Abstract
Body segment inertial parameters (BSIPs) are essential in biomechanics and regressions, in particular scaling equations based on segment lengths, are one of the most practical methods to obtain them. Adjustment of the BSIPs from McConville et al. and Young et al. has been previously proposed to define BSIPs and segment lengths based on joint centres. However, due to the unavailable estimation of the thoracic joint centre, the trunk was previously segmented into two segments: pelvis and torso, restricting the possible applications to movements with limited flexion or bending of the trunk. This short communication presents an update of the adjustment procedure to obtain the BSIPs of the thorax and abdomen segments. The thoracic joint centre is obtained by interpolation based on literature data and allows the computation of the relevant abdomen and thorax segment coordinate systems and segment lengths. The updated scaling equations may be useful for researchers who would like to use the adjusted BSIPs with a more detailed trunk definition.
Introduction
Body segment inertial parameters (BSIPs) are essential parameters in biomechanics when studying the dynamics of the human movement. They can be estimated for a given subject using different methods (Reid & Jensen Citation1990; Jensen Citation1993; Pearsall & Reid Citation1994; Erdmann Citation1999), one of them being the use of regressions and, in particular, the use of scaling equations (i.e. ratios for mass, centre of mass and radii of gyration). Accordingly, each segment mass is computed as a percentage of the subject’s body mass. The position of the centre of mass in the segment coordinate system is computed as a percentage of the subject’s segment length. The square roots of the moments of inertia with respect to the axes of the segment coordinate system, divided by the segment mass, are computed as percentages of the subject’s segment length. As BSIPs have been generally defined in some specific coordinate systems and with respect to segment lengths both based on external anatomical landmarks, some adjustment procedures have been performed in the literature (Hinrichs Citation1990; de Leva Citation1996; Dumas et al. Citation2007a) in order to propose scaling equations more consistent with the segment coordinate system generally used in motion analysis.
For instance, scaling equations adjusted from McConville et al. (Citation1980) and Young et al. (Citation1983) have been proposed (Dumas et al. Citation2007a, 2007b). These scaling equations matched the recommendations of the international society of biomechanics (ISB) (Wu et al. Citation2002, 2005) for the definition of the segment coordinates systems (SCSs). In addition, these scaling equations represented the only regressions to provide estimations for the anterior–posterior and medial–lateral positions of centres of mass as well as for the products of inertia.
However, in these scaling equations (Dumas et al. Citation2007a), the definition for the trunk segments remained inappropriate. In particular, the torso was considered as a unique segment, without distinction between the thorax and the abdomen. This segmentation issue could have prevented using these adjusted BSIPs for application in movements including large flexion or bending of the trunk.
In order to overcome this limitation, a solution was recently found to obtain the adjusted BSIPs of the thorax and abdomen segments and this short communication presents an update of the previous adjustment procedure and scaling equations (Dumas et al. Citation2007a). First, the limitation of the previous adjustment procedure is reviewed. Then, the updated adjustment procedure is presented which includes (a) the definition of the thoracic joint centre (TJC) from the data available in McConville et al. (Citation1980) and Young et al. (Citation1983) and from the anatomical landmarks more generally used in motion analysis, (b) the definition of the abdomen and thorax segment coordinate systems and (c) the computation of the updated scaling equations and their application to a sit-to-stand movement performed by seven subjects.
Previous adjustment procedure
In the previous adjustment procedure (Dumas et al. Citation2007a), no estimation was relevant for the position of the TJC. The positions of the other joint centres of the trunk (i.e. cervical joint centre [CJC], lumbar joint centre [LJC] and shoulder joint centre [SJC]) were obtained according to the regressions established by Reed et al. (Reed et al. Citation1999). These regressions were based on the data of Schneider et al. (Schneider et al. Citation1983). As the regressions were only established for males (Reed et al. Citation1999), the same data (Schneider et al. Citation1983) were used to complete them for females (see appendix in [Dumas et al. Citation2007a]). However, such regressions could not be applied for the position of the TJC because it required anatomical landmarks (i.e. spinal processes landmarks of the eighth and twelfth thoracic vertebrae, T8 and T12) which were not reported in the original data of McConville et al. (Citation1980) and Young et al. (Citation1983). No alternative estimation of the position of the TJC was found at the time of the previous adjustment procedure. That is why only the BSIPs of a torso segment were adjusted, although McConville et al. (Citation1980) and Young et al. (Citation1983) provided separated data for thorax and abdomen segments.
