribute to depletion of neurons and cognitive impairment in AD (Gibson et al., Citation2001, Haughey et al., Citation2002). Moreover, patients with AD suffer from non-cognitive behavioral symptoms, such as depression, anxiety, agitation, hyperactivity and disturbed circadian rhythms and sleep (Assal, Cummings Citation2002; Gillette-Guyonnet et al., Citation2007; Bombois et al., Citation2010; Klaffke, Staedt Citation2006; Stoppe, Brandt, Staedt Citation1999; Finkel Citation2003). Abnormalities have been seen in accordance with a decrease of thermal sensation in AD patients (Gibson et al., Citation2001, Iwamoto, Hanyu, Umahara Citation2013). Decline of thermal sensation increases the risk of burn and/or frostbite. Attenuation of thermal sensation and cognitive function in AD patients are expected to affect behavioral thermoregulatory functions. Because autonomic thermoregulatory functions in the elderly are attenuated (Armstrong, Kenney, Citation1993, Minson et al., 2002, Okawa et al., Citation1991), deficient of behavioral thermoregulatory functions induce several incidents such as hypothermia and/or heat stroke. However, those of thermoregulatory functions in AD have not been proven experimentally.
On the other hand, heat acclimation has been attracting attention as prevention of heat stroke. For animals, constant exposure to moderate heat has been well known to result in development of heat acclimation (Wyndham et al., Citation1976). Heat-acclimated animals show reinforced heat dissipation, reduced heat production and enhanced heat tolerance for acute heating (Wyndham et al., Citation1976, Garrett et al., Citation2009, Sawka et al., Citation2011). We have previously reported that hypothalamic progenitor cell proliferation and neural differentiation, namely neurogenesis, are enhanced in heat-acclimated rats (Matsuzaki et al., Citation2009). Moreover, heat exposure-induced hypothalamic neurogenesis and acquired heat tolerance in rats were interfered with aging (Matsuzaki et al., 2014). Thus, neurogenesis and associated reconstructions of neuronal networks in the hypothalamic area might have a pivotal role in modulating heat acclimation. However, a capacity of acquired heat tolerance by heat acclimation in AD has not been sufficiently examined.
In this study, we investigated the effect of intracelebroventricular (i.c.v.) Aβ infusion on behavioral thermoregulation of rats by measuring their selected ambient temperatures (Ts). Also, a capacity of acquired heat tolerance in Aβ-infused rats was analyzed.
Materials and Methods
Procedure of Aβ infusion
Aβ infusion were carried out as described previously (Nitta et al., 1991, Hashimoto et al., Citation2002, Matsuzaki et al., 2005, Haque et al., Citation2008, Hashimoto et al., Citation2011). Twelve male Wistar rats (Japan SLC) of 10 weeks old were individually housed in transparent plastic cages (width, 270 mm; length, 440 mm; height, 187 mm) with wood chippings and initially maintained at an ambient temperature (Ta) of 24.0 ± 0.1°C and relative humidity of 45 ± 5% under a 12:12-h light–dark cycle. Rats were anesthetized with pentobarbital sodium (50 mg/kg, i.p.) and a temperature transmitter (TA10TA-F40; Data Sciences) was implanted in their intraperitoneal cavity. Aβ1–40 (Peptide institute) was dissolved in a solvent of 35% acetonitrile plus 0.1% trifluoroacetic acid (pH 2.0). The solvent was used as the vehicle. As a small amount of AlCl3 has been documented to facilitate aggregation of Aβ in vitro (Kawahara et al., Citation1994), 0.5 μg of AlCl3 was injected into the right cerebral ventricle by using a micro syringe pump. This procedure strongly improved reproducibility and reliability in yielding an animal model of AD with impaired memory (Nitta et al., 1991, Hashimoto et al., Citation2002, Matsuzaki et al., 2005, Haque et al., Citation2008). The osmotic pump contained 234 ± 13.9 μl of Aβ solution. A total amount of 5.5 nmol Aβ was infused at the infusion rate of 0.56 μl/h. The rats were allowed to recover from surgery for at least 12 d prior to data collection (Aβ-infused rats). Vehicle infused rats were used as control rats (CN rats). Vehicle infusion has not affect cognitive function and locomotors activity in rats. All rats were housed individually to avoid crosstalk of radio telemetry.
