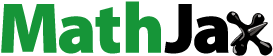
ABSTRACT
This study investigates the impact of different fertilizers under varying irrigation systems and crop water requirements in water-scarce Sinai, Egypt. Field experiments were conducted during the agricultural winter seasons of 2021 and 2022 to study the response of wheat growth, yield, and water productivity under drip and furrow irrigation. The results showed that under drip irrigation system, pH showed no clear trend, while total dissolved solids (TDS) decreased with depth and were reduced by bio-fertilizer and the combination treatment. On the other hand, under furrow irrigation system, maintained a slightly alkaline pH with minimal fertilizer influence. TDS levels significantly varied, and the effects of fertilizers differed. Calcium carbonate content and organic matter varied with fertilizer types and depths. Increasing the crop water requirement from 80% to 100% improved plant growth and yield, with the 80% treatment demonstrating higher water productivity. Furrow irrigation resulted in higher growth and yields, while drip irrigation exhibited greater water productivity. Nano-fertilizer treatments consistently outperformed bio-fertilizer in growth, yields, and water productivity. Specific combinations showed superior performance due to interactions between factors. Suitable irrigation methods and regimes enhance water use efficiency. Higher irrigation levels enhance wheat growth and yield, requiring careful water management to avoid stress. Drip irrigation demonstrates superior water productivity. Reducing the water input to 80% of crop water requirements improves efficiency without compromising yield. Nano-fertilizers promote growth and grain retention as an alternative to soluble fertilizers. Combining nano with bio-fertilizers further enhances wheat growth, yield, and water productivity. These findings provide valuable insights for optimizing wheat production in water-scarce regions, considering irrigation methods, crop water requirements, and nitrogen fertilization sources.
Introduction
The surging Egyptian population necessitates desert soil reclamation and cultivation, demanding innovative methods in arid regions. Supplementary irrigation, a common practice, alters soil chemical composition and pH, affecting mineral saturation indexes (Hamed, Galal, Emara, & Soliman, Citation2019; Jacques, Šimůnek, Mallants, & van Genuchten, Citation2006; Jalali, Citation2007; Yang, Wang, Hu, & Zeng, Citation2017).
To optimize crop growth in calcareous soils, essential nutrition strategies are crucial. Marschner and Römheld (Citation1994) emphasize pH’s impact on nutrient availability, affecting chemical reactions in nutrient management. The presence of CaCO3 directly influences nutrient chemistry, impacting nitrogen, phosphorus, iron, zinc, magnesium, calcium, potassium, and copper accessibility (Taalab et al., Citation2019).
Considering the increasing global population and the rising food demand, agriculture faces significant pressure to enhance crop productivity while minimizing resource usage. Nanotechnology and bio-fertilizer research have garnered considerable attention due to their potential to revolutionize crop cultivation and resource management (Al-Khafaji, Citation2018; Duhan et al., Citation2017).
Wheat (Triticum aestivum L.) is a globally vital cereal crop and staple food. Irrigation system choice significantly impacts water use efficiency (Hamed, Galal, Emara, & Soliman, Citation2019). Drip and furrow methods, known for efficient water delivery and reduced loss, are widely adopted. Evaluating wheat responses to diverse irrigation systems and water regimes assesses their efficiency under various conditions, with drip irrigation notably superior (Jha et al., Citation2019; Wang, Gong, Xu, Yu, & Zhao, Citation2013).
Climate change, extreme weather, and land fertility depletion challenge global food production (Hossain et al., Citation2022). Excessive synthetic fertilizer use worsens issues, causing economic burdens and environmental pollution. Nanotechnology provides a promising solution by enhancing fertilizer performance and minimizing environmental trade-offs. Nano-fertilizers, with nanoparticles delivering essential nutrients, improve nutrient uptake, use efficiency, and reduce environmental impacts (Bhardwaj et al., Citation2022; El-Saadony et al., Citation2021; Guru, Veronica, Thatikunta, & Reddy, Citation2015; Tarafdar, Xiang, Wang, Dong, & Biswas, Citation2012). Bio-fertilizers, with beneficial microorganisms promoting nutrient availability and plant growth, contribute to sustainable agriculture (Nazir, Ayub, & Tahir, Citation2020; Zulfiqar, Navarro, Ashraf, Akram, & Munn´e-Bosch, Citation2019).
Nano-materials in agriculture demand a deep understanding of their behavior in soil and interactions with other components. Studies highlight potential benefits such as controlled nutrient release and improved water retention with nano-fertilizers. Bio-fertilizers contribute to soil fertility and crop productivity (Baruah & Dutta, Citation2009; Cui, Sun, Liu, Jiang, & Gu, Citation2010; Gilbertson et al., Citation2020; Manjunatha, Biradar, & Aladakatti, Citation2016). Both types reduce excessive chemical fertilizer use, minimize nutrient losses, and boost crop yield (Mohammed et al., Citation2020; Prasad, Bhattacharyya, & Nguyen, Citation2017; Singh et al., Citation2017).
Moreover, the combined effects of nano- and bio-fertilizers on wheat crops can optimize nutrient management strategies and improve water use efficiency in agriculture.
This research aims to provide valuable insights into the utilization and impact of nano- and bio-fertilizers under different irrigation systems and varying levels of crop water requirements in a water-scarce region (Sinai, Egypt). Understanding the behavior of nano-materials in the soil system and their interactions with soil components will contribute to enhancing sustainable water and agricultural practices.
Materials and methods
Agricultural operations
Two field experiments were carried out in the El Tor Pilot area, situated in the South Sinai Governorate of Egypt at coordinates 28° 18’34” N and 33° 37’ 53.3” E, during the agricultural winter seasons of 2021 and 2022 (). Soil samples were collected at three different depths, namely 0–20 cm, 20–40 cm, and 40–60 cm, and underwent mechanical and chemical analyses. The results of the soil analyses are presented in .
Table 1. Physicochemical analysis of experimental soil.
Experimental design
The main treatment of the experiment is irrigation systems as follows:
• Furrow Irrigation
• Drip Irrigation
The sub-main treatment of the experiment is fertilizers as follows:
• Without fertilization, zero treatment
• Nano-technology fertilization (nitrogen concentration 3000 ppm) (Nano-fertilizer)
• Biogen Fertilization (Bio-fertilizer)
• Nano-technology nitrogen fertilization + Biogen fertilization
− Three replicates were used for each treatment.
