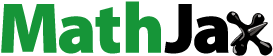
ABSTRACT
As a consequence of fresh water shortage, non-conventional water resources have become increasingly demandable in semi-arid and arid areas due to the increase in population and urbanization. In Egypt, the reuse of drainage water provides a demanding supplement to irrigation water. This research aims to present natural treatment process for drainage wastewater to be reused in irrigation to support water resources and water quality control. Two biological and chemical wastewater treatment techniques (Algae and Zeolite), under different conditions, were applied to allow the reuse of wastewater to be utilized in the agriculture of very economically important crop which is maize, and comparing the production and the chemical components of the crop with the one irrigated by fresh Nile water. Water, soil and plant analyses were performed. Zeolite was characterized by XRD, SEM and FTIR. Enzymes activities were assigned also (dehydrogenase, phosphatase and nitrogenase activities) and finally the statistical analysis was determined by CoStat software. The treated wastewater chemical and biological analyses showed much better results than El-Rahawy drain wastewater analyses. The plants growth parameters results showed the higher ones with plants subjected to the chemical and biological treatments. Protein and carbohydrate levels, which are the most important plant quality indicators, showed higher concentrations in the treated ones than that irrigated with Nile water. NPK concentration of both seeds and leaves also recorded better levels. The results of the enzymes activities in cases of T4, dehydrogenase, phosphatase, and nitrogenase enzyme activity were 127.66, 40.89, and 79.58, respectively, which increased when compared with control treated with Nile water which were (112.44, 28.4, and 41.39) for dehydrogenase, phosphatase and nitrogenase enzymes respectively.
Introduction
The shortage of freshwater to agriculture becomes a global issue, particularly for arid countries such as Egypt. As a result, many Egyptian farmers were forced to use wastewater for irrigation of the crops (Amer et al., Citation2017). Alhashimi et al. (Citation2024) reported that in Egypt due to a lack of freshwater, more than 240,500 feddans in the North Delta are watered by effluent of Al-Gharbiyyah Al-Raisi drain.
The per capita share of water in Egypt has decreased from 1893 m3y1- in 1959 to 940.9 m3y1- in 2010, which is less than the water shortage limit (1000 m3capita 1-y1-), and then to 868.4 m3y1- in 2020 (Kamal & Hashim, Citation2023). This very rapid growing demand for water has many reasons, the first and most significant one is the horrible population increase (from 63 million in 2000 to about 105 million in 2023, and it is expected to reach to 114.8 million in 2065 according to the United Nations projections). The uneven distribution of rainfall and periodic droughts have pushed the scientists, decision makers, and water agencies to find other sources of fresh water, other than the conventional ones, to cope with the increasingly water shortage problem; at least for irrigation sector. In this perspective, the reuse of treated wastewater could be a promising component for the way of solving the expanding food and irrigation water need issue (Kesari et al., Citation2021).
Wastewater reuse has become crucial due to its many economic and environmental benefits. It seems to be among the most useful, and economical means to raise the water budget in Egypt. First, it represents a stable and continuous source especially during high water demand times and considered as one of the main water resources that could be reused on a large scale for agriculture purposes particularly under the shortage in fresh water resources. It also allows the recovery of nutrients, resulting in a reduction of chemical fertilizer used. There are a lot of widely applied chemical and biological water recycling methods. For example: electro-chemical methods, coagulation, ion exchange, desalination, and chemical &biological adsorption methods. Worldwide, about 7.1 Bm3y−1 reused mainly for agricultural and industrial purposes (about 50 and 20% respectively) (Geriesh et al., Citation2023).
Maize Ranking third after rice and wheat in terms of cultivated area and nutritional value, it is one of the Egypt’s most important crops (Abo El-Ezz & Haffez, Citation2019). Maize grain is utilized to produce lactic acid and acetone, which are utilized in a number of industries, as well as corn oil and, corn starch. It also generates income for farm households and traders, in addition to being used as food, fermentation, textile, foundry, poultry and livestock feeds, and finally as a treatment for many medical problems (ex: blood pressure, cholesterol, and urinary problems) (Awwad et al., Citation2015). Because maize is a summer crop that is greatly affected by climate change, it is projected that the amount of irrigation water used in all Egyptian governorates will increase by between 10–19% by 2040 (Elbeltagi et al., Citation2020). Based on climate change scenarios, it is also predicted an approximate 16% increase in irrigation water requirements for summer crops by 2050 (El-Hamdi et al., Citation2020).
