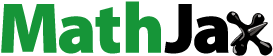
ABSTRACT
Occupational heat stress apart from adverse heat-related health consequences also induces DNA damage in workers exposed to high working temperatures. We investigated the association between chronic heat exposures and Micronuclei (MN) frequency in lymphocytes of 120 workers employed in the steel industry. There was a significant increase in the MN-frequency in exposed workers compared to the unexposed workers (X2 = 47.1; p < 0.0001). While exposed workers had higher risk of DNA damage (Adj. OR = 23.3, 95% CI 8.0–70.8) compared to the unexposed workers, among the exposed workers, the odds of DNA damage was much higher for the workers exposed to high-heat levels (Adj. OR = 81.4; 95% CI 21.3–310.1) even after adjusting for confounders. For exposed workers, years of exposure to heat also had a significant association with higher induction of MN (Adj. OR = 29.7; 95% CI 2.8–315.5). Exposures to chronic heat stress is a significant occupational health risk including damages in sub-cellular level, for workers. Developing protective interventions to reduce heat exposures is imperative in the rising temperature scenario to protect millions of workers across the globe.
Introduction
Globally, a rise in temperatures has paved the way for health threats for millions of people [Citation1,Citation2]. Excess heat exposures is not only an environmental threat but also an occupational hazard for a large worker population engaged in hard manual labor in tropical settings [Citation3] exposed to heat stress and strain [Citation4]. Workers in high-heat industries such as iron and steel, foundries, smelters, brick-firing and ceramic, glass and rubber, bakeries, commercial kitchens, and mining are already subjected to high heat exposures on a day-to-day basis and have high potential for heat-related illnesses like heat exhaustion, heat stroke, and death [Citation5–Citation9] which is likely to increase in the future climate change scenario [Citation10,Citation11].
According to the reports of global climate risk index [Citation12], India is classified under the most vulnerable regions exposed to extreme weather conditions with resulting huge economic loss due to heat-induced decreased health, work capacity, productivity consequences, and fatalities [Citation8,Citation13,Citation14]. In particular, the southern region is most influenced by climatic fluctuations [Citation15], has high-heat conditions for the most part of the year that largely influences the indoor workplace temperatures [Citation16] further worsened by heat generated from the processes with consequent undesirable health and productivity [Citation10,Citation17–Citation19].
Earlier reports have shown that heat stress not only inhibits DNA repair processes but can also act as a DNA damaging agent [Citation20,Citation21]. Some animal and human studies concluded that oxidative stress is the main factor responsible for DNA damage caused by heat stress [Citation20,Citation22]. The oxidative stress and resultant altered cellular redox environment within the cells cause protein degradation, DNA damage, cell death [Citation23], compromised sperm quality and an increased risk of infertility [Citation24,Citation25]. It was reported that workers exposed to high heat conditions had high levels of DNA damage and over-expression of HSP70 levels [Citation26,Citation27]. Rocket et al. [Citation28] showed that the expression of a number of DNA repair genes such Ogg1, XPG and Rad54 were all down-regulated when DNA microarrays were used to investigate the changes in global gene expression when exposed to heat stress at 43°C. Heat stress-induced cryptorchidism results in decreased expression of DNA polymerase b and DNA ligase III, both of which are involved in the final stages of DNA repair [Citation29].
A micronucleus (MN) is a small extra nucleus separated from the main nucleus formed by the condensation of acrocentric chromosomes or by the whole chromosome which has failed to incorporate into the daughter nuclei at the time of cell division [Citation30]. The MN assay has been employed to determine the genotoxic and mutagenic capacity of various chemical stressors/agents that could lead to the production of MN [Citation31]. The MN formation, a marker of genomic damage, was elevated in temperature-dependent studies conducted in human keratinocyte cell line in a statistically significant manner [Citation32,Citation33] and high temperatures induced MN formation in human lymphocytes that further enhanced when combined with other occupational risk factors [Citation26]. Kantidze et al deduced from their experimental studies that heat stress directly results in the formation of various DNA damage and double strand breaks depending on the cell type and level of the heat exposures [Citation11,Citation20,Citation24,Citation26,Citation33–Citation35].
MN is an early marker of DNA damage [Citation36] and measures the extent of chromosome breakage in early cell divisions [Citation37,Citation38], and unlike comet assay biomarker, which is indicative of current exposure over the previous few weeks, the MN assay detects fixed mutations that persist at least one mitotic cycle [Citation39]. MN frequency and MN in a cell is as an excellent biomarker to estimate the damage due to environmental stressors and carcinogenic agents that can induce genotoxicity [Citation36]. Though in the recent times there has been a rise in the number reports on various workplace exposures and DNA damage [Citation35,Citation40–Citation43], heat exposures which are a very common exposure in occupational settings across the globe is still under-addressed [Citation38].
With this background, this preliminary study was conducted as a follow-up of a larger study done by the authors [Citation17] to understand the association between chronic occupational heat exposures and DNA damage in worker population exposed to high-heat work environments in the steel industry in Southern India.
Results
Demographic variables
The general characteristics of the study population (both exposed and unexposed group) are given in . All workers who were identified to participate in the study were males and were permanent employees of the industry. The age of the workers ranged between 26–39 years and the prevalence pattern of both smoking (X2 = 1.52; p = 0.216) and consumption of alcohol (X2 = 0.002; p = 0.964) was not different between the exposed and unexposed workers. About a third of the workers had more than 10 years of experience/exposure in the industry.
Table 1. Distribution and comparison of workers’ characteristics of exposed and unexposed workers in the steel industry.
