ABSTRACT
Poor published data are available for the detections of toxic phenolic compounds in the Egyptian Mediterranean Coast. Seasonal and spatial distributions of eight driven toxic phenolic compounds in coastal waters of Alexandria from ten different locations, situated between Abu Qir bay and Eastern Harbor during July, December (2018), and February, April (2019), were investigated to determine contamination status, possible sources, and ecological risks in the study area. For that purpose, a method was optimized for derivatization and detection of methylphenols, chlorophenols, and nitrophenols in water samples using GC-MS. This analytical method was validated using matrix spikes, indicating detection limits (0.02–0.5 µg/ml) and good recoveries (70–120%). Ranges of phenol concentrations in water samples were as follows: phenol (<LOD-109.2 ng/l), 2,4-DMP (<LOD-117.68 ng/l), 2-nitophenol (<LOD −87.09 ng/l), 2,4 dichlorophenol (19.93–66.30 ng/l), pentachlorophenol (13.50–60.08 ng/l), 2-chlorophenol (13.23–91.43 ng/l), 2,4,6-trichlorophenol (12.9–46.04 ng/l), and 4-chloro-3-methylphenol (15.2–52.3 ng/l). Methylphenols were the major group detected followed by nitrophenols and chlorophenols. Results of the risk assessment revealed that concentrations of the studied phenols were within the safe limits and lower than Surface Water Toxicity Reference Values (TRVs), giving no indication of pollution. Multivariate statistical analysis with different physicochemical parameters of water indicated the greatly variable behavior of phenolic compounds, and the exact sources of these compounds are difficult to identify since no major point sources of pollution are known in the area of study.
Introduction
Alexandria is Egypt’s largest city on the Mediterranean coast and the most important industrial centers, comprising 100 large factories and about 260 smaller ones (Abd-Allah, Citation1993), to cover about 40% of the nation’s industry. It is the main summer resort in Egypt, with about 4 million citizens with 2 to 4 million visitors during the summer months (Nasr, Citation1995). Alexandria’s coastal zone is about 42 km long, extending from Abu Qir in the east to El-Dekhaila in the west, and consists of pockets and embayment beaches morphology. Alexandria coastal zone is a major tourist attraction for their clear waters and coastal habitats, and is vital for fisheries and marine activities. However, the coasts are under constant threat of pollution in the form of untreated domestic sewage from the different human and marine activities in addition to agricultural, and industrial wastewater discharges.
Phenols are important contaminants in surface and ground waters, usually used in daily life. Chlorophenols are carcinogenic and can affect the odor and taste of drinking water with concentrations as low as a few µg/l (Puig & Barcelo, Citation1996). Phenols of anthropogenic origin exist in the environment in domestic and industrial wastewaters, natural waters, and potable water supplies as a result of their wide use in numerous commercial products including pesticides, wood preservatives, bactericides, fungicides and herbicides, polymers, dyes, and by-products of industrial processes, such as petrochemical industries (National Research Council of Canada, Citation1982). These compounds penetrate ecosystems as the result of drainage off the municipal or industrial sewage to surface water. Some phenols are formed during natural processes like the formation of phenol and p-cresol during decomposition of organic matter or synthesis of chlorinated phenols by fungi and plants (Swarts, Verhagen, Field, & Wijnberg, Citation1998). Due to their persistence and toxicity in the environment, both the US Environmental Protection Agency (EPA) and the European Union (EU) have included chlorophenols and nitrophenols in their lists of priority pollutants. Phenol analyses have been reported in several matrices such as human urine (Bartels et al., Citation1999; Crespin, Gallego, & Valcarcel, Citation2002), wastewater (Moder, Schrader, Franck, & Popp, Citation1997), seawater (Jauregui, Moyano, & Galceran, Citation1997), sediments, and others (Takasu, Iles, & Kiyoshi, Citation2002).
For the analysis of phenols, many analytical methods have been used, using gas chromatography (GC) with various detectors or high-performance liquid chromatography (HPLC) (Bagheri & Saraji, Citation2001). Underivatized phenols may lose and lead to peak tailing due to irreversible adsorption on active centers of the separation system (Kovacs et al., Citation2008). Many derivatization methods have been proposed; the most popular as chemical reagents were acetylating agents (Bartak & Cap, Citation1997; Turnes, Rodrıguez, Mejuto, & Cela, Citation1994) because of direct acetylation of phenols in aqueous phase. Extraction process and other steps are always necessary prior to their instrumental detection to detect the phenol levels in environmental samples. To accomplish this difficult task in water and sediment, several methods have been developed including liquid–liquid extraction (LLE), solid-phase microextraction (SPME), and solid-phase extraction (SPE). However, when analyzing both free and bound phenols in the case of nitrophenols and chlorinated phenols, most of these methodologies fail (Alonso, Puig, Silgoner, Grasser Bauer, & Barcelo, Citation1998).