Total coefficients for statistical design
= 2 irrigation systems × 4 fertilization treatments × 3 replicates = 24 complete experimental plots
The area of the experimental plot is = 10 × 10 = 100 m2
The total experimental area = 2400 m2
In this study, the impact of two irrigation methods, furrow and drip, was assessed on wheat cultivation with varying water regimes: 80% and 100% of crop water requirements. Four nitrogen fertilization treatments included nano-fertilizer (3000 ppm N concentration, 3.5 L ha−1, applied thrice before boot stage), bio-fertilizer (“Biogenic” at 2.5 kg ha−1, mixed with sand, applied after planting and 1 month later), a combined treatment of nano- and bio-fertilizers, and a control based on Ministry of Agriculture recommendations. Superphosphate fertilizer (15.5%) at 300 kg ha−1 was applied pre-planting. Ammonium nitrate fertilizer (33.5%) was applied in three doses totaling 120 units, with application stopped at grain formation onset. Potassium sulfate fertilizer (48%) at 50 kg ha−1 was added one-month post-planting. Experimental plots, 100 m2 each with a 3-m separation between irrigation treatments, used a 1-m spacing within each replicate. Two-meter distance between the main pieces prevented the overlap of experimental factors.
The chosen wheat cultivar, Giza 171, was sown in early November of both 2021 and 2022. A seed rate of 190 kg ha−1, sown at a depth of 4–5 cm with a 20 cm row spacing, optimized plant stands. Pre-sowing, superphosphate fertilizer (15.5% P2O5) at 110 kg P2O5 ha−1 was applied, and 4 weeks later, potassium sulfate fertilizer (48% K2O) at 60 kg K2O ha−1 was applied. All recommended cultural practices were followed.
Immediate irrigation took place after planting, following a pre-sowing irrigation event. The specific amount of irrigation water applied can be found in . At the final ripening stage, the plants were harvested on April 2nd in both 2021 and 2022 seasons. Following the completion of the harvesting stage, the number of plants per square meter, biological yield (t ha−1), grain yield (t ha−1), and straw yield (t ha−1) were determined. At harvest stage, plant samples were taken to evaluate growth and quality traits such as plant height (in cm), spike length (in cm), spike weight (in g), root length (in cm), seed index (in g), and harvest index (HI). The harvest index was calculated using the formula:
Table 2. Amount of applied water under each irrigation system.
In addition, the quantities of irrigation water were measured using a flow-meter for each treatment, and the results are presented in . Water productivity (WP) was calculated following the equation proposed by (Ali, Hoque, Hassan, & Khair, Citation2007) to determine the efficient utilization of water resources.
Statistical analysis
The data obtained from the experiments were subjected to statistical analysis using a split–split plot arrangement in a randomized complete block design (RCBD) with three replicates. The statistical software Genstat Twelfth Edition (Goedhart and Thissen, Citation2009) was employed for the analysis. To assess the significance of differences between treatment means, the least significant difference (LSD) test was conducted at a probability level of 0.05.
Results
Soil chemical properties under varied irrigation systems
displays results of fertilization techniques on soil properties post-harvest with drip irrigation. The pH values varied with fertilizers and depths. The control group (NPK) showed pH values of 8.28, 8.42, and 8.30 at 0–20 cm, 20-40 cm, and 40–60 cm, respectively. Bio-fertilizer slightly reduced pH to 7.79, 7.89, and 8.21. Nano-fertilizer exhibited pH compared to control and the combination of nano-fertilizers and bio-fertilizers decreased pH. Drip irrigation showed no clear pH trend.
Table 3. Effect of different fertilization techniques on soil chemical properties using drip irrigation system.
Total dissolved solids (TDS)
The control group without nano- or biofertilizer had TDS values of 8700 ppm, 7005 ppm, and 6862.5 ppm at 0–20 cm, 20–40 cm, and 40–60 cm. Bio-fertilizer reduced TDS to 7540, 7472, and 8235 ppm, while nano-fertilizer showed mixed TDS results, with higher values at 0–20 cm (9860 ppm) and similar or slightly lower values at deeper depths. The combination of nano- and bio-fertilizers resulted in decreased TDS values (7308, 7285.2, and 8052 ppm). Drip irrigation led to decreasing TDS values with depth. Bio-fertilizer and the combination generally had lower TDS compared to the control, while nano-fertilizer showed mixed effects.
CaCO3 content
CaCO3 content varied across depths and fertilizers. Without nano- or bio-fertilizer, CaCO3 percentages ranged from 46.1% to 50.0%. Bio-fertilizer reduced CaCO3 (44.00% to 49.1%), while nano-fertilizer showed slightly higher CaCO3 (46.1% to 50.2%), especially in the 20–40 cm range. The combination treatment had mixed results, with CaCO3 percentages ranging from 45.62% to 49.00%.
Organic content
Organic matter content percentages in the control treatment ranged from 0.62% to 0.68%. Bio-fertilizer maintained consistent organic matter content (0.55% to 0.69%), while nano-fertilizer showed slightly lower organic content (0.51% to 0.59%) in the 20–40 cm and 40–60 cm ranges. The combination treatment resulted in varying organic content levels.
Soil chemical properties under furrow irrigation
Results () display the impact of varied fertilization on post-harvest soil properties in furrow irrigation. pH, generally alkaline (8.28–8.42) without nano- or bio-fertilizer, slightly decreased with bio-fertilizers (7.84–7.90) and nano-fertilizers (7.76–7.86). Combining both showed a slightly higher pH (7.89–7.95). Changes were minimal, suggesting the furrow irrigation system and fertilizers minimally affected pH, remaining alkaline.
Table 4. Effect of different fertilization techniques on soil chemical properties using furrow irrigation system.
Total dissolved solids (TDS)
TDS levels varied significantly. Without fertilizers, TDS ranged from 5947.5 ppm to 7540 ppm. Bio-fertilizer maintained consistent levels (7098.4 ppm to 7777.5 ppm), while nano-fertilizer showed higher levels (7939 ppm to 9280 ppm). Combining both resulted in TDS ranging from 6380 ppm to 7320 ppm. Different fertilizers and depths influenced TDS significantly in the furrow irrigation system.