Heat stress, heat strain profiling and workers’ perception of heat and health
The WBGT ranged between 22.1°C – 41.7°C in the various work sections () and among the workers, nearly 63.3% had WBGT exposures above the Threshold Limit Value (TLV) of >27.5°C for workers with heavy workload due to direct heat exposures from furnaces and/or hot processes categorized as exposed group. 36.6% of workers were in the unexposed group with WBGT exposures < 27.5°C. Inside the industry, high process-generated radiant heat was prevalent in almost all areas, and results of the area WBGT measurements reflect the heat exposures of the 120 workers ().
Table 2. Distribution of heat exposures (WBGT°C) across various work sections in the steel industry (n = 120).
Physiological responses
Increased physiological strain as indicated by the rise in CBT >1°C, SwR> 1L/hr and USG > 1.020 was higher among exposed workers (93.4%) compared to the unexposed workers (84.1%). The measured physiological responses as Core Body Temperature (CBT), Sweat Rate (SR) and Urinary Specific Gravity (USG) in various work areas given in clearly showed that the heat strain symptoms were higher among the workers in work locations with very high chronic heat exposures compared to the unexposed workers.
Workers perception of heat and health
The results of the 120 workers’ self-reported affirmative response for any one of the heat-related symptoms such as excessive sweating or thirst, tiredness, cramps, headache, nausea/vomiting, fainting, prickly heat or urinogenital issues was 84.1% (n = 101).
DNA damage and MN frequency in lymphocytes of workers
The frequency of MN scored manually was reconfirmed in select samples using the automated cytogenetic imaging and the results obtained from both of the methods are presented in . The results of DNA damage assessed by MN frequency for the exposed and unexposed workers in the Bi-Nucleate cells (BN) are summarized in . The mean MN frequency in workers exposed to chronic high-heat conditions was much higher than the MN frequency encountered in the unexposed workers’ category which was significantly associated. The dependent variable was categorized as a dichotomic variable using a cut-off value corresponding to the baseline MN frequency of 0.07 MN/cell based on a previous study to find the risk [Citation44]. As can be seen from , the mean MN frequency encountered in workers exposed to chronic high-heat conditions was significantly higher than those encountered in the unexposed workers(X2 = 47.1; p < 0.0001).
Table 3. MN frequency scoring for 1000 cells done by manual scoring vs. automated methods for select samples in the steel industry.
Table 4. DNA damage in the blood lymphocytes in the heat exposed and unexposed workers in steel industry (n = 120).
Results of MN assay conducted in the blood lymphocytes of workers in both exposed and unexposed groups revealed that the exposed workers had higher odds of DNA damage compared to the unexposed workers after adjusting for potential confounders (Adj. OR = 23.3; 95% CI 8.0–70.8 p < 0.0001). Level of heat exposure was significantly associated with the extent of DNA damage and among the exposed workers, the workers group exposed to WBGT >30°C (high-heat exposed group) had 81-times higher odds of DNA damage compared to the worker group exposed to WBGTs of 27.5–30°C (medium-heat exposed group), even after adjusting for other potential confounders such as age, smoking, alcohol consumption, workload and other pre-existing health conditions that could be a confounder such as diabetes, hypertension or thyroid diseases. The relationship between WBGT & DNA damage between high-heat, medium-heat, and unexposed groups is represented in and .
Table 5. Logistic regression model of risk factors inducing DNA damage (MN frequency) in the study samples (N = 120).
Figure 2. Relationship between WBGT (°C) & DNA Damage in high heat, medium heat unexposed groups (n = 120).
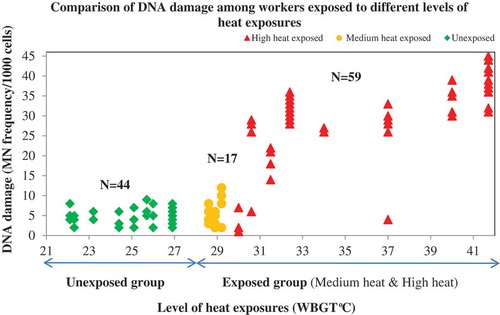
As is evident from , age and years of exposures in the industry had a significant positive association with DNA damage (p < 0.0001). The odds of DNA damage was significantly higher in workers under the age group < 30 years (Adj. OR = 12.6; 95% CI 2.9–55.9; p < 0.0001) compared to workers > 30 years after adjusting for key confounders such as smoking, alcohol and any pre-existing conditions such as diabetes, hypertension or thyroid diseases (Adj. OR = 16.5; 95% CI 3.9–70.3; p < 0.0001).
shows the relationship between years of exposure to heat and DNA damage that clearly shows that the exposed workers with >10 years of exposures had 70-times higher odds of DNA damage compared to the unexposed workers with same years of exposures in the same industry (95% CI 2.8–315.5; p < 0.0001). Statistical analysis conducted within the exposed group, workers with >10 years of heat exposures, had higher odds of DNA damage compared to workers with <10 years of heat exposures (OR = 15.5; 95% CI 4.6–52.7; p < 0.0001) after adjusting for potential confounders.
Figure 3. Relationship between years of exposure to heat and DNA damage (MN frequency) between the exposed and unexposed workers (n = 120).
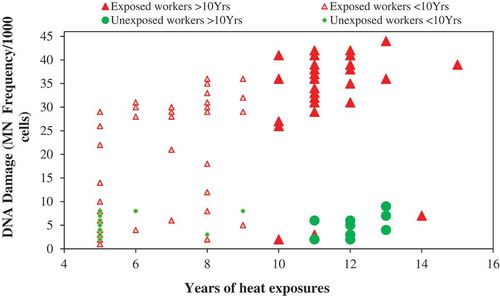
The images of the select exposed and unexposed samples with the MN frequency done manually () and the automated cytogenetic imaging using the Metafer systems () clearly show the distinction in the MN frequency encountered in the binucleate cells of exposed and unexposed workers.