Updated adjustment procedure
Estimation of the TJC
TJC from the data available in McConville et al. (Citation1980) and Young et al. (Citation1983)
In the updated adjustment procedure, the position of the TJC is interpolated directly from the data of Schneider et al. (Schneider et al. Citation1983) by a Kriging method (Trochu Citation1993; Dumas & Cheze Citation2009). The principle is to use the 3D positions of some points in two datasets to compute the parameters of an interpolation function (i.e. a linear polynomial of coordinates plus a weighted average of inter-point distances). The position of any point available in only one of both datasets can be then interpolated (see Appendix 1). The positions of eight anatomical landmarks (i.e. midspine at 10th rib level [MSR10], right and left 10th ribs [RR10 and LR10], right and left anterior-superior iliac spines [RASIS and LASIS], symphision [SYM], and right and left iliocristales [RI and LI]) are available in Schneider et al. (Schneider et al. Citation1983) and in McConville et al. (Citation1980) and Young et al. (Citation1983) for males and females, respectively. These anatomical landmarks allow the computation of the interpolation parameters and, thus, interpolating the position of TJC in the abdomen anatomical coordinate system (ACS), as defined by McConville et al. (Citation1980) and Young et al. (Citation1983) for males and females, respectively. These ACSs for all segments are based on external anatomical landmarks and differ from the SCSs generally used in motion analysis that are based on joint centres (Wu et al. Citation2002, 2005).
TJC from anatomical landmarks used in motion analysis
The 3D interpolation is necessary in the updated adjustment procedure because no regression exists based on the anatomical landmarks available in McConville et al. (Citation1980) and Young et al. (Citation1983). However, when applying the scaling equations to estimate the BSIPs of a given subject, the position of the TJC can be preferably estimated using regressions, in the same way as the positions of the other joint centres. A regression for the position of the TJC has been proposed, for males only, by Reed et al. (Reed et al. Citation1999). The TJC is defined (a) on a direction forming, in the sagittal plane of the thorax, an angle of 94° with respect to the vector from T12 to T8 and (b) at a distance of 52% of the thorax depth. Thorax depth is the distance from the 7th cervicale (C7) to the suprasternale (SUP). In the present study, such regression for the position of the TJC is also completed for females, based on the anthropometric measurements from Schneider et al. (Schneider et al. Citation1983). Therefore, the TJC is defined (a) on a direction forming, in the sagittal plane of the thorax, an angle of 83° with respect to the vector from T12 to T8 and (b) at a distance of 53% of the thorax depth.
Definition of abdomen and thorax SCS
Abdomen SCS
To define the axes of abdomen SCS, the position of the LJC, obtained in the pelvis ACS during the previous adjustment procedure (Dumas et al. Citation2007a), is transformed to the abdomen ACS. To do so, the sagittal planes of the abdomen ACS and the pelvis ACS are first assumed to be coincident. The translation and the rotation between both ACSs, in this plane, are computed from two anatomical landmarks (i.e. cut centroid and midpoint between posterior-superior iliac spines [MPSIS]) available in both ACSs in the data of Young et al. (Citation1983). Second, for the data of McConville et al. (Citation1980), the rotation is assumed to be the same as for Young et al. (Citation1983) and the translation is computed from one anatomical landmark (i.e. MPSIS) available in both ACSs.
The abdomen SCS is then defined as follows. The Y axis of the abdomen SCS runs from the LJC to the TJC and is normed. The Z axis is the normal to the sagittal plane of the abdomen ACS defined by McConville et al. (Citation1980) and Young et al. (Citation1983) for males and females, respectively. As TJC and LJC lie in the sagittal plane of the abdomen ACS, Y and Z axes are orthogonal. The X axis is the cross product of the Y and Z axes, pointing anteriorly. The origin is the TJC (Figure ).
Figure 1. Locations of selected anatomical landmarks from McConville et al. (Citation1980) and from Young et al. (Citation1983) and orientations of the segment coordinate systems (SCSs) built from these landmarks.