Because of its small production and high consumption, corn became a major import for Egypt. In Egypt, maize is planted in the summer and winter. Early planting for cultivation is favored at the second half of April as it allows growing of good maize plants, and lowers aphid infection. The maize crop must be harvested within 110 to 120 days (Khodeir & Abdelsalam, Citation2016).
Zeolite is a soil additive that improves soil properties by reducing water leaching, which helps agronomic crops by preserving soil water. Zeolites, both natural and synthetic, serve as carriers of the active ingredients of insecticides, fungicides, and herbicides, and contribute to slow-release mineral fertilizers, leading to increased nutrient efficiency over multiple vegetation periods. By gradually releasing essential nutrients like nitrogen, potassium, calcium, and magnesium, zeolites offer a sustainable solution for crop growth while minimizing fertilizer costs (Cataldo et al., Citation2021; Eroglu et al., Citation2017).
The term zeolite is a Greek word for “boiling stone,” referring to these materials’ ability to absorb water and release it when heated. Zeolite is a mineral in the “tectosilicates” class that occurs naturally as hydrated aluminosilicate minerals. It enhances the efficiency of water by increasing soil water holding capacity and plant availability (Lima et al., Citation2019). According to Al-Juthery et al. (Citation2021), the usage of mineral fertilizers combined with zeolite significantly increased nutrient uptake and efficiency by maize plants due to zeolite’s great ability to store nutrients as long as possible. Experimental results indicate that zeolite can be reused multiple times for heavy metal absorption before reaching its saturation point. Specifically, it can be reused for at least 20 cycles, which enhances its economic and practical viability (Zorpas et al., Citation2021).
For the biological side, many studies investigate the role of algae in wastewater treatment, emphasizing their capacity to purify water by absorbing and utilizing pollutants such as nitrogen, phosphorus, heavy metals, pesticides, toxins, and pathogens from surrounding water because of their bioaccumulation abilities (Shahi Khalaf Ansar et al., Citation2023).
Algae growing in nutrient-rich wastewater as a cultivation medium provides a dual benefit, reducing the need for traditional fertilizers by recycling the nutrients into algae biomass as a fertilizer and offering a sustainable biological wastewater treatment method by reducing the inorganic and organic load of wastewater (Wang & Zhang, Citation2022).
Algae able to fix CO2 by 40 times more than higher plants, which in turn became the most oxygen generator for the growth of aerobic bacteria Algal wastewater treatment led to the increase of O2 content in water bodies contributing to an eco-friendlier wastewater treatment process (Saba et al., Citation2017). Valchev and Ribarova (Citation2022) reported that Algal-based wastewater technology could be applied as a post-treatment in one step to remove nutrients. When it comes to eliminating nutrients from wastewater, a community of algae would perform better than a monoculture.
The study also emphasizes the importance of soil enzyme activities as markers of soil health. Enzyme activities respond promptly to soil management practices, fertilization, and heavy metal concentrations, making them valuable tools for determining the effects of different factors on soil quality. The water’s microbial community is essential for controlling the material cycle of the ecosystem. Soil microorganisms contributing to organic matter decomposition, nutrient cycling, fertilization of the soil, and development of soil structure, because the soil microorganisms synthesize soil organic carbon which increases soil’s fertility and water-retaining ability (Johns, Citation2017).
Soil enzyme activity serves as indicators of the physicochemical and microbiological quality of the soil (Aon & Colaneri, Citation2001; Mkhinini et al., Citation2020). Soil enzymes are essential parameters in carbon sequestration and soil nutrient dynamics. Soil dehydrogenases represent oxidoreductase enzymes that do not accumulate extra cellularly in soil but occur inside microbial cells linked to their oxido-reduction processes and indicate overall soil microbial activity, as they do share in biological oxidation of soil organic matter (OM) upon which their activities are found proportional to microbial biomass in soil (Lemanowicz et al., Citation2023; Wolińska & Stępniewska, Citation2012).