Discussions
Heat stress & heat strain profiling and workers’ perception of heat and health
In the current changing climate scenario, heat exposures are predicted to be one of the major health burdens to human beings, especially in tropical settings with hot humid climate for the most part of the year [Citation45]. In addition to exposures to high environmental temperatures, people working in various industries are also exposed to excessive heat that induces additional health burden [Citation4]. Heat stress profiles prevailing in the workplace evaluated via area WBGT monitors in the industry showed high occupational heat stress exposures () that exceeded the recommended TLVs which has been observed in other studies conducted in India [Citation18,Citation46]. Workplaces that exceeded the recommended TLVs involved processes with high-heat exposure, like in the steel manufacturing industry and/or were outdoor jobs, like agriculture, construction, etc. [Citation17,Citation47], The measured WBGT in different sections of the steel industry ranged between 22.1°C – 41.7°C with average exposures of 33.5°C ± 3.2°C (). In the steel industry, most of the workers were exposed to high process-generated radiant heat that prevailed in almost all locations inside the production area, which is apparent from the average high globe temperature reading (53.2°C). The radiation in these enclosed areas was primarily from the processes often with minimal ventilation in these areas due to the nature of the production processes [Citation47]. Apart from the environmental heat imposed on the workers, high metabolic heat load is added for workers engaged in heavy physical work that involves intense arm and trunk work, carrying, pushing, and pulling heavy loads throughout their shifts, which categorizes them as being at a high risk as far as heat stress is concerned that could further be enhanced by continuous physical workload to complete production targets with less opportunity for self-pacing [Citation5,Citation48,Citation49].
The manifestation of excessive heat exposures are usually expressed by an individual’s physiological responses, viz., rise in CBT, SwR, and USG, and the relationship between physiological indicators of heat stress and associated adverse health risks are well established [Citation4]. Excessive sweating and tiredness/weakness was reported by 84.1% (n = 101) workers due to heat exposures and heavy workload [Citation17,Citation47]. The behavioral modifications by the workers in fluid intake and urinating pattern also influences the physiological indicators resulting in consequent adverse health concerns [Citation50]. Research has shown that excessive sweating and consequent dehydration increases heat strain [Citation51] and increases the risk of developing heat-related illness [Citation52,Citation53]. Heat and heavy workload subjects the workers to a higher risk of heat-related health illnesses (Crude OR = 1.9; 95% CI 1.4–2.5; p < 0.0001) which have been reported earlier in many studies across the globe [Citation7,Citation13,Citation17,Citation54].
Heat stress and DNA damage (micronuclei frequency)
The current study aimed to investigate the genotoxicity induced by exposures to high-heat stress work environments which were measured by the frequency of MN in the lymphocyte cells that have been reported earlier [Citation11,Citation20,Citation23,Citation34]. Study conducted by Xiao et al in workers’ exposed to coke oven heat and other pollutants induced DNA damage that was measured by MN frequency in blood lymphocytes [Citation26]. In our study, there was a significant positive association between exposures to heat and DNA damage that was significantly different between the heat exposed and un-exposed worker groups (X2= 47.1; p < 0.0001). The risk of DNA damage was 34-times higher in the exposed workers compared to the unexposed workers [Citation55] () and among the exposed workers, the “level of heat” had an impact on the extent of DNA damage which was apparent from the differences in MN frequency in medium-heat and high-heat exposed workers. Workers with high heat exposures (WBGT > 30°C) had an 81-times higher risk of DNA damage compared to the medium heat-exposed workers, even after adjusting for potential confounders (). This can be explained in the light of genomic instability that increases the number of mutations and/or chromosomal aberrations, which cytogenetically translate into increases in the number of chromosomal numbers and/or structure with formation of MN [Citation56]. Association between years of exposure to chronic heat and MN frequency was significant (p < 0.0001), similar to the observations of other studies [Citation20,Citation26,Citation28,Citation31]. The workers <30 years of age had a higher risk of DNA damage (OR = 38.3; 95% CI 2.9–55.9) compared to the workers in the age group >30 years (OR = 16.5; 95% CI 3.9–70.3), which did not concur with the theory that aging is associated with genomic instability and that higher incidence of MN manifests in older age groups [Citation57]. This data could not be explained by other factors such as smoking or alcohol associated with risk of higher frequency of MN as there was no significant difference between the smoker and non-smokers in the exposed and unexposed group, which has been a subject of controversy in many studies [Citation58]. The odds of DNA damage were lowered after adjusting for the confounders such as smoking, alcohol, intake of NSAIDs and years of exposure to heat (Adj. OR = 12.5; 95% CI 2.9–55.9). The only logical explanation would be the years of exposure to heat as a risk factor that increases the odds of DNA damage in the workers as all other confounders are not significantly different between the two age categories. It must be noted that the workers’ exposures to other occupational risks factors such as lack of welfare facilities, exposures to chemicals from manufacturing processes and other risk factors in the work settings are the same except for the “level of heat” and “metabolic workload” for both the unexposed and exposed groups.
The results are similar to another study in which the authors observed DNA damage and HSPs in workers exposed to benzene and heat stress in the paint industry [Citation59]. While numerous in-vitro studies have been performed regarding the effects of hyperthermia on DNA damage, the few experimental studies conducted in humans [Citation60] on the effect of hyperthermia on DNA damage [Citation61] and the release of HSPs [Citation62,Citation63] support the findings of our study that heat could have an association in the induction of DNA damage with consequent HSP release in human subjects. Professional or occupational drivers and individuals who have long daily commuting are more prone to having increased scrotal temperatures that were associated with sperm damage [Citation64]. In a review on the causes and effect of heat stress on sperms, Durairajanayagam et al. discussed the effect on temperature on the sperm concentration & count and reported 30% lower sperm concentration & count during hotter seasons [Citation24]. In addition to damaging DNA, hyperthermia also causes a decrease in DNA synthesis and the degradation of many mRNAs and proteins necessary for cell survival [Citation65]. The significant positive correlation between heat stress exposures and DNA damage in our study corroborates with previous reports [Citation53–Citation55] that DNA damage is a potential consequence of chronic high-heat exposures. Long-term increase in temperatures due to climate change is likely to increase the incidence of heat-related illnesses [Citation66] and an understanding of the impacts of heat stress at a sub-cellular level is essential.