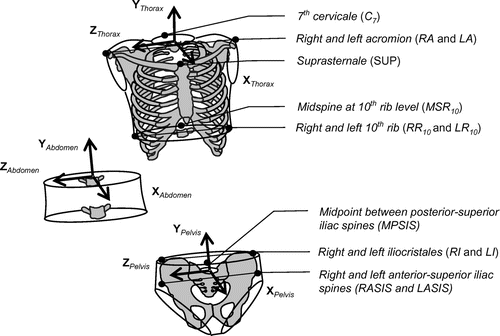
Thorax SCS
To define the axes of the thorax SCS, the position of the TJC is transformed from the abdomen ACS to the thorax ACS. For that, the transformation from the pelvis ACS to the abdomen ACS, as mentioned above, and the transformation from the pelvis ACS to the thorax ACS, determined in the previous adjustment procedure (Dumas et al. Citation2007a), are both used to compute the transformation from the abdomen ACS to the thorax ACS.
The thorax SCS is then defined as follows. The Y axis of the thorax SCS runs from the TJC to the CJC and is normed. The Z axis is the normal to the sagittal plane of the thorax ACS defined by McConville et al. (Citation1980) and Young et al. (Citation1983) for males and females, respectively. As CJC and TJC lie in the sagittal plane of the thorax ACS, Y and Z axes are orthogonal. The X axis is the cross product of the Y and Z axes, pointing anteriorly. The origin is the CJC (Figure ).
Adjusted scaling equations
Segment mass, position of centre of mass, moments and products of inertia
For the thorax and abdomen, the segment lengths, L, are computed as the distances between the proximal and the distal joint centres. The anatomical landmarks (C7, SUP, MSR10, RR10, RI, MPSIS, RASIS), mentioned above, plus the right acromion (RA) and the joint centres (CJC, SJC, TJC, LJC) as well as the centres of mass (COM) are expressed in the SCSs of abdomen and thorax (Table ) with the determined transformations from the ACSs to the SCSs. These transformations are also used to compute the inertia tensors at COM, I, with respect to the SCSs axes.
Table 1. 3D positions of the anatomical landmarks in the segment coordinate systems (SCSs) of the thorax and abdomen.
The segment mass m is expressed relative to the total body mass (without adjustment), assuming a uniform density of 1 g.cm−3. The vector from the origin of the SCS to the COM is expressed relative to the segment length L. Both moments and products of inertia with respect to the SCSs axes are expressed relative to m and L: (with i and j denoting the X, Y, or Z axis of the SCS). The adjusted scaling equations are given in Table .
Table 2. Definition of the segment lengths and of the origins of the segment coordinate systems (SCSs) – values of the segment length L (used for scaling) and of the scaling factors for the segment mass m, the position of centre of mass, the moments of inertia and the products of inertia (i denotes negative product of inertia).
Application to sit-to-stand movement
To evaluate the impact of using two thorax and abdomen segments rather than one torso segment, the joint moments at the LJC are computed during two repeated sit-to-stand movements of seven subjects (Robert et al. Citation2011). The seat heights were set at 50% of the knee height leading to large flexion of the trunk. Both a 15-segment model (i.e. with a torso segment) and a 16-segment model (i.e. with thorax and abdomen segments) were used. Accordingly, the BSIPs were estimated using the scaling equations obtained with the previous and the updated adjustment procedures. The root mean square (RMS) differences, ε15 and ε16, are computed between the lumbar joint moments obtained with a bottom-up and a top-down computation (Robert et al. Citation2007). These differences are representative of all the modelling errors including the segmentation and the BSIPs.
This preliminary application reveals a decrease in the difference between bottom-up and top-down computations when using a 16-segment model (i.e. with thorax and abdomen segments). The average (standard deviation) improvement (Δ = ε16−ε15) was 8.2 (±1.4) N.m on the Z axis of the pelvis. No substantial modifications were found concerning the other axes.
Discussion
Updated adjustment procedure
Estimations of the joint centres
For the adjustment of the BSIPs of thorax and abdomen segments, the position of the TJC in the abdomen ACSs is first obtained by a 3D interpolation based on literature data. To allow a functional application of the scaling equations, the regression for the position of the TJC based on anatomical landmarks (C7, SUP, T8, T12) is provided for females and extends the previous estimations available for males proposed by Reed et al. (Reed et al. Citation1999). The regressions for the positions of the CJC, TJC, LJC, as well as the shoulder joint centres (SJCs) for both males and females proposed in Reed et al. (Citation1999), completed in the previous (Dumas et al. Citation2007a) and the present adjustment procedures, represent a unique solution for a thorough torso definition.