Although trace organic pollutants along the Egyptian Mediterranean Sea have been investigated by numerous workers (Abbassy, Citation2000; Abd-Allah & Abbas, Citation1994; Abd-Allah & EL-Sebae, Citation1995; Barakat, Citation2001, Citation2004; Barakat, Mostafa, Wade, Sweet, & Badr, Citation2010; El-Naggar et al.,Citation2018; El-Naggar et al.,Citation2019), poor published data are detected for the levels of phenolic compounds in the aquatic environment of Egypt particularly, coast of Alexandria; only Khairy (Citation2013) and El-Naggar (Citation2013) published data on phenol compounds in water and sediments of Lake Maryut and El-Mex Bay. Therefore, the aim of the current study was to determine the contamination status, possible sources, and ecological risks of phenolic compounds from ten locations along the Alexandria coastal water from Abu Qir Bay to Eastern Harbor.
Materials and methods
Study area and sampling
Seasonally, water samples were collected from July, December 2018, February, and April 2019 at ten beaches: the Abu-Qir Bay, El-Montazah, El-Asafra, Miami, Sidi Bichr, Glym, Stanly, Sidi Gaber, Chatby, and Eastern Harbor along Egyptian Mediterranean coast (Alexandria). The examined beach area was selected to cover the expected polluted areas (Abu-Qir Bay, Eastern Harbor). The Eastern Harbor, semi-closed basin, is considered as a model of polluted sea water bodies mainly affected by sewage disposal beside its ship-yard waste disposal (Khaled, Citation2004a; Shriadah & Emara, Citation1991) while the Abu-Qir Bay is exposed to industrial and agricultural wastes (El-Tawil and Khalil, Citation1983), as well as unpolluted regions (El-Montazah, El-Asafra, Miami, Sidi Bichr, Glym, Stanly, Sidi Gaber, Chatby) are affected by the water current from the west to the east ().
After collection of samples, water samples were filtered through Millipore glass fiber filters, preserved by addition of 0.1 g ascorbic acid and 10 ml methanol, then stored at 4°C and finally immediately transported to the laboratory.
Chemicals and reagents
EPA 8040 A phenol calibration mixture (500 μg/ml each component in iso-propanol) was of analytical grade and purchased from Sigma-Aldrich, USA, containing phenol (Ph), 2,4-dimethylphenol (2,4-DMP), 2-chlorophenol (2-CP), 2,4 dichlorophenol (2,4-DCP), 2,4,6-trichlorophenol (2,4,6-TCP), pentaclorophenol (PCP), 4-chloro-3-methylphenol (4-Cl-3-MP), and 2-nitophenol (2-NP). The working solutions were prepared by an appropriate dilution in iso-propanol. All the used solvents were HPLC grade and purchased from Supelco (Bellefonte, USA). 2,4,6-Tribromophenol was dissolved in methanol and used as surrogate standard (SUSTD).
Water extraction
Each water sample (1 L) was spiked with the SUSTD and extracted three times at a pH equal to 2 with dichloromethane (100 mL each) using 2 L separating funnel. Extracts were evaporated under a gentle stream of nitrogen to a final volume of 1 ml (EPAMethod 604 (Citation1984), EPA Method 625 (Citation1984), EPA Method 8041 (Citation1995)).
Clean up with column chromatography
Column chromatography was used to clean up water extracts according to EPA Method 604 (Citation1984). Column chromatography (2 cm x 30 cm) was plugged with a piece of glass wool, packed with 4.0 g of silica gel (60 neutral, 230–400 mesh previously activated at 130°C overnight) topped with 2 g anhydrous sodium sulfate, and conditioned with about 6 ml n-hexane. The extract was added to the column. Afterward, the compounds were eluted with 10 ml n-hexane and discarded and then eluted with 10.0 ml of 15% toluene in hexane (Fraction 1); 10.0 ml of 40% toluene in hexane (Fraction 2); 10.0 ml of 75% toluene in hexane (Fraction 3); and 10.0 ml of 15% 2-propanol in toluene (Fraction 4). Finally, all fractions were combined and evaporated, and the solvent was exchanged with iso-propanol to a final volume of 1 ml.