This work was conducted to investigate two biological and chemical wastewater treatment techniques (Algae and Zeolite) in order to assess the impact of reusing treated drainage wastewater, of El-Rahawy drain, to be used in the agriculture of specific type of the very economically important crop “corn” and comparing it with the one irrigated by fresh Nile water. Zeolite was added to the soil as a chemical treatment, while Chlorella vulgaris algae were used in the biological one (in the irrigation water). This research will also evaluate the remediation process on the crops’ quality and yield.
Materials and methods
Site description and experiment designation
The study of this research was carried out under field conditions in the research facilities of the Central Laboratory for Environmental Quality Monitoring (CLEQM), with the National Water Research Center, Qalubiya (30° 11´ 53.89” N; 31° 7´ 21.57” E). It was conducted during the summer season of 2022 to investigate the effect of row wastewater reuse and the treated wastewater, on some soil parameters and its effect on production of maize crop (vegetative growth, chemical components of dry seeds, and leaves yield) as well as soil enzyme activity.
The land was divided into four parts according to the type of applied treatment: Irrigated by fresh Nile water as control (T1), Irrigated by row wastewater (T2), Subjected to biological treatment: irrigated with wastewater treated by algae; Chlorella vulgaris (T3) and Exposed to biological and chemical treatment: wastewater treated by algae (Chlorella vulgaris) and zeolite was mixed to the soil (T4). Each treatment was divided into three replicate rows which resulting for 12 rows and a buffer zone separate between different treatments. A schematic diagram explains the experimental area is described by . The area of the experimental region was about 40 m2 of 10 m length and 4 m width and divided into four regions of about 1.5–2 m width and buffer zone of about 0.75 m separate between the four divisions.
Water sources and treatment tanks
Different water sources were used during the study including fresh Nile water and wastewater. Row wastewater was taken from El-Rahawy drain which located in Giza, Egypt (30°11′12.3′′N latitude and 31°02′53.3′′E longitude). The drain discharges combined effluent from two major sewage plants (Zenein and Abo-Rawwash), and an agricultural sewer system. El-Rahawy drain discharged between 1.9 and 2.8 Mm3day−1 into the Rosetta branch.
Natural Chlorella vulgaris algae were delivered from the National Research Center, Giza, Egypt. It is used, in this research, to study the concept of bio-treating wastewater to be reused in agriculture purposes. Chlorella vulgaris algae belong to Chlorophyta, that grow well in most Egyptian habitat. The biological treatment was done by putting El-Rahawy wastewater in glass tanks (with 50, 30, and 50 cm length, width, and height respectively). The selected algae Chlorella vulgaris was grown under both nitrogen and phosphorus enriched drain water. Thus, nitrogen was added as urea of 0.5 gL−1 in presence of Phosphoric acid 7 mgL−1 and, 10 gm of fresh Chlorella vulgaris Algae for each 50 L of wastewater. The tanks are subjected to aeration and lightening systems to allow the algae to grow normally. A small unit for growing algae has been constructed as shown in .
Cultivation, irrigation and harvesting process
Grains were planted on April 2022 at a rate of three seeds every 20 cm in rows that are 75 cm apart from each other. Three replicates of a split-plot were used in the investigation. Maize seeds TWC (Three-Way Cross) were delivered from Field Crops Research Institute, Agricultural Research Center, Ministry of Agriculture, Giza, Egypt.
In T1, commercial fertilizers were added to the plots to overcome nutrient deficiencies in relation to crop requirements and water quality as follows: fresh water plots (control) were fed 100% of the recommended nitrogen, phosphorous, and potassium (NPK) dose, in accordance with the Egyptian Ministry of Agriculture’s recommendations. Plants were fertilized without organic fertilizer. During land preparation, calcium super phosphate (15.5% P2O5) was supplied by a rate of 150 kg fed.−1 and, Urea (46% N) was applied by a rate of 200 kg fed.−1 in two equal dosages, before the initial and subsequent irrigations. For the other treatments (T2, T3, T4) the fertilizers reduced by 50% depending on algae and nutrient of wastewater as natural fertilizer (Zhang et al., Citation2024)
Commercial zeolite was obtained from Technolab Elbahaa group and, added for T4 by about 1500 Kg fed−1 for topsoil (Szatanik-Kloc et al., Citation2021). The plants were thinned after complete germination in order to attain the recommended plant density. Harvest dates were done on 4th August.