Definitions of the segment coordinate systems
The abdomen segment has not been defined in the recommendations of the ISB (Wu et al. Citation2002, 2005). In the present study, consistent with the other segments, the Y axis was defined using the proximal and distal joint centres. To define the X and Z axes, McConville et al. (Citation1980) and Young et al. (Citation1983) have used specific anatomical landmarks on the spine and the ribs (MSR10, RR10, and LR10) but these anatomical landmarks are not widely used in movement analysis. When applying the scaling equations to estimate the BSIPs of a given subject, one solution is to assume that no axial rotation exists at the LJC between the pelvis and the abdomen. This is also consistent with some hypotheses that were made for the adjustment procedure described above. The thorax and abdomen SCSs were defined with the TJC and LJC joint centres that could be estimated in only one ACS with the available data in McConville et al. (Citation1980) and Young et al. (Citation1983). The transformations from one ACS to the other were determined assuming that the sagittal planes of both abdomen and pelvis ACSs (as well as SCSs) were coincident and that the rotations in this plane were the same for males and females.
Updated scaling equations
In the updated scaling equations, the abdomen segment represents only 3–4% of total body mass because it corresponds to the part of the trunk below the 10th ribs (i.e. at the level of the 1st lumbar vertebrae) and above the iliocristales (i.e. at the level of the 4th lumbar vertebrae). This differs from other scaling equations, e.g. the middle part of the trunk of de Leva (de Leva Citation1996) as this segment corresponds to the part of the trunk below the xyphoid process and above the navel. Apart from the segmentation, the main difference with other scaling equations for the trunk found in the literature is the additional information provided for the anterior–posterior position of the COM in the thorax and abdomen SCSs (e.g. not available in [de Leva Citation1996; Wicke et al. Citation2009]) and for the products of inertia (e.g. not available in [de Leva Citation1996; Pearsall et al. Citation1996; Erdmann Citation1997; Wicke et al. Citation2009]).
The proposed thorax and abdomen scaling equations may be useful for researchers who would like to use the BSIPs adjusted from McConville et al. (Citation1980) and Young et al. (Citation1983) with a more appropriate trunk definition. A preliminary application to sit-to-stand movements demonstrates that using two thorax and abdomen segments rather than one torso segment may improve the results of inverse dynamics. It is currently difficult to anticipate if the updated scaling equations of thorax and abdomen could also change the results of the studies that have evaluated the adjusted BSIPs with a torso segment in other movements (Robert et al. Citation2007; Pillet et al. Citation2010; Iino & Kojima Citation2012; Hansen et al. Citation2014). Further evaluations of the scaling equations of the thorax and abdomen segments are still required.
Disclosure statement
No potential conflict of interest was reported by the authors.
References
- Dumas R, Cheze L. 2009. Soft tissue artifact compensation by linear 3D interpolation and approximation methods. J Biomech. 42:2214–2217.10.1016/j.jbiomech.2009.06.006
- Dumas R, Chèze L, Verriest JP. 2007a. Adjustments to McConville et al. and Young et al. body segment inertial parameters. J Biomech. 40:543–553.10.1016/j.jbiomech.2006.02.013
- Dumas R, Chèze L, Verriest JP. 2007b. Corrigendum to “Adjustments to McConville et al. and Young et al. body segment inertial parameters” [J. Biomech. 40 (2007) 543–553]. J Biomech. 40:1651–1652.10.1016/j.jbiomech.2006.07.016
- Erdmann WS. 1997. Geometric and inertial data of the trunk in adult males. J Biomech. 30:679–688.10.1016/S0021-9290(97)00013-4
- Erdmann WS. 1999. Geometry and inertia of the human body – review of research. Acta Bioeng Biomech. 1:23–35.
- Hansen C, Venture G, Rezzoug N, Gorce P, Isableu B. 2014. An individual and dynamic body segment inertial parameter validation method using ground reaction forces. J Biomech. 47:1577–1581.10.1016/j.jbiomech.2014.03.004
- Hinrichs RN. 1990. Adjustments to the segment center of mass proportions of Clauser et al. (1969). J Biomech. 23:949–951.10.1016/0021-9290(90)90361-6
- Iino Y, Kojima T. 2012. Validity of the top-down approach of inverse dynamics analysis in fast and large rotational trunk movements. J Appl Biomech. 28:420–430.