The study is preliminary in nature and the first of its kind to explore the association between occupational heat exposures and DNA damage in organized Indian occupational settings. One of the major limitations of the study is the cross-sectional study design, the small sample size, and samples being collected only from one industry. The inclusion of more industrial sectors with various exposure patterns and heat levels are warranted to come to conclusive conclusions about the dose-response relationship between heat exposures and DNA damage. Within the limitations of the study, the findings do concur with other studies that have also shown associations between high-heat stress exposures and DNA damage [Citation11,Citation20,Citation24,Citation26,Citation34,Citation35].
Conclusions
Heat-related illnesses are one of the silent causes of morbidities and mortalities worldwide and will continue to increase in severity with the rise in global temperatures due to climate change. Heat stress not only affects workers health and productivity but also inhibits DNA repair systems and can also act as a DNA damaging agent. In summary, this preliminary research evidence indicates that high-heat exposures can potentially induce DNA damage and genetic damage, an area of research that has been under-addressed at a global level. Need for further longitudinal and in-depth studies to understand the long-term effects is warranted to be conclusive about the heat and DNA damage dose-response relationship. A complete understanding of heat-related illnesses at physiological, as well as molecular level, is required to facilitate the design of more efficient preventive and treatment strategies. Given the research evidence, preventive actions must prioritize safe workplace conditions for the workers which may be achieved through adequate ventilation and feasible interventions. In general, the study highlights the need for protective labor policies for the working population exposed to chronic high-heat work environments with an aim prevent adverse heat-related health implications for the working population in the hotter world as climate change proceeds.
Methods
Study design and study population
We conducted a cross-sectional study to assess occupational heat stress and its association with damages at the subcellular levels (DNA) in a steel industry located in southern India which was a follow-up of a previous study conducted by the authors [Citation17]. Prior ethical clearance from the Institutional Ethics Committee (IEC) and permission from the concerned management was obtained for the study. We selected the industry for the study as the workers in the steel industry were exposed to chronic high-heat BF-II on a regular basis. The willingness of the management to participate in the study and acceptance for further follow-ups with workers such as medical surveillance was an added reason for the selection of this industry. After we conducted a walk-through survey and made spot WBGT measurements within the industry, we recruited 120 workers employed for more than 5 years in the same industry from various locations within the industry who volunteered and consented for the study. The 5-year cut-off was kept to rule out any other risk occupational risk factors, that may drive the association due to any recent exposures from a change of job from other occupational sectors. The risks and benefits of participating in the study were explained to the workers and signed informed consent was obtained. The workers who had 5 years’ experience in the same industry also had the following advantages: 1) they were tenured workers with occupational health records and 2) experienced workers were only posted in key manufacturing areas, most of which had very high-heat exposures. The identified areas were Coke Oven (CO), Blast furnace (BF), Continuous Casting Mill (CCM), Energy Optimizing Furnace (EOF), Blooming Mill (BM), Coke Oven (CO), and the Power Plant (PP). Based on the spot WBGT measurements done for screening purposes, we included 76 workers with WBGT exposures ≥ 27.5°C (ACGIH – Threshold Limit Value (TLV) [Citation67] who were employed in areas where they had direct exposures to process-generated heat like from furnaces. Among the exposed workers, workers with exposures ≥30°C were categorized as high-heat exposed group and workers with exposures 27.5–30°C were categorized as a medium-exposed group. We categorized the other 44 workers based on their exposures to temperatures <27.5°C (below TLV) as unexposed workers who were employed in jobs such as housekeeping, offices, who were not directly exposed to process generated heat after frequency matching them to the exposed workers by age and years of experience in the industry.
Assessment of heat stress, physiological strain and workers perception on health
Heat stress measurements
We made quantitative assessments of environmental area heat stress using a calibrated portable heat stress WBGT monitor, (QuesTemp°34; QUEST Technologies, USA) during the hottest part of the day, (10am–4pm) which has an accuracy level of ±0.5°C between 0°C and 120°C of dry bulb temperature and ±5% relative humidity, as per the protocols recommended by NIOSH [Citation68]. We used the WBGT permissible heat exposure TLV to evaluate the risk of heat stress and the corresponding WBGT under which continuous work during an hour could be safely undertaken. In each work location, we measured WBGT at least twice a day and the average WBGT of the measurements was used as workers’ exposure during the shift. Then clothing correction was made to the WBGT values depending on the kind of clothing/uniform worn by the workers as per the ACGIH guidelines [Citation67]. The heat exposures (heat stress index that comprises temperature, relative humidity, radiation, and wind velocity) was recorded for each worker plus workload, clothing factors were collected by using standard methods [Citation69].