Estimation of behavioral thermoregulation
Behavioral thermoregulation of Aβ-infused rats were estimated their selected ambient temperatures (Ts) as described previously (Sugimoto, Sakurada, Shido Citation1999). Briefly, a long wire mesh cage (200 × 12 × 12 cm) located in an outer temperature gradient box constructed from 0.78-cm-thick aluminum and dimensions of 210 × 15 × 15 cm. Eighteen position sensors activated by an infrared light source (PZ-41, KEYENCE) were located along the length of the temperature gradient at approximately 10-cm intervals. The ends of the outer gradient box were maintained at 15 and 37°C with water perfusion devices, which resulted in air temperatures inside the box ranging from approximately 17 to 36°C. AM receivers (RLA3000, Data Sciences) were placed on the temperature gradient box. Food was placed on the floor of the internal cage and water was provided through 4 holes made on the ceiling of the temperature gradient box. Then, the rats could have food at their Ts and drink water at a temperature close to their Ts. The light control was maintained throughout the experiments. The location of the rats in the thermal gradient was detected by the position sensors and Ts was estimated by the calibration curve of air temperature as a function of the location. Measurements started at 16:00 hour and continued for following 3 d Tab and Ts data were sampled every minute with a computer logging system. Spontaneous activity levels of the rats were estimated according to the number of times that the rats crossed the infrared beam emitted from the position sensors in a minute.
Heat tolerance test
Heat tolerance test was carried out as described previously (Matsuzaki et al., 2014). After Ts measurements, CN and Aβ-infused rats were subjected to a 160-min heat exposure that was increased by 0.7–0.8°C every 10 min from 24–36°C, as shown in . When the rats were subjected to the heat, Tab of the rats in each group was measured by telemetry system (Dataquest; Data Sciences). Food and water were removed during the test.
Figure 1. The mean Tab in CN and Aβ–infused rats. (A) Before surgery, Tab levels of CN (black circle) did not differ from those of Aβ-infused rats (red circle). (B) Twelve days after surgery, Tab of the light phase in Aβ-infused rats was significantly higher than that of CN. Dark bars above abscissa indicate the dark phase of the day. Values are the means ±S.E.Ms (n = 6 in each group).
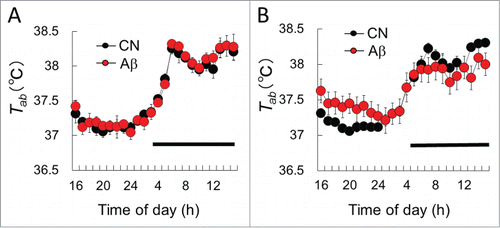
Figure 2. The mean Ts in CN and Aβ–infused rats. (A) Ts value of day in CN (black circle) and Aβ-infused rats (red circle). Dark bar above abscissa indicates the dark phase of the day. (B) Mean Ts of CN (black bar) and Aβ-infused rats (red bar) in the light and dark phase. In the light phase, mean Ts of Aβ-infused rats was significantly lower than that of CN. However, in the dark phase, mean Ts of Aβ-infused rats was significantly higher than that of CN. Values are the means±S.E.Ms (n = 6 in each group). *Shows significant difference of the light phase and dark phase. # shows significant difference between CN and Aβ-infused rats.
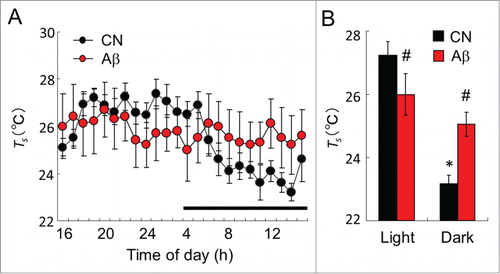
Figure 3. Heat tolerance test in CN and Aβ–infused rats. (A) Ta of the chamber in the heat tolerance test. Ta was gradually raised from time at 0 min. (B) Tab responses to gradient heat of CN (black circle) and Aβ-infused rats (red circle). The Tab values of all rats were monitored by a telemetry system. Tab responses of CN and Aβ-infused rats did not significantly change. (C) c-Fos+ cells in the PO/AH. Green signals show c-Fos+ cells. The expression level of c-Fos+ cells of CN (left) and Aβ-infused rats (right) did not alter. Scale bar: 50 μm. (D) Over view of PO/AH of hypothalamic section. Red box shows immuno-stained area of (C). ac, anterior comm. ox, optic chiasm.