Phenolic compounds derivatization
GC is a popular technique for the analysis of phenol compounds. However, because of their high polarity, phenols tend to give broad, tailed peaks, and the effect increases as the chromatographic column ages. To avoid this drawback, several derivatization reactions have been proposed to transform phenols to less polar compounds, with better chromatographic characteristics. Acetylation of phenolic compounds with acetic anhydride in the presence of carbonate is one of the most studied derivatization procedures (Renberg & Lindstrom, Citation1981), with some modifications. Three milligram of potassium carbonate and 0.5 ml of acetic anhydride were added to 1.0 ml of sample extract and shaken gently after each addition using a vortex mixer. The mixture was heated for 30 min at 80°C in a hot water bath, then removed from the water bath and cooled. The solution was extracted twice using 3 ml of 5% of potassium carbonate and 2 ml of hexane and shaken vigorously for several minutes using a vortex mixer. After phase separation, the solvent was collected and the water phase was extracted with 2 ml of hexane. Finally, the extracts were concentrated using nitrogen gas to a final volume of 1.0 ml.
Instrumental analysis
The chromatographic analysis of phenolic compounds was performed by gas chromatography-mass spectrometer; GC-MS (Agilent model 7890A- 5975, USA) equipped with auto-sampler and a fused-silica capillary column; DB 5 MS (30 m, 0.25 mm, 0.25 μm) with 35% phenyl polysilphenylene-siloxane. For 2 min, the instrument was operated in the splitless mode then the purge flow was maintained at 50 ml/min. The injection port temperature was maintained at 260°C. The injection volume was 1 μL. The oven temperature was maintained at 60 °C for 1 min then increased to 80 °C at the rate of 5 °C /min and to 210 °C at the rate of 10 °C /min. Finally, the temperature reached 280 °C. Helium was the carrier gas (flow rate, 0.9 ml/min). Data of mass spectra and retention time of the acetylated derivatives were obtained in the full scan mode (50–550 m/z). The selective ion monitoring system (SIM) is used to evaluate the separated compounds: three characteristic ions per compound were selected and scanned. Chromatograms of GC-MS were evaluated using relative peak areas of standard. Other phenolic compounds were identified in some samples using published mass spectra and relative retention time. Total ion chromatogram (TIC) of phenol standard solutions is shown in . Standard solutions between 0.01 and 5.00 μg/ml were used to establish calibration curves. In the calibration curves, the correlation coefficients were all above 0.98. For standard solutions, blanks were run to check for contamination and interferences. The laboratory results of phenolic compounds showed lower detection limits from 0.02 to 0.5 µg/ml and recoveries of 70–120%.
Statistical analysis
IBM SPSS Statistics program version 19 is used to perform statistical analysis, including the multivariate statistical analysis, between the different physicochemical parameters: temperature, salinity, pH, dissolved oxygen, oxidizible organic matter, and biological oxygen demand for testing the significance of correlation between them. Factor analysis is also performed and used to reduce the dimension of the matrix by creating new variables, called factors, which have some advantages over measured variables. Factor analysis was applied to the data of phenolic compounds in seawater in order to verify pollution sources as well as to illustrate the distribution of samples in a two-dimensional plot.
Results and discussion
Concentrations of total and individual phenols, including PH, 2-CP, 2,4,6-TCP, PCP, 2-NP, 2,4-DCP, 4-Cl-3-MP, 2,4-DMP in Alexandria coastal waters from ten different locations, situated between Abu Qir bay and Eastern Harbor from July 2018 to April 2019, are given in . Average total phenols in water ranged from minimum of 30.33 ng/l (Miami) to a maximum of 47.07 ng/l (El-Montazah) (), showing irregular distribution. Average high concentrations (>35 ng/l) were observed at near-shore coastal waters of El-Montazah, El-Asafra, Sidi Bichr, Sidi Gaber, Chatby, and Eastern Harbor.
Table 1. Concentrations of phenolic compounds in coastal water of the area of study during 2018–2019
Figure 3. Distribution of total concentrations of phenolic compounds (ng/l) in water samples of Alexandria coastal areas during 2018–2019.