- Jensen RK. 1993. Human morphology: its role in the mechanics of movement. J Biomech. 26:81–94.10.1016/0021-9290(93)90081-O
- de Leva P. 1996. Adjustments to Zatsiorsky-Seluyanov’s segment inertia parameters. J Biomech. 29:1223–1230.10.1016/0021-9290(95)00178-6
- McConville JT, Churchill TD, Kaleps I, Clauser CE, Cuzzi J. 1980. Anthropometric relationships of body and body segment moments of inertia. Wright-Patterson Air Force Base, Dayton, OH. (Technical report AFAMRL-TR-80-119).
- Pearsall DJ, Reid G. 1994. The study of human body segment parameters in biomechanics. Sports Med. 18:126–140.10.2165/00007256-199418020-00005
- Pearsall DJ, Reid JG, Livingston LA. 1996. Segmental inertial parameters of the human trunk as determined from computed tomography. Ann Biomed Eng. 24:198–210.10.1007/BF02667349
- Pillet H, Bonnet X, Lavaste F, Skalli W. 2010. Evaluation of force plate-less estimation of the trajectory of the centre of pressure during gait. Comparison of two anthropometric models. Gait Posture. 31:147–152.10.1016/j.gaitpost.2009.09.014
- Reed MP, Manary MA, Schneider LW. 1999. Methods for measuring and representing automobile occupant posture. Warrendale, PA. (SAE Technical Paper Series: 1999-01-0959).10.4271/1999-01-0959
- Reid JG, Jensen RK. 1990. Human body segment inertia parameters: a survey and status report. Exerc Sport Sci Rev. 18:225–242.
- Robert T, Chèze L, Dumas R, Verriest JP. 2007. Validation of net joint loads calculated by inverse dynamics in case of complex movements: application to balance recovery movements. J Biomech. 40:2450–2456.10.1016/j.jbiomech.2006.11.014
- Robert T, Causse J, Wang X. 2011. Dynamics of sit-to-stand motions: effect of seat height, handle use and asymmetrical motions. Comput Methods Biomech Biomed Engin. 14:191–192.10.1080/10255842.2011.594720
- Schneider LW, Robbins DH, Pflug MA, Snyder RG. 1983. Anthropometry of motor vehicle occupants. Volume 2: anthropometric specifications for mid-sized male dummy. Volume 3: anthropometric specifications for small female and large male dummies. Ann Arbor, MI. (Technical report UMTRI-83-53-2 / 3).
- Trochu F. 1993. A contouring program based on dual Kriging interpolation. Eng Comput. 9:160–177.10.1007/BF01206346
- Wicke J, Dumas GA, Costigan PA. 2009. A comparison between a new model and current models for estimating trunk segment inertial parameters. J Biomech. 42:55–60.10.1016/j.jbiomech.2008.10.003
- Wu G, Siegler S, Allard P, Kirtley C, Leardini A, Rosenbaum D, Whittle M, D’Lima DD, Cristofolini L, Witte H, et al. 2002. ISB recommendation on definitions of joint coordinate system of various joints for the reporting of human joint motion–part I: ankle, hip, and spine. J Biomech Apr;35:543–548.
- Wu G, van der Helm FCT, Veeger HEJ, Makhsous M, Van Roy P, Anglin C, Nagels J, Karduna AR, McQuade K, Wang X, et al. 2005. ISB recommendation on definitions of joint coordinate systems of various joints for the reporting of human joint motion – Part II: shoulder, elbow, wrist and hand. J Biomech. 38:981–992.10.1016/j.jbiomech.2004.05.042
- Young JW, Chandler RF, Snow CC, Robinette KM, Zehner GF, Lofberg MS. 1983. Anthropometric and mass distribution characteristics of the adults female. Oklahoma City, OK. ( Technical Report FA-AM-83-16).
The 3D position of the TJC in the abdomen ACS as defined by McConville et al. (Citation1980) and Young et al. (Citation1983) is obtained from its position
in a laboratory coordinate system as defined by Schneider et al. (Schneider et al. Citation1983), knowing the 3D position of n other anatomical landmarks
:
with the Euclidean distances between the TJC (point p) and the other points q. The 3D interpolation parameters (a and b) are determined by resolving a square system of linear equations for the n anatomical landmarks (whose positions
and
are known both in the coordinate systems as defined by McConville et al. (Citation1980) and Young et al. (Citation1983) and in the coordinate systems as defined by Schneider et al. (Schneider et al. Citation1983), respectively):
with ,
,
,
and where the elements (q1, q2) of the matrix K(n×n) are the Euclidean distances
.