Water analysis
Sampling and preservation methods were performed according to Standard Method for Examination of Water and Wastewater APHA (Citation2017). Most of physicochemical analysis was determined for water samples as pH, electrical conductivity (EC), total dissolved solids (TDS), cations (Ca++, Mg++, Na+, K+), anions (Cl−, NO2−, NO3−, PO4—, SO4–), ammonia (NH3) and some heavy metals as (Cu++, Fe ions, Mn++, and Zn++). Also COD, BOD, Total Coliform, and Fecal Coliform were determined.
Plant sampling and analysis
Three groups (cobs, leaves, and stalks) were collected from each row of the 12 rows mentioned before, resulting in 36 samples. The samples collected carefully in bags of polyethylene, and taken to the lab for analyses. Each sample was weighed and the stalk length was measured, the samples washed and rinsed by distilled water. Thereafter, the leaves, and seeds were separated and oven dried at 70°C till constant weight, and grinded, and then maize grains and leaves samples were digested with sulfuric acid and hydrogen peroxide according to Kalra et al. (Citation1989), to convert them to the liquid state to facilitate the chemical analyses (potassium, and heavy metal contents). Also, determination of nitrogen by Kjeldahl method (Sosulski & Imafidon, Citation1990), and phosphorus by the vanadomolybdate procedure (Jackson, Citation1958) were performed. Carbohydrate concentrations were measured by hydrolyzing the polysaccharides into sample sugers by acid hydrolisis and estimating the resultant monosaccharides (Dubios et al., Citation1951). Chlorophyll is determined by extraction in 80% acetone, and the amount of chlorophyll is calculated according to Arnon (Citation1949). The concentrations of total chlorophyll were calculated using the following equation:
Where, A: absorbance at specific wavelengths,
V: final volume of chlorophyll extract, and
W: fresh weigh of tissue extracted
Soil analyses
The soil samples were air drying, crushing, and sieving through a 2.0 mm sieve before being examined for chemical and physical characteristics. Soil water extraction method (1 soil: 5 water) was utilized to determine of pH, EC, total dissolved solids (TDS) and chemical characteristics according to standard methods outlined by (Klute, Citation1986; Page, Citation1982), and heavy metals contents were determined by EDTA extraction.
Characterization of Zeolite
Natural zeolite used in this research is a commercial one and it was characterized by: X-ray diffraction (XRD) with PAN alytical – Empyrean using Cu Kα radiation (λ = 0.15406 nm, 40 mA, 45 kV, step size (°2Th.) 0.0260). While, scanning electron microscopy (SEM) was performed using Tescan SEM (TESCAN VEGA 3, Czech Republic). Samples were mounted on aluminum microscopy stubs using carbon tape, then coated with gold (Au) for 90 s using Quorum Techniques Ltd, sputter coater (Q150t, England), and Fourier transform infrared spectroscopy (FTIR) techniques have been analyzed with the ATR FTIR spectra (SHIMADZU, IRAffinity-1S, Surgut, Russia) over the wavenumber 4000–400 cm1-.
Hydrogen ion concentration (pH) and electrical conductivity (EC) of water samples were determined by WTW Multi 9620 IDS, while Biological oxygen demand (BOD) was measured by a respirometric method (5-day test) with a respirometer system (OxiTop measuring system “WTW” model 890) and Chemical oxygen demand (COD) was measured by a spectrophotometer (HACH 3900). Total anions as Chloride (Cl−), Nitrate (NO3–), Nitrite (NO2–), Phosphate (PO43–), and Sulphate (SO42-) were measured by ion chromatography model ICS-5000. Na+ and K+ ions were estimated using Flame photometer 410 Sherwood. Heavy metals were measured by (ICP-OES) with Ultra Sonic Nebulizer (USN) with instrumental detection limit 10%, Perkin Elmer Optima 5300, USA. The total soluble nitrogen (N) by Kjeldahl (Model: Gerhardt Vapodest 20s), phosphorus (P) using a spectrophotometer (UNICO Vis Model 1200, USA), and carbohydrate were determined T80 UV/Vis Spectrophotometer for plant samples.