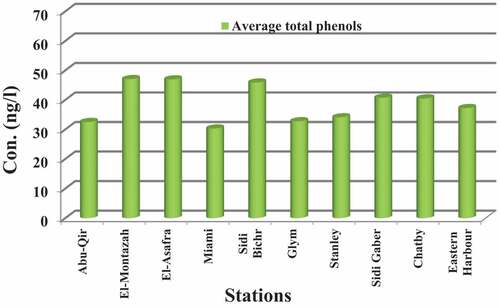
Two principal factors influenced this distribution: water currents and anthropogenic factors due to the tourism boats and ship activities. These activities lead to the accumulation of these compounds through adsorption on the surface of suspended matter and its high resistance to degradation.
Relatively low concentrations (<35 ng/l) were observed at Abu-Qir, Miami, Glym, and Stanly (). Higher concentrations of phenol, CPs, 2NP, 2,4-DMP,and 4-Cl-3-MP with 109.2, 91.4, 63.5, 117.7, and 34.4 ng/l were recorded during July 2018, affecting higher tourist activities during summer 2018.
Many organic compounds from natural and anthropogenic origin may be present in the surface bodies of water. Different natural factors or kinds of human activities can affect the composition of the particular compounds. The occurrence of chlorophenols in the marine environment is strictly related to human activities such as industry, production and degradation of pesticides, and sewage discharges to water (Martins, Ferreira, Santos, Queiroz, & Lima, Citation2001; Oberg, Citation2004). Simultaneously, the natural sources of phenols that stem from production of phenols and chlorophenols in soil and water by plants, fungi, and bacteria are often ignored. If the ecosystem is not exposed to contamination, the above factors may play the most important role.
2,4-DMP was the most dominant compounds in water samples of the study area (<LOD −117.68 ng/l) with an average concentration of 52.5 ng/l, representing from 7% to 22.5% of the total detected phenols, with higher concentrations at El-Montazah (). This compound of low molecular weight commonly exists in the environment in considerable concentrations and formed during coal and gasoline combustion and lubricating oils and, sometimes, forms during geochemical processes like methylation that proceed in geological structures (Kahl, Makynen, Kosi, & Ankley, Citation1997). This is confirmed by Ahmed, Mahmoud, and El Nady (Citation2017) who found 3631.284 ng/L of Benzo [ghi] perylene (BP) in the water samples of Alexandria coastal that has the fingerprint of a combustion engine.
Figure 4. Annual average of phenol compounds in coastal water of the area of study during 2018–2019.
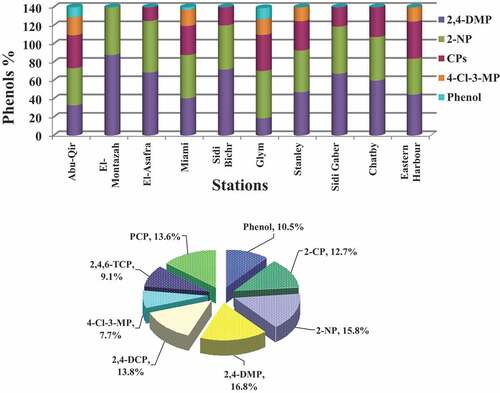
The second group determined in water samples was NP (<LOD −87.09 ng/l), recording also high concentrations at most stations (). CPs (<LOD-109.2 ng/l) came in the third order after MPs and NPs and were dominated by 2,4-DCP (19.93–66.30 ng/l), followed by PCP (13.50–60.08ng/l), 2-CP (13.23–91.43ng/l), and finally 2,4,6-TCP (12.9–46.04 ng/l). CP concentrations were significantly higher at El-Montazah, Miami, Glym, Stanly, Chatby, and Eastern Harbor (13.8–79.9 ng/l) and dominated by 2-CP, 2,4-DCP, 2,4,6-TCP and PCP, that are usually used as pesticides, insecticides, and fungicides (Czaplicka, Citation2004). In addition, 4-Cl-3-MP was also detected at these locations in higher concentrations (15.2–52.3 ng/l). 4-Cl-3-MP may be produced by the chlorination of drinking of wastewater. Transformation of chlorophenols in water during dechlorination processes usually leads to the formation of phenol (Juteau et al., Citation1995). Finally, phenol (<LOD-109.2 ng/l) came in the last order after MPs, NPs, and CPs and is used in dyes and textiles production ().