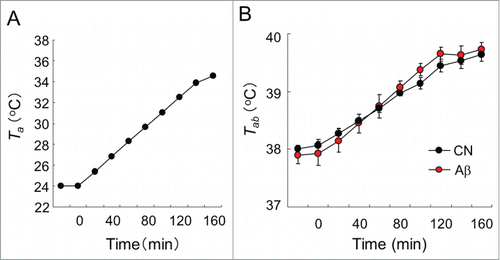
Immunohistochemistry
After the heat tolerance test, pentobarbital sodium (50 mg/kg, i.p.) was used to anesthetize the rats and was transcardially perfused with ice cold 4% Paraformaldehyde phosphate buffer solution (Wako, Tokyo, Japan) preceded by a saline perfusion. The brain was removed, fixed overnight at 4°C in 4% formaldehyde phosphate buffer solution, and immersed in 20% (w/v) sucrose solution. A cryostat was used to prepare brain sections (40-µm thickness), which were collected as free-floating sections, of all the rats. The brain sections were incubated in 50% formamide/2× standard sodium citrate for 2 h at 65°C, incubated in 2 N HCl for 30 min at 37°C, rinsed in 100 mM boric acid (pH 8.5) for 10 min at 25°C, and washed with 0.25% Triton X-100 in Tris-buffered saline (pH 7.4). For multiplex immunoassaying, coronal sections were incubated with several primary antibodies for 12 h at 4°C. The primary antibodies used in this study were polyclonal rabbit anti c-Fos IgG (1:500; SantaCluz), polyclonal rabbit anti-doublecortin (Dcx) IgG (1:200; Abcam), monoclonal mouse anti-proliferating cell nuclear antigen (PCNA) IgG (1:200; Cell Signaling). To identify c-Fos-immunopositive (c-Fos+) cells, PCNA-immunopositive (PCNA+) cells and Dcx immunopositive (Dcx+) cells, Alexa Fluor 488 anti-rabbit IgG and Alexa Fluor 488 anti-mouse IgG (1:500; Molecular Probes) were used as the secondary antibodies. After staining, brain sections were mounted on glass slides and covered with 80% glycerol. A confocal microscope (FV-1000; Olympus) and imaging software (Olympus Fluoview; Olympus) were used to visualize all sections under 20× or 40× magnifications. For the hypothalamic area, brain sections (between −0.26 and −4.80 mm from the bregma) were obtained according to the Paxinos and Watson atlas (Paxinos, Watson Citation1998).
Additional experiment; measurements of acquired heat tolerance
Additionally, we have confirmed whether Aβ-infused rats can improve heat tolerance by long-term heat acclimation. Eight male Wistar rats were used for this additional experiment. After recovery period, all rats were subjected to a constant Ta of 32.0 ± 0.2°C and relative humidity of 40 ± 10%. On the 40th day after the start of the heat exposure period, all rats were subjected to a heat tolerance test. Then, brains were sampled and used for immunohistochemistry and/or Western blot analysis.
Western blot analysis
Hypothalamic section were extracted with lysis buffer including 1 mM EDTA, 1% SDS, 1 × Complete protease inhibitor cocktail (Roche Diagnostics) and 10 mM HEPES (pH 7.5). Lysate was boiled at 95°C for 10 min, sonicated and centrifuged at 14,000 r.p.m for 20 min at 4°C to obtain the supernatant as cell extract. The lysates were separated by 12.5% SDS-PAGE and transferred onto membrans. The membrans were incubated with polyclonal rabbit anti-Dcx antibody (1:1000). HRP-conjugated anti rabbit IgG (1:2000, Cell Signaling) was used as secondary antibody. Immunoblots were incubated with ECL detection kit (Amersham ECL Prime, GE Health Care) and were visualized by image analyzer (LAS-4000, FUJI FILM). Then the membrane were stripped and reprobed with monoclonal rabbit anti-GAPDH antibody (1:1000; Cell Signaling).
Data quantification and statistical analysis
The results are presented as the mean ± SEM. The parameters obtained were analyzed by 2-way analysis of variance (ANOVA) with Tukey's post hoc test and/or Student's T-test. Statistical analyses were performed with SPSS (version 18.0, IBM) software. A P value <0.05 was considered to indicate statistical significance.
Results
The mean Tab values of CN and Aβ-infused rats were measured by telemetry system. Before surgery, Tab levels of CN rats did not differ from those of Aβ-infused rats (). Eleven days after surgery, Tab of the light phase in Aβ-infused rats was significantly higher than that of CN (, F(1,24) = 14.2, P < 0.01, 2-way ANOVA with Tukey's post hoc test). Tab of Aβ-infused rats in the dark phase was slightly lower than that of CN.