Physiological strain measurements
We collected data on physiological parameters, such as core body temperature (CBT), Sweat Rate (SwR), and Urine Specific Gravity (USG) [Citation70], as per the standard internationally accepted procedures for workers. CBT was assessed using 3M, Quest Temp Personal II monitors. The aural sensor has an electronic accuracy of better than ± 0.1°C over the temperature range of 32°C to 40°C. This gives minute-by-minute CBT variations experienced by the worker through the shift. We measured it before the onset of work (pre-exposure CBT) and after at least 2 hours into their work shift (post-exposure CBT). The workers were made to sit in a comfortable chair before the pre and post measurements were taken. The sweat rate was calculated using the formula of the Canadian Sports Association [Citation71]. The body weight was measured, using a weighing scale (accurate to 0.1 kg) before the start and end of the work. Data on the quantity of liquid consumption during the study time in a work shift and the time gap between urinations were collected from the study participants to check the Sweat rate. The urine sample was collected after their work shift. USG was measured via a standard Urinometer. The reference range used for the physiological parameters was 1) Raise in 1°C in post-exposure CBT [Citation4]. 2) SwR of 1.0 L/h [Citation71] and 3) USG was between the ranges of 1.010–1.020 [Citation69].
Workers’ perception of heat and health impacts
We collected a total of 120 perception questionnaires using a modified version of an internationally validated HOTHAPS (High Occupational Temperature Health and Productivity Suppression) questionnaire from the workers [Citation47]. Data on the workers’ perceptions of heat exposures, clothing acclimatization, symptoms of potential health impacts, were collected. An elaborate section with questions on self-reported heat-related health illnesses was administered and the interviewer explained the symptoms of each illness. We also made observations about the workplace conditions, provision of cooling interventions and coping mechanisms of workers in managing heat stress.
Heat stress-induced DNA damage measured using micro nuclei assay
Prevalence of high chronic heat exposures and heat-related health illnesses was common in the steel industry and workers were highly vulnerable to risks of adverse health issues. The steelworkers were selected to study the association between heat stress and DNA damage due to the very high chronic heat exposures on a daily basis.
We used the standard methods used for the MN assay method [Citation57,Citation72–Citation75]. About 2–3 ml of peripheral blood was collected in a heparinized container and transported to the laboratory at 4 ± 1°C. In a culture flask, 80% of RPMI 1640 medium with 20% of FBS, 1-ml of the peripheral blood and 40 μg/ml of phytohemagglutinin (PHA) were added. The cultures were incubated at 37°C for 72h. At the 44th hour, cells were blocked at cytokinesis stage using cytochalasin-B (4 μg/ml) [Citation76]and further incubated for 24 h at 37°C. At the end of the 72nd hour, the cells were treated with hypotonic (0.45%) solution and fixed with Carnoy’s Fixative. The slides were cast and stained with 10% Giemsa followed by scoring the slides at 40X magnification under the light microscope (Nikon Eclipse) MN and documentation. Slides of the exposed and unexposed samples (with about 1000 cells) were analyzed for each sample and the MN frequency was calculated using the Fenech formula [Citation77].
The MN frequency, an indicator of DNA damage, in the exposed group ranged between 0.026–0.45 as against 0.007 which was the baseline in regular population [Citation44,Citation78].
Reconfirmation of micro nucleus using automated cyto-genetic imaging
For reconfirmation, increased accuracy and to remove any researcher bias/error, we assessed MN induction using a semi-automated Metafer™ DNA damage scoring platform (Meta System, Althlussheim, Germany). The Metafer MN scoring platform consists of a motorized slide loading platform, Carl Zeiss Axio Imager fluorescence microscope, and a Charge-Coupled Device (CCD) camera. Image acquisition was carried out by using Metafer 4 software (version 3.12.137). We loaded the stained slides on to a motorized slide scanning platform of the Metafer system, scanned the slides and the images of nuclei and MN were captured with 10X objective. A 100X objective was used for MN scoring by relocating the cell and MN on the slide form the coordinates displayed in the gallery view. The MN module for MetaferSearch finds cells on a slide and automatically counts the MN. Each BN cell is captured and the image is displayed in the gallery. A real-time histogram lists the BN cells with the micronuclei counts. Non-overlapping, DAPI stained circular/oval nuclei with a size between a third and a sixteenth of the main nuclei were scored as MN [Citation79].
Statistical analysis
Baseline characteristics between participants and non-participants were compared. Continuous data is represented as Mean ± SD. For single proportions like the prevalence of heat stress and heat-related health problems, etc. were tested for significance using the Chi-square test and Crude Odds Ratio (OR). The dependent variable was categorized as dichotomic variables using a cut-off value corresponding to the baseline MN frequency of 0.07 MN/cell in southern India based on a previous study [Citation44] to find the risk of DNA damage due to heat exposures between the exposed and unexposed worker groups. Hence binary logistic regression was used for analysis using a stepwise method to control for possible confounders [Citation55]. The adjusted ORs thus calculated is presented with the corresponding p-values and 95% CI. The level of significance was taken at 5% level. SPSS-licensed statistical software version 16, and “R” software were used for statistical analysis and graphs were created using Microsoft Excel.
Abbreviations
ACGIH | = | American Conference of Governmental Industrial Hygienists |
BF | = | Blast furnace |
BM | = | Blooming Mill |
BN | = | Bi-Nucleate Cells |
CBT | = | Core Body Temperature |
CCM | = | Continuous casting mill |
CO | = | Coke Oven |
DNA | = | DeoxyRibo Nucleic Acid |
EOF | = | Energy optimizing Furnace |
HOTHAPS | = | High Occupational Temperature Health and Productivity Suppression |
MN | = | Micro Nuclei |
NIOSH | = | National Institute for Occupational Safety and Health |
OR | = | Odds Ratio |
PHA | = | Phytohemagglutinin |
SwR | = | Sweat Rate |
TLV | = | Threshold Limit Value |
USG | = | Urine Specific Gravity |
WBGT | = | Wet Bulb Globe Temperature |
Authors’ contributions
Dr. VV, MK, and Dr. Vetri conceived the initial idea. Dr. VJ, Dr. SFDP took lead in sample analysis. SR and KK undertook the statistical analysis in conjunction with Dr. VV. All authors contributed to statistical interpretation. MK, Dr. VV wrote the draft manuscript. All authors supported in manuscript preparation.