CaCO3 content
CaCO3 content varied across depths and fertilizers. Without nano- or bio-fertilizer, CaCO3 percentages ranged from 44.1% to 50.0%. Bio-fertilizer reduced CaCO3 (41.00% to 45.8%), while nano-fertilizer slightly increased it (45.1% to 49.23%). The combination treatment showed mixed results (44.62% to 48.00%). Varied fertilization influenced CaCO3 content, providing insight into furrow irrigation system effects.
Organic content
Organic content fluctuated across depths and fertilizers. The control treatment recorded organic content percentages ranged from 0.69% to 0.71%. Bio-fertilizer increased organic content (0.69% to 0.59%), while nano-fertilizer showed consistency (0.61% to 0.58%), and the combination treatment varied (0.59% to 0.70%). These findings offer insights into fertilization impact on soil properties in furrow irrigation, vital for optimizing agricultural practices and maximizing crop yield.
Crop water requirement (CWR)
The influence of different percentages of crop water requirement 80% and 100% on wheat plant characteristics and yields was investigated. Increasing the CWR had a significant positive effect on plant growth and yield in both the first and second seasons. Specifically, raising the CWR from 80% to 100% resulted in a considerable increase in spike length by 14.12% and 5.26%, spike weight by 13.79% and 17.50%, and seed index by 5.02% and 5.28% during the 1st and 2nd seasons, respectively ().
Table 5. Wheat plant growth and seed index as affected by crop water requirement (CWR) levels in 2021 and 2022 seasons.
Furthermore, a modest but noteworthy increase was observed in biological yield (2.38% and 2.71%), grain yield (11.45% and 3.75%), straw yield (5.06% and 1.36%), and harvest index (HI) (10.89% and 1.04%) ().
Table 6. Number of plants, yields, and harvest index (HI) as affected by crop water requirement (CWR) levels in 2021 and 2022 seasons.
Water productivity (WP)
Conversely, intriguingly, the treatment with 80% of CWR demonstrated superior water productivity (WP) compared to the 100% treatment. Specifically, the 80% CWR treatment exhibited a notable 11.84% and 11.92% enhancement in WP for biological yield, a significant 4.55% and 11.51% increase in WP for grain yield, and a substantial 9.37% and 13.63% augmentation in WP for straw yield were demonstrated during the first and second seasons, respectively ().
Table 7. Water productivity (WP) affected by crop water requirement (CWR) levels in 2021 and 2022 seasons.
Irrigation method
Significant differences were observed among irrigation systems in terms of growth and yield traits, as well as water productivity, in both seasons, except for spike length and spike weight in the first season. The furrow irrigation system resulted in higher wheat plant growth and yields, followed by the drip irrigation system ().
Table 8. Wheat plant growth and seed index as affected by irrigation methods in 2021 and 2022 seasons.
In terms of the harvest index, the drip irrigation system recorded values of 32.45% and 33.81% in the 1st and 2nd seasons, respectively, while the furrow irrigation system had values of 29.60% and 32.50% ().
Table 9. Yield and yield components of wheat as affected by irrigation methods in 2021 and 2022 seasons.
On the contrary, the drip irrigation system exhibited the highest values of water productivity (WP) for biological yield, with an average of 2.83 kg.m−3 for the first and second seasons. Additionally, it showed values of 0.94 kg.m−3 for WP for grain yield and 1.78 kg.m−3 for WP for straw yield. These values represented significant increases compared to the furrow irrigation system. Specifically, there was a 24.12% increase in WP for biological yield, a 27.03% increase in WP for grain yield, and a 29.93% increase in WP for straw yield ().
Table 10. Water productivity of biological, grain, and straw as affected by irrigation methods in 2021 and 2022 seasons.
Nitrogen fertilization source
The data presented in revealed significant differences among nitrogen fertilization sources in the growth, yields, and water productivity of wheat plants for each season. Both the nano + bio-fertilizer treatments and nano-fertilizer treatment consistently exhibited the highest values across the studied traits compared to the bio-fertilizer treatment.
Table 11. Plant height, root length and spike length, spike weight, and seed index as affected by fertilization type in 2021 and 2022 seasons.
Table 12. Number of plants/m2, biological yield, grain yield, straw yield, and harvest index of wheat as affected by fertilization type in 2021 and 2022 seasons.
Table 13. Water productivity (WP) of wheat crop as affected by fertilization type in 2021 and 2022 seasons.
The nano + bio-fertilizer treatment demonstrated remarkable enhancements in the measured parameters when compared to the control treatment. On average, it resulted in substantial increases of 30.75% in plant height, 70.60% in root length, 23.66% in spike length, 48.33% in spike weight, 51.47% in seed index, 16.02% in the number of plants at harvest, 76.31% in biological yield, 105.41% in grain yield, 58.87% in straw yield, 23.01% in harvest index (HI), 78.19% in water productivity (WP) for biological yield, 110.00% in WP for grain yield, and 60.00% in WP for straw yield compared to the control treatment.
Similarly, significant improvements were observed when comparing the nano + bio-fertilizer treatment with the bio-fertilizer treatment. The nano + bio-fertilizer treatment showed notable increases of 16.22% in plant height, 38.43% in root length, 10.85% in spike length, 20.27% in spike weight, 5.50% in seed index, 10.53% in the number of plants at harvest, 38.33% in biological yield, 59.00% in grain yield, 21.41% in straw yield, 15.88% in harvest index (HI), 37.79% in water productivity (WP) for biological yield, 61.54% in WP for grain yield, and 20.35% in WP for straw yield.
Interaction
The interplay of crop water percentage, irrigation method, and nitrogen source significantly impacted wheat growth, yields, and water productivity in both seasons. Notably, pairing 100% crop water with drip irrigation and nano-fertilizer led to superior outcomes. This combination resulted in maximum plant height (129.5 cm) () the highest number of harvest plants (273) (), superior seed index (67.47 g) (), significant straw yield (9.84 t ha−1) (), elevated harvest index (0.49) (), and improved water productivity (WP) for biological yield (4.80 kg m3), WP for grain yield (1.83 kg m3), and WP for straw yield (3.23 kg m3) ().