From the above results, we can conclude that water currents and anthropogenic factors due to the tourism boats’ and ships’ activities that adsorbed these compounds on the surface of suspended matter and its high resistance to degradation are the major source of pollution by phenolic compounds near-shore Alexandria coast of Egypt. The average concentrations of phenols in Alexandria coast were 43.9, 44.04, 29.5, and 36.5 ng/l during summer, autumn (2018), winter, and spring (2019), respectively (). The maximum concentration of phenols was recorded at El-Montazah, El-Asafra, Sidi Bichr, Sidi Gaber, Chatby, and Eastern Harbor.
indicates that all measured values of phenolic compounds were far below than that recorded worldwide. For assessment of the level of phenolic compounds in the coastal water of Alexandria, detected concentrations of phenols were compared with Surface Water Toxicity Reference Values (TRVs), which are used in the Ecological Risk Assessment (ERA) process as part of the Ecological Constituent of Potential Concern (COPC) Selection Process (WSRC, Citation1999). Ecological screening levels for only eight different phenols are given in (EPA, Citation1995).
Table 2. Concentrations of phenols (μg/l) in the present study compared with other areas worldwide
Table 3. Concentrations of phenolic compounds and its descriptive statistics of Alexandria coastal water
indicates that concentrations of phenol compounds are much lower than TRVs and not regarded as contaminants of potential concern in coastal water of Alexandria.
indicates that a strong significant correlation was found between 2,4-DMP and ∑PHs (r = 0.847) and between PH and ∑PHs (r = 0.826), reflecting that 2,4-DMP is the main factor of increasing phenolic compounds and also can be converted to PH. This is confirmed by significant correlation between 2,4-DMP and PH (r = 0.68). A significant correlation was found between phenol and pH (r = 0.463), reflecting the effect of pH only on phenol, and the behavior of the organic compounds was greatly variable.
Table 4. Correlation matrix of phenolic compounds and physicochemical parameters collected from coastal water of Alexandria during 2018–2019
Results of factor analysis of the phenolic compounds data (n = 39) indicates three factors that can be related to various controlling processes. shows the varimax rotated factor matrix consisting of the component factors, variables loading on each factor, and percentage of data variance explained by each factor. These three factors account for 71.27% of the total variance in the dataset.
Table 5. Varimax rotated factor loading matrix for phenolic compounds data in the present study
Factor 1 has a high positive loading of PH and 2,4-DMP and explains 30.29% of the total variance ( and ). This factor is clearly related with compounds characterized by low molecular weights that commonly exist in the environment in considerable concentrations, and this is confirmed by significant correlation between 2,4-DMP and PH (r = 0.68).
Figure 5. Scatter plot of factor loadings (after Varimax rotation) for the investigated compounds in the study area.
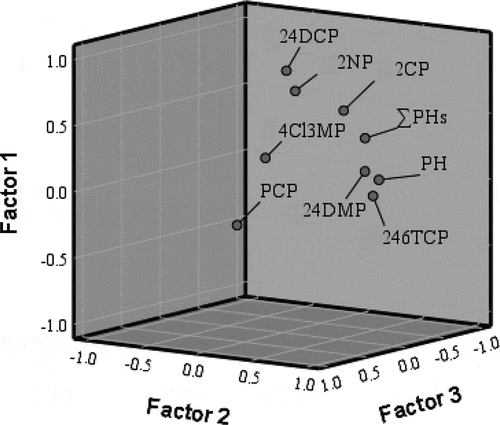
Factor 2, which explains 23.57% of the total variance, includes 2-CP, 2-NP, and 2,4-DCP (). The positive contribution can be associated to higher concentration of these compounds in the area of study. Factor 3 explains 17.40% of the total variance and includes 2,4,6-TCP and PCP. The positive contribution can be associated to a predominant chlorination of phenol under different conditions. The positive contribution to the second and third principal component analysis is accounted for new anthropogenic origin compounds coming from land-based sources: different industrial origin (phenols) especially chloro-phenols. These results agree with El-Naggar (Citation2013).
The Egyptian Mediterranean coast receives huge volumes of wastewaters every year through the coastal lagoons and from other land-based effluents. The continuous discharges polluted water, caused massive development of algal blooms, and gradually deteriorated the water quality (Zakaria, Radwan, & Said, Citation2007). The presence of phenolic compounds may be due to the degradation or decomposition of natural organic matter present in the water, these chemicals, upon entry into the water, have the tendency of undergoing transformations into other moieties that can even be more harmful than the original compounds. This transformation is normally due to their interaction with physical, chemical, and biological or microbial factors in the water (Kulkarni & Kaware, Citation2013). So, the exact sources of this contamination are difficult to identify since no major point sources of pollution are known in the area of study.