Institution and Ethics approval and informed consent
Prior ethical clearance was obtained from the ethical committee of Sri Ramachandra Institute of Higher Education & Research, (Ref No: IEC-NI/14 August 1941/51) and their authors declare no competing interest for the present study. Informed consent was taken from each worker.
Acknowledgments
The author thanks the participants for their involvement and also acknowledges the organized sector management for permission to conduct the study. The authors are grateful for the support rendered by Sri Ramachandra Institute of Higher Education & Research for providing the platform to conduct the study. The authors are highly appreciative of the technical support provided by Dr. P. Venkatachalam, Professor of Genetics and Associate Dean (Research) throughout the study. Financial support for student fellowship and heat assessment part of the study by the Department of Science and Technology, Govt. of India is appreciated (Project sanction no Grant No. DST/CCP/(NET-2) PR-37/2012(G).
Disclosure statement
No potential conflict of interest was reported by the authors.
Additional information
Funding
References
- Haines A, Patz JA. Health effects of climate change. Jama. 2004;291(1):99–103.
- Costello A, Abbas M, Allen A, et al. Managing the health effects of climate change: lancet and University College London Institute for Global Health Commission. Lancet. 2009;373(9676):1693–1733.
- Kovats RS, Hajat S. Heat stress and public health: a critical review. Annu Rev Public Health. 2008;29:41–55.
- Parsons K. Human thermal environments: the effects of hot, moderate, and cold environments on human health, comfort, and performance. London: CRC press; 2014.
- Kjellstrom T, Kovats RS, Lloyd SJ, et al. The direct impact of climate change on regional labor productivity. Arch Environ Occup Health. 2009;64(4):217–227.
- Dutta P, Chorsiya V. Scenario of climate change and human health in India. Int J Innov Res Dev. 2013;2(8): 157-160.
- Wesseling C, Crowe J, Hogstedt C, et al. Resolving the enigma of the mesoamerican nephropathy: a research workshop summary. Am J Kidney Dis. 2014;63(3):396–404.
- Mitchell KM, Cheuvront SN, King MA, et al. Use of the heat tolerance test to assess recovery from exertional heat stroke. Temperature. 2019; 6(2):1–14. doi: 10.1080/23328940.2019.1574199.
- Chen M-L, Chen C-J, Yeh W-Y, et al. Heat stress evaluation and worker fatigue in a steel plant. Aiha J. 2003;64(3):352–359.
- Xiang J, Bi P, Pisaniello D, et al. Health impacts of workplace heat exposure: an epidemiological review. Ind Health. 2014;52(2):91–101.
- Bouchama A, Aziz MA, Al Mahri S, et al. A model of exposure to extreme environmental heat uncovers the human transcriptome to heat stress. Sci Rep. 2017;7(1):9429.
- Kreft S, Eckstein D, Melchior I Global Climate Risk Index 2017: Who suffers most from extreme weather events? Weather-related loss events in 2015 and 1996–2015: Germanwatch Nord-Süd Initiative eV, 2016. https://germanwatch.org/sites/germanwatch.org/files/publication/16411.pdf
- Kjellstrom T, Lemke B, Otto M, et al. Threats to occupational health, labor productivity and the economy from increasing heat during climate change: an emerging global health risk and a challenge to sustainable development and social equity. Mapua (New Zealand): Health and Environment International Trust; 2014.
- Kenny GP, Poirier MP, Metsios GS, et al. Hyperthermia and cardiovascular strain during an extreme heat exposure in young versus older adults. Temperature. 2017;4(1):79–88. doi: 10.1080/23328940.2016.1230171.
- Ramachandran A, Praveen D, Jaganathan R, et al. Spatiotemporal analysis of projected impacts of climate change on the major C3 and C4 crop yield under representative concentration pathway 4.5: insight from the coasts of Tamil Nadu, South India. PloS oneGlob Health Action. 2017;12(7):e0180706.
- Humphreys M. Outdoor temperatures and comfort indoors. Batiment Int Building Res Pract. 1978;6(2):92.
- Krishnamurthy M, Ramalingam P, Perumal K, et al. Occupational heat stress impacts on health and productivity in a steel industry in southern India. Saf Health Work. 2017;8(1):99–104.
- Nag PK, Nag A, Sekhar P, et al.: Vulnerability to Heat Stress: scenario in Western India, WHO APW SO 08 AMS 6157206, National Institute of OccupationalHealth,Ahmedabad, http://www.searo.who.int/india/topics/occupational_health/Occupational_Health_Vulnerability_to_heat_stress_scenario_of_western_India.pdf Available from 2018 Jan 17, 2009.
- Methner M, Eisenberg J. Evaluation of heat stress and heat strain among employees working outdoors in an extremely hot environment. J Occup Environ Hyg. 2018;15(6):474–480.
- Kantidze O, Velichko A, Luzhin A, et al. Heat stress-induced DNA damage. Acta Naturae. 2016;8(2 (29)): 75-78.
- Venugopal V, Krishnamoorthy M, Venkatesan V, et al. Occupational heat stress, DNA damage and heat shock protein-a review. Med Res Arch. 2018;6(1). DOI:10.18103/mra.v6i1.1631
- Bridge AJ, Pebernard S, Ducraux A, et al. Induction of an interferon response by RNAi vectors in mammalian cells. Nat Genet. 2003;34(3):263.
- Purschke M, Laubach H-J, Anderson RR, et al. Thermal injury causes DNA damage and lethality in unheated surrounding cells: active thermal bystander effect. J Invest Dermatol. 2010;130(1):86–92.