Enzyme activities
Soil biological activities were determined for enzymes activities in soil rhizosphere. Some biological activities were determined in collected soil samples from maize rhizospheres at the end periods of planting. Dehydrogenase activity (EC 1.1.1.) was assayed using tri-phenyl tetra-zolium chloride (TTC) method according to Solaiman (Citation2007). One unit of dehydrogenase was defined as the amount of enzyme required to hydrolyze of TTC to form 1.0 µmol of tri-phenyl formazan (TPF) per hour (µmol g1-h1-). Phosphatase activity (E.C 3.1.3) was measured using para nitro phenyl phosphate (PNPP) according to Tabatabai and Bremner (Citation1969). Phosphatase activity of one unit was defined as the amount of enzyme needed to release 1 µg of p-nitro phenol hydrolyze per hour. Nitrogenase activity (E.C.1.18.6.1) was measured by acetylene reduction assay as described by Senthilkumar et al. (Citation2021).
Statistical analysis
The results that are shown are a mean of three replications of each experiment and analytical results. In order to determine the significance between treatments, the collected findings were submitted to a one-way (ANOVA) analysis of variance analysis (the type of analysis relied on the factors influenced the experiment) using CoStat software (Stern, Citation1991).
Result and discussion
The soil type of this experimental field was clay loam; zeolite was added to the soil to improve soil, and plant characterization under irrigation by wastewater
Characterization of Zeolite
Zeolites, depicted in , are porous crystalline framework substances having pores. Common zeolites have a structure based on silicate, in which part of the Si is substituted with Al (or other metals). This causes the framework and cations (usually Na or other alkaline earth metals) inside the pore structure to become negatively charged. This additionally contributes to a further significant feature, ion exchange, in which metal ions in the pore structure can be exchanged by other cations (Chester & Derouane, Citation2009).
XRD, FTIR and SEM were performed to characterize the structure of the present Zeolite. displays the zeolite’s XRD diffraction pattern. The sample’s XRD peaks were appeared to be with good agreement with the clinoptilolite’s diffraction patterns, while there were also some additional peaks that were identified as heulandite. Besides the zeolitic phase, silica (SiO2) was also detected. In the SEM image of zeolite typical crystals of clinoptilolite – heulandite family of zeolite were appeared.
Some of the common transmittance peaks of the IR chart are a mixture of clinoptiliolite and holandite zeolites are ():
3600–3200 cm−1: This region corresponds to the O-H stretching vibrations of water molecules and hydroxyl groups in the zeolites. The intensity and shape of the peaks depend on the amount and type of water present in the zeolites, as well as the hydrogen bonding network.
The chart shows that the transmission percentage in the region of 3600–3200 cm−1 is low, which means that there is a high absorption of infrared radiation by the O-H groups in the zeolites. This indicates that the zeolites have a high degree of hydration and contain many water molecules in their pores and channels with different types (free water, bound water, and zeolitic water). The free water is loosely attached to the zeolite surface and can be easily removed by heating.
2400–2000 cm−1: This region corresponds to the C-H stretching vibrations of organic molecules that may be adsorbed on the zeolites. The presence and nature of these molecules can affect the catalytic activity and selectivity of the zeolites.
1800–1400 cm−1: This region corresponds to the C=O stretching vibrations of carbonates, carboxylates, and carboxylic acids that may be present in the zeolites. These groups can act as acid-base sites and influence the acidity and basicity of the zeolites.