Ts of Aβ-infused rats
Two weeks after surgery, Ts value of CN and Aβ-infused rats were analyzed by the thermal gradient chamber. Although there were clear day-night variations of Ts in CN rats, those of variations were abolished in Aβ-infused rats (). Mean Ts of CN rats in the light phase was significantly higher than that of the dark phase (, P < 0.01). In the light phase, mean Ts of Aβ-infused rats was significantly lower than that of CN rats (, P<0.05). However, in the dark phase, mean Ts of Aβ-infused rats was significantly higher than that of CN (, P < 0.01).
Heat tolerance of Aβ-infused rats without heat acclimation
During heat tolerance test, Ta was raised from 24°C to 36°C as shown in . The mean Tab values of 2 groups during the heat tolerance test are shown in . Both CN and Aβ-infused rats, Tab gradually increased with time. The increases in Tab in CN and Aβ-infused rats did not significantly differ ().
c-Fos+ cells in the hypothalamus of Aβ-infused rats
After heat tolerance test, brain section of the preoptic area/anterior hypothalamus (PO/AH) in the hypothalamus, a center of autonomic thermoregulation, were stained with anti-c-Fos antibody to determine neural activation. Although much number of c-Fos+ cells were detected in PO/AH, the expression level and distribution of c-Fos+ cells did not alter between CN and Aβ-infused rats ().
Additional experiment; Improvement of heat tolerance of Aβ-infused rats
Additionally, Aβ-infused rats (n=4) were newly made and loaded to 32°C for 40 d to improve heat tolerance. After the heat exposure period, rats were subjected to acute heat as described previously. The mean Tab values of 2 groups during the heat load are shown in . Before heat load, the Tab values of the CN and Aβ-infused rats did not differ. However, the increase in Tab values during 100–160 min after commencing heat load of Aβ-infused rats was significantly higher than that of CN rats (, F(1,20) = 21.3, P < 0.01, 2-way ANOVA with Tukey's post hoc test). The Tab difference between CN and Aβ-infused rats at 160 min after starting heat load was 0.56°C.
Figure 4. Ability of acquired heat tolerance in CN and Aβ-infused rats. (A) Heat tolerance test in CN (black circle) and Aβ–infused rats (red circle) after long term heat exposure. The increase in the Tab values was slower for CN rats than that for the Aβ-infused rats. #, significant difference between CN and Aβ-infused rats. Values are the mean ±SEM (n = 4). (B) PCNA and (C) Dcx immunostaining in the hypothalamus. Scale bar, 100 µm. (D) Western blot analysis of Dcx protein in the hypothalamus. (Left) Western blot analyses were performed using anti-Dcx antibody. Blots were then stripped and reprobed with anti-GAPDH antibody to verify that equal amount of protein was electrophoresed in each lane. (Right) The densitometric data on Dcx/GAPDH of CN (black bar) and Aβ-infused rats (red bar). # shows significant difference between CN and Aβ-infused rats. Values are the mean ±SEM (n = 4).
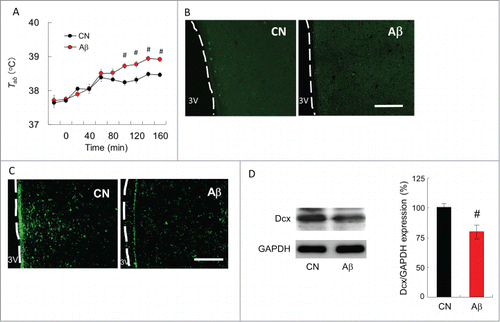
PCNA+ and Dcx+ cells in the hypothalamus after heat acclimation
Rats were anesthetized and brains were sampled for immunohistochemistry. The hypothalamic section were immuno-labeled with an anti-PCNA antibody, which labels nuclei of proliferating neuronal progenitor cells, and/or an anti-Dcx antibody, which labels soma of migrating immature neuron. In CN rats, PCNA+ cells and Dcx+ cells were broadly expressed in PO/AH. These cells in Aβ-infused rats, however, were hardly detected in PO/AH (). Western blot analysis indicated that the expression level of Dcx protein in the hypothalamus is significantly decreased by Aβ infusion ()
Discussion