- Durairajanayagam D, Agarwal A, Ong C. Causes, effects and molecular mechanisms of testicular heat stress. Reprod Biomed Online. 2015;30(1):14–27.
- Harrouk W, Codrington A, Vinson R, et al. Paternal exposure to cyclophosphamide induces DNA damage and alters the expression of DNA repair genes in the rat preimplantation embryo. Mutat Res. 2000;461(3):229–241.
- Xiao C, Chen S, Li J, et al. Association of HSP70 and genotoxic damage in lymphocytes of workers exposed to coke-oven emission. Cell Stress Chaperones. 2002;7(4):396–402.
- Pelham H. Hsp70 accelerates the recovery of nucleolar morphology after heat shock. Embo J. 1984;3(13):3095–3100.
- Rockett JC, Mapp FL, Garges JB, et al. Effects of hyperthermia on spermatogenesis, apoptosis, gene expression, and fertility in adult male mice. BiolReprod. 2001;65(1):229–239.
- Tramontano F, Malanga M, Farina B, et al. Heat stress reduces poly (ADPR) polymerase expression in rat testis. Mol Hum Reprod. 2000;6(7):575–581.
- Ramirez A, Saldanha PH. Micronucleus investigation of alcoholic patients with oral carcinomas. Genet Mol Res. 2002;1(3):246–260.
- Jagetia GC, Baliga MS. Syzygium cumini (Jamun) reduces the radiation-induced DNA damage in the cultured human peripheral blood lymphocytes: a preliminary study. ToxicolLett. 2002;132(1):19–25.
- Hintzsche H, Riese T, Stopper H. Hyperthermia-induced micronucleus formation in a human keratinocyte cell line. Mutat Res. 2012;738:71–74.
- Feng H, Sun L, Li Y, et al. Study on induced mutagenesis interaction of the high temperature and/or cigarette smoke. Wei Sheng Yan Jiu. 1998;27(6):379–381.
- Fehrenbach E, Northoff H. Free radicals, exercise, apoptosis, and heat shock proteins. Exerc Immunol Rev. 2001;7:66–89.
- Yang X, Zheng J, Bai Y, et al. Using lymphocyte and plasma Hsp70 as biomarkers for assessing coke oven exposure among steel workers. Environ Health Perspect. 2007;115(11):1573.
- Stich HF, Curtis JR, Parida BB. Application of the micronucleus test to exfoliated cells of high cancer risk groups: tobacco chewers. Int J Cancer. 1982;30(5):553–559.
- Stich HF, Rosin MP, Hornby AP, et al. Remission of oral leukoplakias and micronuclei in tobacco/betel quid chewers treated with beta‐carotene and with beta‐carotene plus vitamin A. Int J Dent. 1988;42(2):195–199.
- Jyoti S, Siddique YH, Khan S, et al. Effect on micronucleus frequency and DNA damage in buccal epithelial cells of various factors among pan masala and gutkha chewers. Oral Sci Int. 2015;12:9–14.
- Kassie F, Parzefall W, Knasmüller S. Single cell gel electrophoresis assay: a new technique for human biomonitoring studies. Mutat Res. 2000;463(1):13–31.
- Dobrzyńska MM, Pachocki KA, Gajowik A, et al. The effect occupational exposure to ionizing radiation on the DNA damage in peripheral blood leukocytes of nuclear medicine personnel. J Occup Health. 2014;56(5):379–386.
- Wang H, Chen W, Zheng H, Guo L, Liang H, Yang X, Bai Y, Sun J, Su Y, Chen Y, Yuan J. Association between plasma BPDE-Alb adduct concentrations and DNA damage of peripheral blood lymphocytes among coke oven workers. Occupational and environmental medicine. 2007 Nov 1;64(11):753–8.
- Singh RK, Mishra SK, Kumar N, et al. Assessment of DNA damage by comet assay in lymphocytes of workers occupationally exposed to petroleum fumes. Int J Genet. 2010;2(1):18.
- Intranuovo G, Schiavulli N, Cavone D, et al. Assessment of DNA damages in lymphocytes of agricultural workers exposed to pesticides by comet assay in a cross-sectional study. Biomarkers. 2018;23(5):462–473.
- Paul SF, Venkatachalam P, Jeevanram R. Analysis of radiation dose-response curve obtained with cytokinesis block micronucleus assay. Nucl Med Biol. 1997;24(5):413–416.
- Pachauri RK, Allen MR, Barros VR, et al. Climate change 2014: synthesis report. Contribution of Working Groups I, II and III to the fifth assessment report of the Intergovernmental Panel on Climate Change: IPCC, 2014.
- Ayyappan R, Sankar S, Rajkumar P, et al. Work-related heat stress concerns in automotive industries: a case study from Chennai, India. Glob Health Action. 2009;2(1):2060.
- Venugopal V, Chinnadurai JS, Lucas RA, et al. Occupational heat stress profiles in selected workplaces in India. Int J Environ Res Public Health. 2016.13(1):89.
- American Conference of Governmental Industrial Hygienists (ACGIH). Threshold limit value for chemical substances and physical agents and biological exposure indices, 2010:112.
- Lemke B, Kjellstrom T. Calculating workplace WBGT from meteorological data: a tool for climate change assessment. Ind Health. 2012;50(4):267–278.
- Rooney C, McMichael AJ, Kovats RS, et al. Excess mortality in England and Wales, and in Greater London, during the 1995 heatwave. Epidemiol Community Health. 1998;52(8):482–486.
- Sawka MN, Latzka WA, Montain SJ, et al. Physiologic tolerance to uncompensable heat: intermittent exercise, field vs laboratory. J Appl Physiol. 2001;33(3):422–430.