Conclusion
The rapid increase in industrial and domestic activities as a result of the desire to meet the demands of the ever‐increasing human population creates the possibility of phenolic compounds introduction into water bodies. This paper represents the first comprehensive survey of phenolic compounds and provides important data on eight selected phenolic compounds, contamination status, possible sources, and ecological risks in the shoreline of Egyptian Mediterranean Sea coast (Alexandria coastal seawater).
Optimized method was used for derivatization and determination of methylphenols, chlorophenols, and nitrophenols in water samples using GC-MS, which indicated good detection limits and good recoveries. 2,4-DMP was more frequently detected phenol followed by nitrophenols and chlorophenols. The maximum concentrations of phenols were recorded at El-Montazah, El-Asafra, Sidi Bichr, Sidi Gaber, Chatby, and Eastern Harbor. Results of the risk assessment revealed that concentrations of the studied phenols were within the safe limits and lower than Surface Water Toxicity Reference Values, giving no indication of pollution. Multivariate statistical analysis with different physicochemical parameters of water indicated the greatly variable behavior of the phenolic compounds, and the exact sources of these compounds are difficult to identify since no major point sources of pollution are known in the area of study.
Disclosure statement
No potential conflict of interest was reported by the author(s).
References
- Abbassy, M. S. (2000). Pesticides and polychlorinated biphenyls drained into north coast of the Mediterranean Sea, Egypt. Bulletin of Environmental Contamination and Toxicology, 64(4), 508–517. doi:10.1007/s001280000033
- Abd-Allah, M., 1993. Concentration of mercury in fresh, brackish and saline water in Alexandria region. M.Sc. Thesis, Inst. of Grad. Stud. and Res., Alex. Univ., Egypt, 145 pp.
- Abd-Allah, A. M., & Abbas, M. M. (1994). Residue levels of organochlorine pollutants in the Alexandria Region, Egypt. Toxicological & Environmental Chemistry, 41(3–4), 239–247. doi:10.1080/02772249409357979
- Abd-Allah, M. A., & EL-Sebae, A. K. (1995). Hydrocarbon contamination of the Egyptian Mediterranean Coast. Toxicological & Environmental Chemistry, 47(1–2), 15–22. doi:10.1080/02772249509358122
- Ahmed, O. E., Mahmoud, S. A., & El Nady, M. M. (2017). Organic sources in the Egyptian seawater around Alexandria coastal area as integrated from polycyclic aromatic hydrocarbons (PAHs. Egyptian Journal of Petroleum, 26(3), 819–826. doi:10.1016/j.ejpe.2016.10.016
- Alonso, M. C., Puig, D., Silgoner, I., Grasser Bauer, M., & Barcelo, D. (1998). Determination of priority phenolic compounds in soil samples by various extraction methods followed by liquid chromatography-atmospheric pressure chemical ionization mass spectrometry. Journal of Chromatography A, 823(1–2), 231–239. doi:10.1016/S0021-9673(98)00110-1
- Bagheri, H., & Saraji, M. (2001). New polymeric sorbent for the solid-phase extraction of chlorophenols from water samples followed by gas chromatography-electron-capture detection. Journal of Chromatography A, 910(1), 87–93. doi:10.1016/S0021-9673(00)01165-1
- Barakat, A. O. (2001). PAHs and petroleum markers in the atmospheric environment of Alexandria City, Egypt. Water, Air, & Soil Pollution, 189, 289–310.