In this study, we measured Ts variation of Aβ-infused rats by the thermal gradient system. The thermal gradient system is well known to be one of the ideal methods for studying long-term changes in behavioral thermoregulation, because rats can select an optimal ambient temperature for regulating body core temperature simply by moving to a different location (Laughter, Blatteis Citation1985). Also, animals have mechanisms that allow them to optimize their energy expenditure for thermoregulation by selecting a thermal environment that corresponds to the lowest metabolic oxygen requirements (Gordon, Citation1994). Therefore selected ambient temperature measured by the thermal gradient system is capable to one of the marker for behavioral thermoregulation. Chronic infusion of Aβ into lateral ventricle attenuated Ts variation in rats (). This result suggest that Aβ suppresses behavioral thermoregulatory function in rats. Compared with the knowledge about autonomic thermoregulation, central mechanism of behavioral thermoregulation is not fully examined. Although central mechanism of behavioral thermoregulation is hardly identified, the neural pathway from skin thermoreceptors to the cerebral cortex for somatic thermal sensation has been well investigated (Craig et al., Citation2000). Neurons responding non-noxious thermal stimuli applied to the skin are located in the lamina I of the spinal cord (Han, Zhang, Craig Citation1998). Signals from these neurons reach mainly the posterior part of the ventral medial nucleus in the thalamus. Previous studies using functional magnetic resonance imaging (fMRI) have shown that these signals reach several areas in the cerebral cortex, i.e., the insula, primary and secondary somatosensory, orbitofrontal, and cingulate cortex (Becerra et al., Citation1999, Craig et al., 1994, Davis et al., 1998). Although specific role of cerebral cortex for behavioral thermoregulation are unclear, cerebral cortex is attributable to behavioral thermoregulation. In this study, Aβ has been chronically injected into rats' lateral ventricle by using osmotic pump. In this animal model, much Aβ plaque have been seen in the cerebral cortex and hippocampus whereas Aβ deposits are hardly detected in hypothalamic parenchyma (Nabeshima, Nitta Citation1994, Hashimoto et al., Citation2011). This deposition patterns of Aβ is very similar to AD patients. Indeed, our western blot analysis indicated that the expression level of PSD-95, a postsynaptic neuronal marker, in the cerebral cortex is significantly reduced in Aβ-infused rats whereas this protein expression in the hypothalamus was hardly changed (data not shown). Probably, this distributional changes of Aβ and a synaptic marker reduction in cerebral cortex may be related to disruption of behavioral thermoregulatory functions. On the other hand, the magnitude of increase in Tab during heat tolerance test did not differ between CN and Aβ-infused rats (). Also, c-Fos protein expression, a neural activation marker, in PO/AH after 160 min heat stimulation did not alter (). It is now widely accepted that PO/AH plays a key role in autonomic thermoregulation in the heat. Animals with lesions of the PO/AH have reduced ability of autonomic thermoregulation; however, they can conduct behavioral thermoregulation (Carlisle, Citation1969), indicating that PO/AH is not so important for the behavioral processes. Aβ infusion into cerebral cortex may hardly affects autonomic thermoregulatory functions in young rats.
Aβ infusion into lateral ventricle altered Tab of the light phase in rats (). In contrast, the mean Ts in the light phase of the Aβ-infused rats was significantly lower than that of CN, whereas the mean Ts in the dark phase was raised by Aβ infusion (). Although exact reason for this relationship between changes of Tab and Ts is unknown, disruption of variance in day-night locomotor activity in Aβ-infused rats may be associated. Indeed, there was day-night variance of locomotor activity in CN rats, while those of variance in Aβ-infused rats was disappeared (data not shown). This result may be involved that sleep-wake cycle of Aβ-infused rats was impaired. In AD patients, disturbances in the sleep-wake cycle and circadian rhythms are typical symptoms. Most notably, a fragmented sleep-wake pattern characterized by decreased daytime activity and disrupted nighttime sleep is a common complaint in AD patients (Bonanni et al., Citation2005). Both the frequency and duration of nocturnal awakenings and daytime naps can influence an abnormal variability of Tab and behavioral thermoregulation. Although the sleep-wake cycle of Aβ-infused rats has not been measured in the present study, disruption of variance in day-night locomotor activity of Aβ-infused rats may also be associated with the changes of Tab and Ts.