1200–900 cm−1: This region corresponds to the Si-O-Si and Al-O-Si stretching vibrations of the zeolite framework. The position and intensity of the peaks depend on the composition, structure, and symmetry of the zeolites. The peaks can also be affected by the presence of extra-framework cations, such as sodium, potassium, calcium, or magnesium, that balance the negative charge of the framework.
900–400 cm−1: This region corresponds to the Si-O-Si and Al-O-Si bending vibrations of the zeolite framework. The peaks can provide information about the ring size, pore size, and channel system of the zeolites. The peaks can also be influenced by the presence of extra-framework cations and water molecules.
Treatment of wastewater
The results of the physicochemical characteristics water samples that were collected are illustrated in . It was clear that the water of El-Rahawy drain contains more organic contaminants than the Nile River water, as indicated by high values of BOD, COD, and TOC and low values of DO for the former. In addition, El-Rahawy drain water was also contaminated with some heavy metals like Cu, Fe, Mn, and Zn. The current study’s findings showed that the wastewater contains BOD, and COD concentrations violating the limits of Egyptian standards. Furthermore, the results investigated the efficacy of algae to reduce these pollutants from wastewater.
Table 1. Water quality of Nile river and El-Rahawy wastewater drain, used for growing maize crop.
Growth parameters
It is clear from results that the yield parameters (plant height, stem diameter, ear length, total chlorophyll, and weight of 100 seeds) of the maize that was irrigated with treated waste water by algae and mixing zeolite with soil (T4) exhibited higher yield parameters compared to fresh Nile water (T1) but lower than that irrigated by untreated wastewater (T2).
Table 2. Growth parameters of Maize under different treatments at the end of experiment.
The observed differences in growth and yield parameters between treatments (T3) and (T4) were found to be significantly higher than those of treatment (T1), despite the utilization of only half amount of fertilizer attributed to algae’s nutrient recycling into algae biomass as a fertilizer and zeolite’s ability to retain nutrients in the topsoil which are slowly and gradually leached from the soil. Using of zeolite, and algae allow to apply fertilizer at lower dosages, which lowers crop production costs. Also, it is observed that, irrigation with (T2) showed increase trend comparing with fresh Nile water for the evaluated growth parameters, because wastewater contains more macronutrients and organic matters (OM). Concerning chlorophyll content, maize plants of T4 recorded the highest values of chlorophyll content regarding to control. In general, the treatment with algae improved the Corn growth parameters, but treatment by algae with addition of zeolite showed more increase in the improving growth parameters.
Data of showed the influence of different treatments and their interactions on grain, and leaves quality traits i.e. N, P, K, carbohydrates and protein (%). Additive of zeolite and algae treatment caused marked increase in plant quality parameters, and recorded the highest values. According to Ahmed et al. (Citation2010) and Baddour and El-Kafrawy (Citation2020), adding zeolite to the soil increased the amount of chlorophyll and increased corn plant production. It also shown how using mineral fertilizers combined with zeolite significantly boosted the amount of nutrients absorbed and the efficiency with which maize plants used them because of zeolite’s exceptional ability to retain nutrients for extended periods of time.
Table 3. Chemical analysis of maize seeds and leaves under different treatments at the end of experiment.
The findings also agreed with those of Hh Khalifa et al. (Citation2019) and Kulikova et al. (Citation2020), who found that zeolite application can improve field capacity, available water values, CEC, and available macronutrient availability.
On the other hand, the NPK concentrations had also higher values for the irrigated by wastewater (T2) than that obtained under irrigation by fresh Nile water (T1) due to the nutrient in drainage water. Based on the mean values (), it can be seen that, except for the control, irrigated by Wastewater and the different treatments with half dose fertilizer application, led to improvement of NPK concentrations of Maize plant. Crude protein content and carbohydrate which are the most important factors in maize quality have been significantly affected by zeolite application in corn cultivars ().