Figure 6. Water productivity of biological yield (t ha-1), grain and straw (kg m-3) as affected by irrigation method and fertilizer type.
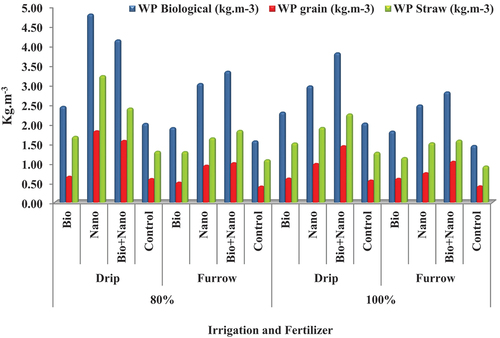
Furthermore, at 100% crop water, drip irrigation, and Nano + bio-fertilizer, the highest spike length (7.7 cm) () and spike weight (0.7 g) () were achieved. In the same conditions but with furrow irrigation, and nano + bio-fertilizer, the greatest root length (14 cm) () and grain yield (6.06 t ha−1) () were observed, along with the highest biological yield (17.43 t ha−1) ().
Discussion
Soil chemical properties
Soil pH and total dissolved solids (TDS)
The drip irrigation system efficiently delivered water to plant roots, reducing TDS values with increasing depth (Elbanna, Abou El-Magd, & Abo-Habaga, Citation2020; Mansingh, Banik, Bag, Sunaratiya, & Das, Citation2023). Bio-fertilizer further decreased TDS, enhancing microbial activity and minimizing salt accumulation. Combining nano- and bio-fertilizers with drip irrigation synergistically lowered TDS, indicating enhanced nutrient availability and cycling (Lei et al., Citation2022).
In contrast, furrow irrigation showed TDS variations, with the control treatment displaying a wide range. Bio-fertilizer maintained consistent TDS levels, positively influencing nutrient composition. Nano-fertilizer effects on TDS were more varied, potentially due to concentration near the soil surface. Combining nano- and bio-fertilizers with furrow irrigation yielded varied but generally lower TDS values, promoting nutrient retention and cycling (Liu et al., Citation2019).
The pH in drip irrigation showed no clear trend across depths, attributed to calcareous soil’s buffering capacity, maintaining pH above 8. Bio-fertilizer slightly reduced pH, and nano-fertilizer variations suggested some influence on soil pH. The precision of drip irrigation minimized the overall pH impact. However, consistent pH trends were lacking in other depths, indicating a relatively stable system. These results align with (Jacques, Šimůnek, Mallants, & van Genuchten, Citation2006) emphasizing irrigation and fertilization’s potential impact on soil composition and pH.
In furrow irrigation, soil pH remained consistently alkaline. Fertilizer types had no significant impact on pH compared to the control. However, Yang, Wang, Hu, and Zeng (Citation2017) found irrigation influenced soil moisture and pH (p < 0.05). Nano-bio-fertilizers showed a slightly lower pH at 20 cm depth, suggesting localized acidification. This trend was not uniform across other depths.
The pH decrease with bio-fertilizer is attributed to beneficial microorganisms enhancing soil microbial activity (Ammar et al., Citation2023). Some produce organic acids during metabolism, causing a slight pH decrease. pH variations with nano-fertilizer and nano+bio-fertilizer may result from specific compounds interacting with soil minerals and organic matter. These results were aligning with those of Liu et al. (Citation2022).
The furrow irrigation system’s slightly alkaline pH may result from factors like leaching acidic compounds, carbonate in irrigation water, or natural soil composition. These align with the findings of Hu et al. (Citation2019), where pH decreases in low rainfall, increasing water content, elevating ion concentration, and decreasing pH. Different fertilizer types minimally affect the furrow irrigation system pH. Minor pH variations could stem from localized effects, such as specific ion release or organic acids in the top 20 cm. However, inconsistent pH trends across depths suggest an overall stable pH, with variations influenced by water flow and fertilizer application.
Soil pH changes during the experiment result from factors such as initial pH, inputs of acidic or alkaline substances, and soil pH buffering capacity (pHBC) (Nelson & Su, Citation2010). Alkaline soils may be present due to base elements or compounds like carbonates. In the pH buffering process, CO2 is retained as HCO3− in the solution phase, increasing with higher pH levels (Oren & Steinberger, Citation2008; Sparling & West, Citation1990).
The use of bio-fertilizer and nano+bio-fertilizer lowered soil pH. Bio-fertilizers with organic materials and microorganisms can produce acidic by-products during microbial activity, contributing to pH decrease. The combination of nano- and bio-fertilizers may enhance microbial activity, leading to a slight pH decrease (Kumar, Diksha, Sindhu, Kumar, & Citation2021; Li et al., Citation2022). Nano-fertilizers minimally impact soil pH equilibrium. However, their application may initially increase pH due to the alkaline nature of the materials used (Rajonee, Zaman, & Huq, Citation2017).
Calcium carbonate (CaCO3) and organic matter content
In both drip and furrow irrigation, CaCO3 varied with depth and fertilizer types. In the drip system, bio-fertilizer lowered CaCO3 compared to control; nano-fertilizer slightly increased CaCO3, especially at 20–40 cm depth. Combined treatment had mixed results. In furrow irrigation, control had varying CaCO3 percentages, bio-fertilizer reduced CaCO3 compared to control, and nano-fertilizer slightly increased CaCO3, especially at 20–40 cm depth. Combined treatment had mixed results. Both irrigation systems and fertilizer types influenced soil CaCO3 content, but changes were relatively small and inconsistent, attributed to water movement and mineral dissolution influenced by irrigation methods.
The bio-fertilizer likely boosted microbial activity, decomposing organic matter (Ammar et al., Citation2023). This released acids reacting with CaCO3, reducing its content compared to the control. Conversely, nano-fertilizer impacted soil properties, affecting water retention and infiltration rates, influencing mineral movement, including CaCO3. This explains the slightly higher CaCO3 in the 20–40 cm depth range. These results were in agreement with Kumari et al. (Citation2014) whom they agreed that variations in colloid and phosphorus leaching were influenced by changes in pH and ionic strength due to CaCO3 presence.