We additionally investigated an ability of heat acclimation in Aβ-infused rats. Acquired heat tolerance obtained by 40-day heat exposure in Aβ-infused rats were significantly lower than that of CN rats (). This result suggest that Aβ impairs an ability of heat acclimation in rats. As a prediction, attenuation of acquired heat tolerance may be at least partially related to the changes in the expression levels of PCNA and Dcx proteins in the hypothalamus. Previously, we have reported that progenitor cell proliferation and neural differentiation are enhanced in the hypothalamus of heat-acclimated rats (Matsuzaki et al., Citation2009). Also, aging attenuates the ability of heat tolerance and acquired heat tolerance in rats (Matsuzaki et al., 2014). Moreover, inhibition of hypothalamic neurogenesis by mitosis inhibitor attenuates acquired heat tolerance in rats (Unpublished observation). These observations suggest that neurogenesis in the hypothalamus may be important to attain heat acclimation in rats. On the other hand, PCNA protein is originally identified by immunofluorescence as a nuclear protein whose appearance correlated with the proliferative state of the cell (Bravo Citation1986). Dcx is typically associated with the migration of newborn neurons during the development of the central nervous system (Brown et al., 2003). The protein expression levels of PCNA and Dcx are kept high during the development of newborn neurons within certain areas of the adult mammalian brain. In this study, the expression levels of PCNA and Dcx in the hypothalamus was reduced by Aβ infusion (). In adult rat hypothalamus, neuronal progenitor cells exist in the ependymal layer of the third ventricle and they migrate into the hypothalamic parenchyma where they differentiate into neurons (Markakis et al., Citation2004, Xu et al., Citation2005). Although Aβ was injected into lateral ventricle, Aβ may have reached third ventricle via interventricular foramen and impaired the functions of progenitor cell proliferation and neural differentiation. In human AD patients, Aβ concentration in the cerebrospinal fluid is increased. Because soluble Aβ has high toxicity for central nervous system, neurogenesis in the hypothalamus of AD patients may be attenuated.
In this study, we have investigated 2 things. At first, we proved that Aβ infusion into lateral ventricle alters Ts variation in rats. Because autonomic thermoregulatory functions in the elderly are declined, the increase in the rate of susceptible to heat stroke in AD patients may partly depend on the abnormalities of behavioral thermoregulation. It may be necessary to experiment with Aβ-infused aged rats in a future. At second, we have demonstrated that Aβ attenuates an ability of heat acclimation in rats. One possible reason for the attenuation of acquired heat tolerance may be that hypothalamic progenitor cells proliferation and neural differentiation are inhibited by Aβ. However, heat tolerance of Aβ-infused rats was slightly improved, because Tab of heat-exposed Aβ-infused rats after 160 min heat load was slightly lower than that of non-acclimated Aβ-infused rats (). Although Aβ attenuates acquired heat tolerance, heat acclimation may be useful to prevent heat stroke for the patients of AD.
Disclosure of Potential Conflicts of Interest
No potential conflicts of interest were disclosed.
Funding
This study was supported by a Ministry of Education, Culture, Sports, Science, and Technology of Japan Grant-in-Aid for Scientific Research (C) 24590300.
References
- Armstrong CG, Kenney WL. Effects of age and acclimation on responses to passive heat exposure. J Appl Physiol 1993; 75:2162-7; PMID:8307874
- Assal F, Cummings, JL. Neuropsychiatric symptoms in the dementias. Curr Opin Neurol 2002; 15:445-50; PMID:12151841; http://dx.doi.org/10.1097/00019052-200208000-00007
- Becerra LR, Breiter HC, Stojanovic M, Fishman S, Edwards A, Comite AR, Gonzalez RG, Borsook D. Human brain activation under controlled thermal stimulation and habituation to noxious heat: an fMRI study. Magn Reson Med 1999; 41, 1044-57; PMID:10332889; http://dx.doi.org/10.1002/(SICI)1522-2594(199905)41:5%3c1044::AID-MRM25%3e3.0.CO;2-M