Heavy metals contents for all treatments
Heavy metals concentrations in maize leaves and seeds have been measured for the four treatments. illustrate the comparison between all treatments. Both figures clearly show that the accumulation of Cu, Fe, Mn, and Zn exhibited different trends in leaves and seeds. The highest concentrations of these heavy metals were found in leaves rather than seeds. Specifically, maize leaves had mean concentrations of 14.2, 167, 43.83, and 41.96 mg.kg1- for Cu, Fe, Mn, and Zn respectively, while seeds had 9.55, 132.13, 18.43, and 38.23 mg.kg1-. The results also indicate that some heavy metals accumulate in the soil, highlighting the role of zeolite in reducing heavy metal concentration in plants. The highest levels of heavy metals were observed in T2 (irrigated with raw wastewater), whereas the lowest levels were in T4 (soil mixed with zeolite). The application of zeolite significantly decreased heavy metal accumulation in plants due to its high exchange capacity, alkalinity, and large specific surface area.
Chemical and physical analysis of soil
In order to assess the effects of the treatments under study, surface soil samples (0–30 cm depth) were randomly taken from each plot once the maize plants reached the harvesting stage (after 110 days after sowing) at the end of the experiment. The soil type which used of this experiment was clay loam (coarse sand % 1.206, clay % 31.714, silt % 29.75, fine sand % 37.329).
Some chemical properties were shown in
Table 4. Main properties and concentrations heavy metals (mg kg−1, d.W.) in soils studied.
The enzyme activities in soil
The residual enzyme activities in soil at harvest in response to soil and different treatments of wastewater irrigation throughout the growth stages which used are shown in . These enzymes activities were representing part of soil biological characters after harvest, which gave considerable expectations for future impacts of using treated wastewater in soil ready conditions for the next plantation season.
Results in showed that the activity of soil enzymes increased in response to wastewater irrigation after the growth stages. In case of T2, the activity of dehydrogenase, phosphatase and nitrogenase enzymes were 148.87, 47.8, and 88.34, respectively, under the untreated wastewater irrigation increased when compared with control (T1) which were 112.44, 28.4, and 41.39 for dehydrogenase, phosphatase and nitrogenase enzymes respectively. Higher activities of all enzymes in soil irrigated with untreated wastewater could be due to the presence of carbon and other nutrients in polluted soil (Narasimha et al., Citation2011).
Likewise, in T3, the activity of dehydrogenase, phosphatase and nitrogenase enzymes were 116.5, 36.69, and 67.6, respectively which increased when compared with control. In contrast, the treatment of wastewater with Chlorella vulgaris decreased than highest activities of the three enzymes generated by wastewater toxic stress.
In cases of T4, the activity of dehydrogenase, phosphatase and nitrogenase enzymes were 127.66, 40.89, and 79.58, respectively, which also increased when compared with control. The treatment of wastewater with Chlorella vulgaris and adding zeolite to the soil increase the activities of enzymes compared with T3. The increased enzyme activity occurs due to addition of organic material and nutrients to the amended soils with zeolite through wastewater which serves as a source of energy for microbes and enzymes (Thimmegowda & Muthuraju, Citation2019).
Higher enzyme activities were noticed in the soil irrigated with treated wastewaters. The dehydrogenase activity ranged from (112.44 to 148.87 μg TPF formed g−1hr−1). The highest dehydrogenase activity for treated wastewater was observed in T4 compared with T3. The activity of phosphatase in the soil ranged from 28.4 to 47.8 μg PNP g−1hr−1 under different treatments. The nitrogenase activity ranged from 41.39 to 88.34 (N moles C2H4g−1hr−1).
Soil enzymes activities have been utilized as sensitive indicators for changes in soil quality associated with management and land uses (Martínez-Rodríguez et al., Citation2014).
Dehydrogenase activity was inhibited by the toxic effect of total phenol and hydroxyl methyl furfural (HMF) added with a byproduct amendment, also Phenolic compounds being released during both the plant vegetation and decomposition of soil additive matter contribute to a reduction in the biological activity of the soil (López-Piñeiro et al., Citation2013; Majchrzak et al., Citation2014).
Additional studies demonstrated that soil application of organic materials like food waste compost enhanced soil enzyme activity in lettuce cultivation (Lee et al., Citation2004; Shivakumara et al., Citation2019).