Similarities between drip and furrow irrigation systems, in water application and soil-water dynamics, imply factors affecting CaCO3 under drip irrigation apply to furrow irrigation. Elbanna, Abou El-Magd, and Abo-Habaga (Citation2020) demonstrated that the accumulation of salts follows the direction of water flow. In general, the electrical conductivity (EC) and chloride concentration tended to increase as the applied water rates decreased. In drip irrigation, organic content variations across depths result from water and organic matter movement. Targeted water application near plant roots causes variations in organic matter distribution, and this finding aligns with Mursec’s (Citation2011) study.
Bio-fertilizers boost organic matter and nutrient cycling, maintaining consistent content compared to the control. In contrast, nano-fertilizers, especially at deeper depths, may slightly decrease organic content due to nano-particle effects on soil and microbial activity, impacting decomposition. The combination treatment shows varied organic content, influenced by interactions between bio-fertilizer and nano-fertilizer, affecting decomposition and nutrient cycling differently. After irrigation, NH4+ fully oxidized and nitrified as NO2− and NO3− approximately within 15 days, based on Liu, Šimůnek, Li, and He (Citation2013).
Irrigation method and crop water requirement
Improving water use efficiency is crucial for addressing water shortages and enhancing wheat growth and yield. Varying irrigation levels, particularly from 80% to 100% of crop water requirement, impact wheat traits. Water stress in lower irrigation treatments reduces leaf area index, photosynthesis rate, and dry matter accumulation, negatively affecting plant height, spike length, weight, and overall yield components. This aligns with previous findings of Kharrou et al. (Citation2011), Mahmoud, Hassanin, Borham, and Emara (Citation2018), and Hamed, Galal, Emara, and Soliman (Citation2019). Bhunia, Verma, Arif, Gochar, and Sharma (Citation2015) and Jha et al. (Citation2019) support these results. El-Hendawy, Hokam, and Schmidhalter (Citation2008) noted the significant impact of different irrigation levels on grain yield.
Irrigation methods, specifically furrow and drip systems, influence wheat growth and productivity. Furrow and drip systems show superior growth and yield traits without significant differences in most studied aspects. Root water uptake is crucial, with surface drip irrigation benefiting from a shallow welting soil zone and low irrigation rates. This aids root uptake, as reported by (Gaiser et al., Citation2012). Slow irrigation rates in low treatments increase soil water storage, contributing to enhanced root water uptake (Jha et al., Citation2019). (Kang et al., Citation2002) found varying harvest indices under different irrigation methods, emphasizing the positive impact of drip irrigation on crop development, reproduction, and yield (Blum, Citation2009).
Contrarily, using 100% of CWR reduced water productivity (WP) for biological, grain, and straw yields. Reducing water input to 80% of crop requirements enhanced water efficiency without compromising yield. Drip irrigation exhibited the highest water productivity, aligning with Wang, Gong, Xu, Yu, and Zhao (Citation2013). Improved water use efficiency can result from increased grain yield or reduced evapotranspiration. Drip irrigation stands out as the most efficient method, conserving water and minimizing deep percolation compared to surface flood irrigation (Jha et al., Citation2019).
Nitrogen fertilization source
Effective NPK nutrient availability in soil fosters vital plant processes, particularly nitrogen’s role in photosynthesis, hormone regulation, chlorophyll, and protein synthesis. This enhances cell division, elongation, and overall plant growth and height (AL-Samawi, Citation2012). This aligns with Mandal, Ali, Amin, Masum, and Mehraj (Citation2015), emphasizing nitrogen’s significance in augmenting plant growth and improving grain percentage on spikes, positively impacting spike grain count.
The use of slow-release nano-fertilizers offers an effective alternative to soluble mineral fertilizers, enhancing nutrient absorption without leaching. Wheat growth and yields, as well as water productivity (WP), improved significantly in nano+bio-fertilizer and nano-fertilizer treatments compared to bio-fertilizer and control. Nanoparticles, ranging from 1 to 100 nm, modify physiochemical properties due to a large surface area-to-volume ratio, enhancing soil fertility (Lopez-Serrano, Olivas, Landaluze, & C´ Amara, Citation2014; Tapan, Biswas, & Kundu, Citation2010). The interaction of nanoparticles with plants influences soil fertility, increasing wheat growth and yield in nano+biofertilizer treatments (Hasan & Saad, Citation2020; Jakhar et al., Citation2022). Nano-fertilizers, with efficient nutrient use, reduce losses, causing morphological and physiological changes (Mahmoud, El-Attar, & Mahmoud, Citation2017). Spruogis, Jakienė, Dautartė, and Zemeckis (Citation2018) reported an increased biological yield in barley with the use of nanoparticle fertilizers. in barley.
Biofertilizers containing non-symbiotic nitrogen-fixing bacteria enhance nitrogen availability, soil fertility, and nutrient efficiency (Mardalipour, Zahedi, & Sharghi, Citation2014; Hasan & Saad, Citation2020). Bio-fertilizers stabilize organic soil material, increasing plant lifespan and nutrient absorption, promoting growth and spike number (Ammar, Citation2018; Kaviani & Negahd, Citation2016). Bio-fertilizers stimulate growth hormones, increasing dry matter accumulation, photosynthesis, and biological yield (Mohamed, Thalooth, Elewa, & Ahmed, Citation2019). Foliar application of Biogen as a nano-fertilizer significantly improves wheat crop growth, seed yield, and nutrient absorption capacity (Mardalipour, Zahedi, & Sharghi, Citation2014). Increased yields in nano+biofertilizer and nano-fertilizer treatments contribute to higher water productivity for biological, grain, and straw compared to other treatments.
Conclusion
The drip system showed minimal pH changes, while furrow maintained a slightly alkaline pH. TDS varied across systems, with drip generally reducing TDS values, indicating improved leaching. Furrow, dependent on fertilizer type, exhibited significant TDS fluctuations, emphasizing careful selection for managing soil salinity.
Bio-fertilizers in the drip system positively influenced pH and TDS, decreasing pH due to organic acid production and reducing TDS through enhanced nutrient cycling. Furrow benefited from bio-fertilizers, maintaining consistent TDS levels and improving nutrient composition. Nano-fertilizers in furrow led to higher TDS, potentially due to increased nutrient uptake. Combining nano- and bio-fertilizers yielded positive effects, enhancing nutrient retention, leaching, and cycling for improved soil fertility and plant growth.