- Bombois S, Derambure, P, Pasquier F, Monaca C. Sleep disorders in aging and dementia. J Nutr Health Aging 2010; 14:212-7; PMID:20191256; http://dx.doi.org/10.1007/s12603-010-0052-7
- Bonanni E, Maestri M, Tognoni G, Fabbrini M, Nucciarone B, Manca ML, Gori S, Iudice A, Murri L. Daytime sleepiness in mild and moderate Alzheimer's disease and its relationship with cognitive impairment. J Sleep Res, 2005; 14:311-7; PMID:16120107; http://dx.doi.org/10.1111/j.1365-2869.2005.00462.x
- Bravo R, Synthesis of the nuclear protein cyclin (PCNA) and its relationship with DNA replication. Exp Cell Res 1986; 163:287-93; PMID:2869964; http://dx.doi.org/10.1016/0014-4827(86)90059-5
- Carlisle H. Behavioral significance of hypothalamic temperature-sensitive cells. Nature 1966; 209:1324-5; PMID:5956045; http://dx.doi.org/10.1038/2091324a0
- Carlisle H. The effects of preoptic and anterior hypothalamic lesions on behavioral thermoregulation in the cold. J Comp Physiol Psychol 1969; 69:391-402; PMID:5404472; http://dx.doi.org/10.1037/h0028170
- Craig AD, Chen K, Bandy D, Reiman EM. Thermosensory activation of insular cortex. Nature Neurosci 2000; 3:184-190; PMID:10649575; http://dx.doi.org/10.1038/72131
- Finkel SI. Behavioral and psychologic symptoms of dementia. Clin Geriatr Med 2003; 19(4):799-824; PMID:15024813; http://dx.doi.org/10.1016/S0749-0690(03)00046-6
- Garrett AT, Goosens NG, Rehrer NJ, Patterson MJ, Cotter JD. Induction and decay of short-term heat acclimation. Eur J Appl Physiol 2009; 107(6): 659-70; PMID:19727796; http://dx.doi.org/10.1007/s00421-009-1182-7
- Gordon CJ. 24-Hour control of body temperature in rats I. Integration of behavioral and autonomic effectors. Am. J. Physiol. 1994; 267(36):71-7
- Han ZS, Zhang ET, Craig AD. Nociceptive and thermoreceptive lamina I neurons are anatomically distinct. Nat Neurosci 1998; 1:218-25; PMID:10195146; http://dx.doi.org/10.1038/665
- Haque AM, Hashimoto M, Katakura M, Hara Y, Shido O. Green tea catechins prevent cognitive deficits caused by Abeta1-40 in rats. J Nutr Biochem 2008; 19(9):619-26; PMID:18280729; http://dx.doi.org/10.1016/j.jnutbio.2007.08.008
- Gibson SJ, Voukelatos X, Ames D, Flicker L, Helme RD. An examination of pain perception and cerebral event-related potentials following carbon dioxide laser stimulation in patients with Alzheimer's disease and age-matched control volunteers. Pain Res Manag. 2001 6(3):126-32; PMID:11854775
- Gillette-Guyonnet S, Abellan Van Kan G, Alix E, Andrieu S, Belmin J, Berrut G, Bonnefoy M, Brocker P, Constans T, Ferry M. IANA (International Academy on Nutrition and Aging) Expert Group: weight loss and Alzheimer's disease. J. Nutr. Health Aging 2007; 11:38-48; PMID:17315079
- Hashimoto M, Hossain S, Shimada T, Sugioka K, Yamasaki H, Fujii Y, Ishibashi Y, Oka JI, Shido O. Docosahexaenoic acid provides protection from impairment of learning ability in Alzheimer's disease model rats J Neurochem 2002; 81:1084-91; PMID:12065621; http://dx.doi.org/10.1046/j.1471-4159.2002.00905.x
- Hashimoto M, Tozawa R, Katakura M, Shahdat H, Haque AM, Tanabe Y, Gamoh S, Shido O. Protective effects of prescription n-3 fatty acids against impairment of spatial cognitive learning ability in amyloid β-infused rats. Food Funct. 2011; 2(7): 386-94; PMID:21894325; http://dx.doi.org/10.1039/c1fo00002k
- Hashimoto M. Animal models of Alzheimer's disease infused with amyloid-beta peptide into the cerebral ventricle. Nihon Rinsho 2011; 8:241-6; PMID:22787789
- Hardy J, Selkoe DJ. The amyloid hypothesis of Alzheimer's disease: progress and problems on the road to therapeutics. Science 2002; 297:353-6; PMID:12130773; http://dx.doi.org/10.1126/science.1072994
- Haughey NJ, Liu D, Nath A, Borchard AC, Mattson MP. Disruption of neurogenesis in the subventricular zone of adult mice, and in human cortical neuronal precursor cells in culture, by amyloid beta-peptide: implications for the pathogenesis of Alzheimer's disease. Neuromolecular Med 2002; 1(2):125-35; PMID:12025858; http://dx.doi.org/10.1385/NMM:1:2:125