- Barakat, A. O. (2004). Assessment of persistent toxic substances in the environment of Egypt. Environment International, 30(3), 309–322. doi:10.1016/S0160-4120(03)00181-8
- Barakat, A. O., Mostafa, A. R., Wade, T. L., Sweet, T., & Badr, N. (2010). Spatial distribution and temporal trends of polycyclic aromatic hydrocarbons in sediments from Lake Maryut, Alexandria, Egypt. Water, Air, & Soil Pollution, 218(1–4), 63–80. doi:10.1007/s11270-010-0624-5
- Bartak, P., & Cap, L. (1997). Determination of phenols by solid phase microextraction. Journal of Chromatography A, 767(1–2), 171–175. doi:10.1016/S0021-9673(96)01090-4
- Bartels, P., Ebeling, E., Kramer, B., Kruse, H., Osius, N., Vowinkel, K., … Zorn, C. (1999). Determination of chlorophenols in urine of children and suggestion of reference values. Fresenius’ Journal of Analytical Chemistry, 365(5), 458–464. doi:10.1007/s002160051640
- Crespin, M. A., Gallego, M., & Valcarcel, M. (2002). Solidphase extraction method for the determination of free and conjugated phenol compounds in human urine. Journal of Chromatography B, 773(2), 89–96. doi:10.1016/S1570-0232(02)00012-0
- Czaplicka, M. (2004). Sources and transformations of chlorophenols in the natural environment. Science of the Total Environment, 322(1–3), 21–39. doi:10.1016/j.scitotenv.2003.09.015
- Dimou, A., Sakellarides, T. M., Vosniakos, F. K., Giannoulis, N., Leneti E. & Albanis, T. (2006). Determination of phenolic compounds in the marine environment of Thermaikos Gulf, Northern Greece. International Journal of Environmental Analytical Chemistry, 86(1–2), 119–130. doi:10.1080/03067310500249963
- El-Naggar, N. A., 2013. Studies on some disposed toxic organic contaminants into Mex-Bay and its adjacent areas, Alexandria-Egypt. Ph.D. Thesis, Faculty of Science. Al-Azhar Univ., Egypt (Unpublished).
- El-Naggar, N. A., Emara, H. I., Moawad, M. N., Soliman, Y. A., & El-Sayed, A. A. (2018). Detection of PAHs along Alexandria coastal water, Egyptian Mediterranean Sea. The Egyptian Journal of Aquatic Research, 44(1), 9–14. doi:10.1016/j.ejar.2018.02.003
- El-Naggar, N. A., Mohamed, L. A., Abdel-Halim, A., & Emara, H. E. (2019). Environmental Characteristics of the Egyptian Mediterranean Coast. Egyptian Journal of Aquatic Biology and Fisheries, 23(2), 475–490. doi:10.21608/ejabf.2019.34027
- El-tawil, B.A.H., & Khalil, A.N. (1983) Chemical Constituents of Some Algal Species from Abu-Qir Bay, Egypt. Journal of Factuality of Marine Science, 3, 1404H.
- EPA, 1995. Supplemental guidance to RAGS: Region 4 Bull. Ecolo. Risk Assess. (http://www.epa.gov/region4/wastepgs/oftecser/otsguid.htm).
- EPA Method 604. (1984, October 26). Phenols, Part VIII, 40 CFR Part 136 (pp. 58). Washington, DC: Environ. Prot. Agency.
- EPA Method 625. (1984, October 26). Base/ Neutrals and Acids, Part VIII, 40 CFR Part 136 (pp. 153). Washington, DC: Environ. Prot. Agency.
- EPA Method 8041. (1995). Phenols by Gas Chromatography: Capillary Column Technique (pp. 1). Washington, DC: Environ. Prot. Agency.
- Jauregui, O., Moyano, E., & Galceran, M. T. (1997). Liquid chromatography-atmospheric pressure ionization mass spectrometry for the determination of chloro and nitro phenolic compounds in tap water and sea water. Journal of Chromatography A, 787(1–2), 79–89. doi:10.1016/S0021-9673(97)00653-5
- Juteau, P., Beaudet, R., McSween, G., Lepine, F., Milot, S., & Bisaillon, J. G. (1995). Anaerobic biodegradation of pentachlorophenol by a methanogenic consortium. Applied Microbiology and Biotechnology, 44(1–2), 218–224. doi:10.1007/BF00164505
- Kahl, M., Makynen, E., Kosi, A. P., & Ankley, G. (1997). Toxicity of 4-nonylphenol in a life – Cycle test with the midge Chironomus tentaus. Ecotoxicology and Environmental Safety, 38(2), 155. doi:10.1006/eesa.1997.1572
- Khairy, A. M. (2013). Assessment of priority phenolic compounds in sediments from an extremely polluted coastal wetland (Lake Maryut, Egypt. Environmental Monitoring and Assessment, 185(1), 441–455. doi:10.1007/s10661-012-2566-4
- Khaled, A. (2004a). Heavy metals concentrations in certain tissues of five commercially important fishes from El-Mex Bay, Alexandria, Egypt. Egyptian Journal of Aquatic Biology and Fisheries, 8(1), 51–64.