- Aragón-Vargas LF, Moncada-Jiménez J, Hernández-Elizondo J, et al. Evaluation of pre-game hydration status, heat stress, and fluid balance during professional soccer competition in the heat. Ter Arkh. 2009;9(5):269–276.
- Casa DJ. Exercise in the heat. II. Critical concepts in rehydration, exertional heat illnesses, and maximizing athletic performance. J Athl Train. 1999;34(3):253.
- Lucas RA, Bodin T, García-Trabanino R, Wesseling C, Glaser J, Weiss I, Jarquin E, Jakobsson K, Wegman DH. Heat stress and workload associated with sugarcane cutting—an excessively strenuous occupation! Extrem Physiol Med. 2015;4(Suppl 1):A23.
- Ladeira C, Viegas S, Carolino E, et al. Genotoxicity biomarkers in occupational exposure to formaldehyde—the case of histopathology laboratories. Mutat Res. 2011;721(1):15–20.
- Ziętkiewicz E, Wojda A, Witt M. Cytogenetic perspective of ageing and longevity in men and women. J Appl Genet. 2009;50(3):261–273.
- Bolognesi C, Lando C, Forni A, et al. Chromosomal damage and ageing: effect on micronuclei frequency in peripheral blood lymphocytes. Age Ageing. 1999;28(4):393–397.
- Bonassi S, Neri M, Lando C, et al. Effect of smoking habit on the frequency of micronuclei in human lymphocytes: results from the human micronucleus project. Mutat Res. 2003;543(2):155–166.
- Wu T-C, Yuan Y, Y-Y B, et al. Plasma free amino acids in workers working under different stress conditions. J Occup Health. 1998;40(3):203–206.
- Selkirk GA, McLellan TM, Wright HE, et al. Expression of intracellular cytokines, HSP72, and apoptosis in monocyte subsets during exertional heat stress in trained and untrained individuals. Am J Physiol Regul Integr Comp Physiol. 2009;296(3):R575–R86.
- Meyn RE, Corry PM, Fletcher SE, et al. Thermal enhancement of DNA damage in mammalian cells treated with cis-diamminedichloroplatinum (II). Cancer Res. 1980;40(4):1136–1139.
- Oh HJ, Easton D, Murawski M, et al. The chaperoning activity of hsp110 identification of functional domains by use of targeted deletions. J Biol Chem. 1999;274(22):15712–15718.
- Oh HJ, Chen X, Subjeck JR. Hsp110 protects heat-denatured proteins and confers cellular thermoresistance. J Biol Chem. 1997;272(50):31636–31640.
- Bujan L, Daudin M, Charlet J-P, et al. Increase in scrotal temperature in car drivers. Hum Reprod. 2000;15(6):1355–1357.
- Izu H, Inouye S, Fujimoto M, et al. Heat shock transcription factor 1 is involved in quality-control mechanisms in male germ cells. Biol Reprod. 2004;70(1):18–24.
- Luber G, McGeehin M. Climate change and extreme heat events. Am J Prev Med. 2008;35(5):429–435.
- ACGIH American Conference of Governmental960 Industrial Hygienists (ACGIH). Heat stress and strain: TLV(R) physical agents. (2019) ISBN: 978-1-607261-06-3
- NIOSH. Occupational exposure to hot environment.U.S. USA: Department of Health and Human Services; 1986. p. 86–113.
- Casa DJ, Armstrong LE, Hillman SK, et al. National athletic trainers’ association position statement: fluid replacement for athletes. J Athl Train. 2000;35(2):212.
- Venugopal V, Rekha S, Manikandan K, et al. Heat stress and inadequate sanitary facilities at workplaces–an occupational health concern for women? Glob Health Action. 2016;9(1):31945.
- Palmer MS, Spriet LL. Sweat rate, salt loss, and fluid intake during an intense on-ice practice in elite Canadian male junior hockey players. Appl Physiol Nutr Metab. 2008;33(2):263–271.
- Kirsch-Volders M, Elhajouji A, Cundari E, et al. The in vitro micronucleus test: a multi-endpoint assay to detect simultaneously mitotic delay, apoptosis, chromosome breakage, chromosome loss and non-disjunction. Mutat Res. 1997;392(1):19–30.
- Fenech M, Holland N, Chang WP, et al. The HumanMicroNucleus project—an international collaborative study on the use of the micronucleus technique for measuring DNA damage in humans. Mutat Res. 1999;428(1–2):271–283.
- Fenech M, Bonassi S. The effect of age, gender, diet and lifestyle on DNA damage measured using micronucleus frequency in human peripheral blood lymphocytes. Mutagenesis. 2011;26(1):43–49.
- Fenech M, Morley AA. Measurement of micronuclei in lymphocytes. Mutat Res. 1985;147(1–2):29–36.
- Fenech M, Morley AA. Cytokinesis-block micronucleus method in human lymphocytes: effect of in vivo ageing and low dose X-irradiation. Mutat Res. 1986;161(2):193–198.
- Countryman PI, Heddle JA. The production of micronuclei from chromosome aberrations in irradiated cultures of human lymphocytes. Mutat Res. 1976;41(2–3):321–331.
- Ban S, Konomi C, Iwakawa M, et al. Radiosensitivity of peripheral blood lymphocytes obtained from patients with cancers of the breast, head and neck or cervix as determined with a micronucleus assay. J Radiat Res. 2004;45(4):535–541.
- Fenech M, Bonassi S, Turner J, et al. Intra-and inter-laboratory variation in the scoring of micronuclei and nucleoplasmic bridges in binucleated human lymphocytes: results of an international slide-scoring exercise by the HUMN project. Mutat Res. 2003;534(1):45–64.