Water availability significantly influenced wheat growth under both systems, with no notable differences between them. The nano+bio-fertilizer treatment produced the highest growth, yield, and water productivity, followed by nano-fertilizer, bio-fertilizer, and control treatments.
In conclusion, this study underscores the need for careful irrigation system and fertilizer selection. Bio-fertilizers positively impacted soil conditions in both systems, while combining nano- and bio-fertilizers showed promise in enhancing soil fertility and crop productivity, offering insights for sustainable agriculture.
Disclosure statement
No potential conflict of interest was reported by the author(s).
References
- Ali, M. H., Hoque, M. R., Hassan, A. A., & Khair, A. (2007). Effects of deficit irrigation on yield, water productivity, and economic returns of wheat. Agricultural Water Management, 92(3), 151–161. doi:10.1016/j.agwat.2007.05.010
- Al-Khafaji, M. H., 2018. Effect of rhizobia and mycorrhiza inoculants and concentrations of vitamin B-complex on the growth and yield of (Vicia faba L.). [ Doctoral dissertation, Ph. D. Thesis]. Iraq: College of Agriculture, Al-Muthanna University.
- AL-Samawi, H. N. K., 2012. Field Evaluation of some plants water extracts on nitrification inhabitation, ammonia volatilization and barley growth (Hordeum vulgare L.). [ Ph.D. Thesis]. Baghdad University: College of Agriculture.
- Ammar, A. S. (2018). Nanotechnologies associated to floral resources in agri-food sector. Acta Agronomy, 67(1), 146–159. doi:10.15446/acag.v67n1.62011
- Ammar, E. E., Rady, H. A., Khattab, A. M., Amer, M. H., Mohamed, S. A., Elodamy, N. I., & AL-Farga, A. (2023). A comprehensive overview of eco-friendly bio-fertilizers extracted from living organisms. Environmental Science and Pollution Research, 30(53), 113119–113137. doi:10.1007/s11356-023-30260-x
- Baruah, S., & Dutta, J. (2009). Nanotechnology applications in pollution sensing and degradation in agriculture: A review. Environmental Chemistry Letters, 7(3), 191–204. doi:10.1007/s10311-009-0228-8
- Bhardwaj, A. K., Arya, G., Kumar, R. P., Hamed, L. M., Pirasteh-Anosheh, H. … Singh, G. (2022). Switching to nanonutrients for sustaining agroecosystems and environment: The challenges and benefits in moving up from ionic to particle feeding. Journal of Nanobiotechnology, 20(19), 1–28. doi:10.1186/s12951-021-01177-9
- Bhunia, S. R., Verma, I. M., Arif, M., Gochar, R., & Sharma, N. C. (2015). Effect of crop geometry, drip irrigation and bio-regulator on growth, yield and water use efficiency of wheat (Triticum aestivum L.). SVU-International Journal of Agricultural Sciences, 11(1), 45–49. doi:10.15740/HAS/IJAS/11.1/45-49
- Blum, A. (2009). Effective use of water (EUW) and not water-use efficiency (WUE) is the target of crop yield improvement under drought stress. Field Crops Research, 112(2–3), 119–123. doi:10.1016/j.fcr.2009.03.009
- Cui, H. X., Sun, C. J., Liu, Q., Jiang, J., & Gu, W., 2010, June. Applications of nanotechnology in agrochemical formulation, perspectives, challenges and strategies. In International conference on Nanoagri, Sao pedro, Brazil (pp. 28–33).
- Duhan, J. S., Kumar, R., Kumar, N., Kaur, P., Nehra, K., & Duhan, S. (2017). Nanotechnology: The new perspective in precision agriculture. Biotechnology Reports, 15, 11–23. doi:10.1016/j.btre.2017.03.002
- Elbanna, E., Abou El-Magd, A., & Abo-Habaga, M. (2020). Economic of drip irrigation system in new reclamination lands أقتصادیات الری بالتنقیط فی الأراضی حدیثة الأستصلاح. Journal of Soil Sciences and Agricultural Engineering, 11(8), 369–375. doi:10.21608/jssae.2020.114856
- El-Hendawy, S. E., Hokam, E. M., & Schmidhalter, U. (2008). Drip irrigation frequency: The effects and their interaction with nitrogen fertilization on sandy soil water distribution, maize yield and water use efficiency under Egyptian conditions. Journal of Agronomy and Crop Science, 194(3), 180–192. doi:10.1111/j.1439-037X.2008.00304.x
- El-Saadony, M. T., ALmoshadak, A. S., Shafi, M. E., Albaqami, N. M., Saad, A. M. … Helmy, A. M. (2021). Vital roles of sustainable nano-fertilizers in improving plant quality and quantity—an updated review. Saudi Journal of Biological Sciences, 28(12), 7349–7359. doi:10.1016/j.sjbs.2021.08.032
- Gaiser, T., Perkons, U., Küpper, P. M., Puschmann, D. U., Peth, S. … Köpke, U. (2012). Evidence of improved water uptake from sub soil by spring wheat following Lucerne in a temperate humid climate. Field Crops Research, 126, 56–62. doi:10.1016/j.fcr.2011.09.019
- Gilbertson, L. M., Pourzahedi, L., Laughton, S., Gao, X., Zimmerman, J. B. … Lowry, G. V. (2020). Guiding the design space for nanotechnology to advance sustainable crop production. Nature Nanotechnology, 15(9), 801–810. doi:10.1038/s41565-020-0706-5
- Goedhart, P. W., & Thissen, J. T. N. M. (2009). Biometris GenStat Procedure Library Manual 12th Edition NVol. 15). Wageningen. https://edepot.wur.nl/50492.
- Guru, T., Veronica, N., Thatikunta, R., & Reddy, S. N. (2015). Crop nutrition management with nano fertilizers. International Journal of Environmental Science and Technology, 1(1), 4–6.