- Haughey NJ, Nath A, Chan SL, Borchard AC, Rao M.S, Mattson MP. Disruption of neurogenesis by amyloid beta-peptide, and perturbed neural progenitor cell homeostasis, in models of Alzheimer's disease. J Neurochem. 2002; 83(6):1509-24 ; PMID:12472904; http://dx.doi.org/10.1046/j.1471-4159.2002.01267.x
- Iwamoto T, Hanyu H, Umahara T. Age-related changes of sensory system. Nihon Rinsho 2013; 71(10):1720-5; PMID:24261198
- Kawahara M, Muramoto K, Kobayashi K, Mori H, Kuroda Y. Aluminium promotes the aggregation of Alzheimer's amyloid β-protein in vitro. Biochem Biophys Res Commun 1994; 198:531-5; PMID:7507666; http://dx.doi.org/10.1006/bbrc.1994.1078
- Klaffke S, Staedt J. Sun downing and circadian rhythm disorders in dementia. Acta Neurol. Belg. 2006; 106:168-75; PMID:17323834
- Laughter JS Jr, Blatteis CM. A system for the study of behavioral thermoregulation of small animals. Physiol. Behav. 1985; 35:993-7; PMID:4095193
- Markakis EA, Palmer TD, Randolph-Moore L, Rakic P, Gage FH. Novel neuronal phenotypes from neural progenitor cells. J Neurosci 2004; 24:2886-97; PMID:15044527; http://dx.doi.org/10.1523/JNEUROSCI.4161-03.2004
- Matsuzaki K, Yamakuni T, Hashimoto M, Haque AM, Shido O, Mimaki Y, Sashida Y, Ohizumi Y. Nobiletin restoring beta-amyloid-impaired CREB phosphorylation rescues memory deterioration in Alzheimer's disease model rats. Neurosci Lett 2006; 400(3):230-4; PMID:16581185; http://dx.doi.org/10.1016/j.neulet.2006.02.077
- Matsuzaki K, Katakura M, Hara T, Li G, Hashimoto M, Shido O. Proliferation of neuronal progenitor cells and neuronal differentiation in the hypothalamus are enhanced in heat-acclimated rats. Pflugers Arch 2009; 458:661-73; PMID:19252922; http://dx.doi.org/10.1007/s00424-009-0654-2
- Matsuzaki K, Katakura M, Inoue T, Hara T, Hashimoto M, Shido O. Aging attenuates acquired heat tolerance and hypothalamic neurogenesis in rats. J Comp Neurol. 2015; 523(8):1190-201; PMID:25556765; http://dx.doi.org/10.1002/cne.23732
- Minson CT, Wladkowski SL, Cardell AF, Pawelczyk JA, Kenney WL. Age alters the cardiovascular response to direct passive heating. J Appl Physiol. 1998; 84:1323-32; PMID:9516200
- Nabeshima T, Nitta A. Memory impairment and neuronal dysfunction induced by beta-amyloid protein in rats. Tohoku J Exp Med. 1994; 174(3):241-9; PMID:7761989; http://dx.doi.org/10.1620/tjem.174.241
- Nitta A, Itoh A, Hasegawa T, Nabeshima T. beta-Amyloid protein-induced Alzheimer's disease animal model. Neurosci Lett. 1994; 17:63-6; PMID:8086012; http://dx.doi.org/10.1016/0304-3940(94)90239-9
- Okawa M, Mishima K, Hishikawa Y, Hozumi S, Hori H, Takahashi K. Circadian rhythm disorders in sleep-waking and body temperature in elderly patients with dementia and their treatment. Sleep. 1991; 14(6): 478-85; PMID:1798879
- Paxinos, G., Watson, C., 1998. The rat brain in stereotaxic coordinates, fourth ed. Academic Press
- Sawka M, Leon LR, Montain SJ, Sonna LA. Integrated physiological mechanisms of exercise performance, adaptation, and maladaptation to heat stress. Comp Physiol 2011; 1:1883-928; PMID:23733692
- Selkoe DJ. The molecular pathology of Alzheimer's disease. Neuron 1991; 6:487-98; PMID:1673054; http://dx.doi.org/10.1016/0896-6273(91)90052-2
- Stoppe G, Brandt CA, Staedt JH. Behavioural problems associated with dementia: the role of newer antipsychotics. Drugs Aging. 1999;14(1):41-54; PMID:10069407; http://dx.doi.org/10.2165/00002512-199914010-00003
- Sugimoto N, Sakurada S, Shido O. Selected ambient temperatures of rats acclimated to heat given on various schedules. Pflugers Arch 1999; 438(6):766-70; PMID:10591063; http://dx.doi.org/10.1007/s004240051104
- Wyndham CH, Rogers GG, Senay LC, Mitchell D. Acclimization in a hot, humid environment: cardiovascular adjustments. J Appl Physiol 1976; 40:779-85; PMID:931906
- Xu Y, Tamamaki N, Noda T, Kimura K, Itokazu Y, Matsumoto N, Dezawa M, Ide C. Neurogenesis in the ependymal layer of the adult rat 3rd ventricle. Exp Neurol 2005; 192:251-64; PMID:15755543; http://dx.doi.org/10.1016/j.expneurol.2004.12.021