- Kovacs, A., Kende, A., Mortl, M., Volk, G., Rikker, T., & Torkos, K. (2008). Determination of phenols and chlorophenols as trimethylsilyl derivatives using gas chromatography–mass spectrometry. Journal of Chromatography A, 1194(1), 139–142. doi:10.1016/j.chroma.2008.04.043
- Kulkarni, S. J., & Kaware, D. J. P. (2013). Review on research for removal of phenol from wastewater. International Journal of Scientific and Research Publication, 3, 1–4.
- Martins, M. A., Ferreira, I. C., Santos, I. M., Queiroz, M. J., & Lima, N. (2001). Biodegradation of bioaccessible textile azo dyes by Phanerochaete chrysosporium. Journal of Biotechnology, 89(2–3), 91–98. doi:10.1016/S0168-1656(01)00318-2
- Michalowicz, J., Bukowska, B., & Duda, W. (2008). The differences in phenolic content in rivers exposed and non-exposed to anthropogenic contamination, Chemosphere, 71, 735–741.
- Moder, M., Schrader, S., Franck, U., & Popp, P. (1997). Determination of phenolic compounds in waste water by solid phase micro extraction. Fresenius Journal of Analytical Chemistry, 357(3), 326–332. doi:10.1007/s002160050162
- Nasr, S. M., 1995. Geochemistry and granulometric normalization for heavy metals in the bottom sediments off Alexandria, Egypt. Proceeding of the 2nd Conference on the Mediterranean Coastal Environment. MEDCOAST 95, Tarragona, Spain, 1473–1481.
- National Research Council of Canada. (1982). NRC Associate Committee on Scientific Criteria for Environmental Quality, Chlorinated Phenols: Criteria for Environmental Quality, Public No. 18578. Ottawa, Microfiche.
- Oberg, T. (2004). Halogenated aromatics from steel production: Results of pilot-scale investigation. Chemosphere, 56(5), 441–448. doi:10.1016/j.chemosphere.2004.04.038
- Puig, D., & Barcelo, D. (1996). Determination of phenolic compounds in water and waste water. Trends in Analytical Chemistry, 15, 362.
- Renberg, L., & Lindstrom, K. (1981). C18 reversed-phase trace enrichment of chlorinated phenols, guaiacols and catechols in water. Journal of Chromatography A, 214(3), 327. doi:10.1016/S0021-9673(00)80561-0
- Rompa, M., Kremer, E., & Zygmunt, B. (2005). Screening of Alkanocarboxylic and Phenolic Herbicides in Water Samples by Means of Derivatization-Based Gas Chromatography. Polish Journal of Environmental Studies, 14(1), 81–86.
- Shriadah, M. A., & Emara, H. I., 1991. The distribution of chromium, copper, cadmium and lead in areas of multi-polluting factors of Alexandria. In: Proceeding of Symposium of Marine Chemistry in the Arab Region, Suez, April, pp. 39–50.
- Swarts, M., Verhagen, F., Field, J., & Wijnberg, J. (1998). Trichlorinated phenols from Hypholoma elongatum. Phytochemistry, 49(1), 203. doi:10.1016/S0031-9422(97)01067-4
- Takasu, T., Iles, A., & Kiyoshi, H. (2002). Determination of alkylphenols and alkylphenol poly-ethoxylates by reversed phase high-performance liquid chromatography and solid phase extraction. Analytical and Bioanalytical Chemistry, 372(4), 554–564. doi:10.1007/s00216-001-1135-4
- Turnes, M. I., Rodrıguez, I., Mejuto, M. C., & Cela, R. (1994). Determination of chlorophenols in drinking water samples at the subnanogram per millilitre level by gas chromatography with atomic emission detection. Journal of Chromatography A, 683(1), 21–29. doi:10.1016/S0021-9673(94)89098-6
- WSRC. (1999). Ecological Constituents of Potential Concern Selection Process. Savannah River Site, Aiken, SC: Westinghouse Savannah River Company.
- Zakaria, H. Y., Radwan, A. A., & Said, M. A. (2007). Influence of salinity variations on zooplankton community in El-Mex Bay, Alexandria, Egypt. Egyptian Journal of Aquatic Research, 33(3), 52–67.