- Hamed, L., Galal, Y., Emara, E., & Soliman, M. (2019). Optimum Applications of Nitrogen Fertilizer and Water Regime for Wheat (Triticum aestivum L.). Using 15N Tracer technique under Mediterranean Environment. Egyptian Journal of Soil Science, 41–52. doi:10.21608/ejss.2019.9863.1250
- Hasan, B. K., & Saad, T. M. (2020). Effect of nano biological and mineral fertilizers on NPK uptake in wheat (Triticum aestivum L.). Indian Journal of Ecology, 47(12), 126–130.
- Hossain, A., Maitra, S., Pramanick, B., Bhutia, K. L., Ahmad, Z. … Hassan, M. M. (2022). Chapter 22-wild relatives of plants as sources for the development of abiotic stress tolerance in plants. In T. Aftab & A. Roychoudhury (Eds.), Plant perspectives to global climate changes (pp. 471–518). Elsevier. doi:10.1016/B978-0-323-85665-2.00011-X
- Hu, A., Yu, Z., Liu, X., Gao, W., He, Y., & Li, J. (2019). The effects of irrigation and fertilization on the migration and transformation processes of main chemical components in the soil profile. Environmental Geochemistry and Health, 41(6), 2631–2648. doi:10.1007/s10653-019-00298-3
- Jacques, D., Šimůnek, J., Mallants, D., & van Genuchten, M. T. (2006). Operator-splitting errors in coupled reactive transport codes for transient variably saturated flow and contaminant transport in layered soil profiles. Journal of Contaminant Hydrology, 88(3–4), 197–218. doi:10.1016/j.jconhyd.2006.06.008
- Jakhar, A. M., Aziz, I., Kaleri, A. R., Hasnain, M., Haider, G., Ma, J., & Abideen, Z. (2022). Nano-fertilizers: A sustainable technology for improving crop nutrition and food security. NanoImpact, 27, 100411. doi:10.1016/j.impact.2022.100411
- Jalali, M. (2007). Assessment of the chemical components of famenin groundwater, western Iran. Environmental Geochemistry and Health, 29(5), 357–374. doi:10.1007/s10653-006-9080-y
- Jha, S. K., Tefo, S. R., Guangshuai, W., Yueping, L., Hao, L., Yang, G., & Aiwang, D. (2019). Response of growth, yield and water use efficiency of winter wheat to different irrigation methods and scheduling in North China Plain, agric. Agricultural Water Management, 217, 292–302. doi:10.1016/j.agwat.2019.03.011
- Kang, S., Zhang, L., Liang, Y., Hu, X., Cai, H., & Gu, B. (2002). Effects of limited irrigation on yield and water use efficiency of winter wheat in the Loess Plateau of China, Agric. Water Management, 55(3), 203–216. doi:10.1016/S0378-3774(01)00180-9
- Kaviani, B., & Negahd, N. (2016). Effects of biological nanofertilizer on the morphological, physiological and proliferation traits and quality of Buxus Hyrcana Pojark. Bangladesh Journal of Botany, 45(5), 1135–1142.
- Kharrou, M., Er-Raki, S., Chehbouni, A., Duchemin, B., Simonneaux, V. … Jarlan, L. (2011). Water use efficiency and yield of winter wheat under different irrigation regimes in a semi-arid region. Agricultural Sciences, 2(3), 273–282. doi:10.4236/as.2011.23036
- Kumar, S., Diksha, Sindhu, S. S., & Kumar, R. (2021). Biofertilizers: An ecofriendly technology for nutrient recycling and environmental sustainability. Current Research in Microbial Sciences, 3, 100094. doi:10.1016/j.crmicr.2021.100094
- Kumari, K. G. I. D., Moldrup, P., Paradelo, M., Elsgaard, L., Hauggaard-Nielsen, H., & de Jonge, L. W. (2014). Effects of biochar on air and water permeability and colloid and phosphorus leaching in soils from a natural calcium carbonate gradient. Journal of Environmental Quality, 43(2), 647–657. doi:10.2134/jeq2013.08.0334
- Lei, H., Jie, Y., Zang, M., Pan, H., Liu, X., Zhang, Z., & Jun, D. (2022). Effects of water-fertilizer-air-coupling drip irrigation on soil health status: Soil aeration, enzyme activities and microbial biomass. Agronomy, 12(11), 2674. doi:10.3390/agronomy12112674
- Li, H., Luo, N., Ji, C., Li, J., Zhang, L., Xiao, L., & Guo, Q. (2022). Liquid organic fertilizer amendment alters rhizosphere microbial community structure and co-occurrence patterns and improves sunflower yield under salinity-alkalinity stress. Microbial Ecology, 84(2), 423–438. doi:10.1007/s00248-021-01870-0
- Liu, Y., Cui, E., Neal, A. L., Zhang, X., Li, Z. … Hu, C. (2019). Reducing water use by alternate-furrow irrigation with livestock wastewater reduces antibiotic resistance gene abundance in the rhizosphere but not in the non-rhizosphere. Science of the Total Environment, 648 2019 Jan 1512–24.10.1016/j.scitotenv.2018.08.101
- Liu, Q., Pang, Z., Yang, Z., Nyumah, F., Hu, C., Lin, W., & Yuan, Z. (2022, Nov). Bio-fertilizer affects structural dynamics, function, and network patterns of the sugarcane rhizospheric microbiota. Microbial Ecology, 84(4), 1195–1211. Epub 2021 Nov 24. PMID: 34820729; PMCID: PMC9747866. 10.1007/s00248-021-01932-3
- Liu, X., Šimůnek, J., Li, L., & He, J. (2013). Identification of sulfate sources in groundwater using isotope analysis and modeling of flood irrigation with waters of different quality in the Jinghuiqu district of China. Environmental Earth Sciences, 69(5), 1589–1600. doi:10.1007/s12665-012-1993-4
- Lopez-Serrano, A., Olivas, R. M., Landaluze, J. S., & C´ Amara, C. (2014). Nanoparticles: A global vision. Characterization, separation, and quantification methods. Potential Environmental and Health Impact Analysis Methods, 6, 38–56. doi:10.1039/C3AY40517F
- Mahmoud, A. W. M., El-Attar, A. B., & Mahmoud, A. A. (2017). Economic evaluation of nano and organic fertilisers as an alternative source to chemical fertilisers on Carum carvi L. Plant yield and components. Agriculture (Pol’nohospodárstvo), 63(1), 33–49. doi:10.1515/agri-2017-0004
- Mahmoud, E. S. A., Hassanin, M. A., Borham, T. I., & Emara, E. I. (2018). Tolerance of some sugar beet varieties to water stress. Agricultural Water Management, 201, 144–151. doi:10.1016/j.agwat.2018.01.024
- Mandal, M. S. H., Ali, M. H., Amin, A. K. M. R., Masum, S. M., & Mehraj, H. (2015). Influence of source of nitrogen on growth and yield of wheat. International Journal of Agronomy and Agricultural Research, 6(1), 89–95.
- Manjunatha, S., Biradar, D., & Aladakatti, Y. R. (2016). Nanotechnology and its applications in agriculture: A review. International Journal of Farm Sciences, 29, 1–13.
- Mansingh, B., Banik, R., Bag, S., Sunaratiya, A., & Das, T. (2023). Drip irrigation system: An effective approach for increasing water use efficiency. In Green technologies for sustainable agriculture (pp. 247–262). Biotech Books. doi:10.5281/zenodo.7985739
- Mardalipour, M., Zahedi, H., & Sharghi, Y. (2014). Evaluation of nanobiofertilizer efficiency on agronomic traits of spring wheat at different sowing date. Biological Forum–An International Journal, 6(2), 349–356.
- Marschner, H., & Römheld, V. (1994). Strategies of plants for acquisition of iron. Plant and Soil, 165(2), 261–274. doi:10.1007/BF00008069
- Mohamed, M. F., Thalooth, A. T., Elewa, T. A., & Ahmed, A. G. (2019). Yield and nutrient status of wheat plants (Triticum aestivum) as affected by sludge, compost, and biofertilizers under newly reclaimed soil. Bulletin of the National Research Centre, 43(1), 31. doi:10.1186/s42269-019-0069-y
- Mohammed, S. B., Mohammad, I. F., Pangirayi, T. B., Vernon, G., Dzidzienyo, D. K., Umar, M. L., & Umar, S. (2020). Farmers’ knowledge, perception, and use of phosphorus fertilization for cowpea production in Northern Guinea Savannah of Nigeria. Heliyon, 6(10), e05207. doi:10.1016/j.heliyon.2020.e05207
- Mursec, M. 2011. Agricultural practices impact on soil quality and health: Case study of Slovenian irrigated or organic orchards. Earth sciences. English Université de Bourgogne. https://theses.hal.science/tel-00708232
- Nazir, R., Ayub, Y., & Tahir, L. (2020). Green-nanotechnology for precision and sustainable agriculture. In M. Ghorbanpour, P. Bhargava, A. Varma, & D. Choudhary. Eds., Biogenic nano-particles and their use in agro-ecosystems. Springer, Singapore. 10.1007/978-981-15-2985-6_18
- Nelson, P., & Su, N. (2010). Soil pH buffering capacity: A descriptive function and its application to some acidic tropical soils. Australian Journal of Soil Research, 48(3), 201–207. doi:10.1071/SR09150
- Oren, A., & Steinberger, Y. (2008). Coping with artifacts induced by CaCO3–CO2–H2O equilibrium in substrate utilization profiling of calcareous soils. Soil Biology & Biochemistry, 40(10), 2569–2577. doi:10.1016/j.soilbio.2008.06.020
- Prasad, R., Bhattacharyya, A., & Nguyen, Q. D. (2017). Nanotechnology in sustainable agriculture: Recent developments, challenges, and perspectives. Frontiers in Microbiology, 8, 1014. doi:10.3389/fmicb.2017.01014
- Rajonee, A., Zaman, S., & Huq, S. (2017). Preparation, characterization and evaluation of efficacy of phosphorus and potassium incorporated nano fertilizer. Advances in Nanoparticles, 6, 62–74. doi:10.4236/anp.2017.62006
- Singh, S., Vishwakarma, K., Singh, S., Sharma, S., Dubey, N. K., Singh, V. K., & Tripathi, D. K. (2017). Understanding the plant and nanoparticle interface at transcriptomic and proteomic level: A concentric overview. Plant Gene, 11, B, 265–272. doi:10.1016/j.plgene.2017.03.006
- Sparling, G. P., & West, A. W. (1990). A comparison of gas chromatography and differential respirometer methods to measure soil respiration and to estimate the soil microbial biomass. Pedobiologia, 34(2), 103–112. doi:10.1016/S0031-4056(24)00013-1
- Spruogis, V., Jakienė, E., Dautartė, A., & Zemeckis, R. (2018). The influence of bioorganic nanofertilizer on spring barley and oilseed rape productivity and economical effectiveness. Žemės Ūkio Mokslai, 25(1), 18–26. doi:10.6001/zemesukiomokslai.v25i1.3666
- Taalab, A. S., & Ageeb, G. W. (2019). Comparison between procaine and isocarboxazid metabolism in vitro by a liver microsomal amidase-esterase. Middle East Journal of Agriculture Research, 8; 1, 96–105. doi:10.1016/0006-2952(75)90029-5
- Tapan, A., Biswas, A., & Kundu, S. (2010). Nano-fertiliser-a new dimension in agriculture. Indian Journal of Fertilisers, 6, 22–24.
- Tarafdar, J., Xiang, Y., Wang, W., Dong, Q., & Biswas, P. (2012). Nanoparticle synthesis characterization and application to solve some chronic agricultural problems. Applied Biological Research, 14, 138–144.
- Wang, J., Gong, S., Xu, D., Yu, Y., & Zhao, Y. (2013). Impact of drip and level-basin irrigation on growth and yield of winter wheat in the North China Plain. Irrigation Science, 31(5), 1025–1037. doi:10.1007/s00271-012-0384-7
- Yang, Y., Wang, Z., Hu, Y., & Zeng, Z. (2017). Irrigation frequency alters the abundance and community structure of ammonia-oxidizing archaea and bacteria in a northern Chinese upland soil. European Journal of Soil Biology, 83, 34–42. doi:10.1016/j.ejsobi.2017.09.005
- Zulfiqar, F., Navarro, M., Ashraf, M., Akram, N. A., & Munn´e-Bosch, S. (2019). Nanofertilizer use for sustainable agriculture: Advantages and limitations. Plant Science, 289, 110270. doi:10.1016/j.plantsci.2019.110270