Conclusion
Irrigation of maize plants using untreated wastewater significantly increased the contents of yield parameters, compared with control (irrigated with Nile water), also copper, iron, manganese, and zinc exhibit the highest rate of plant uptake in seeds (edible part). However, applying Chlorella vulgaris treatment reduced the negative impacts of wastewater irrigation. Using Chlorella vulgaris algae in wastewater treatment plants effectively removes many contaminants, enhancing treatment efficiency and reducing biochemical oxygen demand. Zeolite can capture and store essential nutrients, gradually releasing them over time. This long-term storage reduces nutrient losses due to leaching and ensures a steady supply of NPK to plants throughout their growth cycles, and reducing the accumulation of copper, iron, manganese, and zinc in the edible part of maize plants and seeds. Higher enzyme activities, e.g. dehydrogenase, phosphates and nitrogenase, were noticed in the soil irrigated with treated wastewaters. The increased enzyme activity occurs due to addition of organic material and nutrients to the amended soils with zeolite through wastewater which serves as a source of energy for microbes and enzymes.
Therefore, the applications of Zeolite and Chlorella vulgaris in wastewater treatment supports environmental sustainability by decreasing reliance on chemicals and producing valuable biomass. Future studies for the economic and financial analysis is quite essential and needed to know the economic feasibility and practical application.
Disclosure statement
No potential conflict of interest was reported by the author(s).
References
- Abo El-Ezz, S. F., & Haffez, S. H. (2019). Effect of nitrogen fertilization, proline, plant spacing and irrigation intervals on growth of maize plant. Journal of Soil Sciences and Agricultural Engineering, 10(8), 447–456.
- Ahmed, O., Sumalatha, G., & Muhamad, A. N. (2010). Use of zeolite in maize (Zea mays) cultivation on nitrogen, potassium and phosphorus uptake and use efficiency. International Journal of the Physical Sciences, 5(15), 2393–2401.
- Alhashimi, A., Abdelkareem, A., Amin, M. A., Nowwar, A. I., Fouda, A., Ismail, M. A., Mustafa, A. E., Alharbi, M., Elkelish, A., & Sayed, A. M. (2024). Eco-friendly approach to decrease the harmful effects of untreated wastewater on growth, yield, biochemical constituents, and heavy metal contents of carrot (Daucus carota L. Environmental Science and Pollution Research, 31(9), 14043–14058.
- Al-Juthery, H. W., Lahmod, N. R., & Al-Taee, R. A. (2021). Intelligent, nano-fertilizers: A new technology for improvement nutrient use efficiency (article review). IOP Conference Series: Earth and Environmental Science, Ancona, Italy, IOP Publishing.
- Amer, M. H., Abd El Hafez, S. A., & Abd El Ghany, M. B. (2017). Water Saving in irrigated agriculture in Egypt. LAP LAMBERT Academic Publishing.
- American Public Health Association (APHA). (2017). Water and wastewater examination manual. Routledge.
- Aon, M., & Colaneri, A. (2001). II. Temporal and spatial evolution of enzymatic activities and physico-chemical properties in an agricultural soil. Applied Soil Ecology, 18(3), 255–270.
- Arnon, D. I. (1949). Copper enzymes in isolated chloroplasts. Polyphenoloxidase in beta vulgaris. Plant Physiology, 24(1), 1.
- Awwad, M., El-Hedek, K., Bayoumi, M., & Eid, T. (2015). Effect of potassium humate appliction and irrigation water levels on maize yield, crop water productivity and some soil properties. Journal of Soil Sciences and Agricultural Engineering, 6(4), 461–482.
- Baddour, A., & El-Kafrawy, M. (2020). Effect of zeolite soil addition under different irrigation intervals on maize yield (Zea mays L.) and some soil properties. Journal of Soil Sciences and Agricultural Engineering, 11(12), 793–799.
- Cataldo, E., Salvi, L., Paoli, F., Fucile, M., Masciandaro, G., Manzi, D., Masini, C. M., & Mattii, G. B. (2021). Application of zeolites in agriculture and other potential uses: A review. Agronomy, 11(8), 1547.
- Chester, A. W., & Derouane, E. G. (2009). Zeolite characterization and catalysis. Springer.
- Dubios, M., Gilles, J., Robers, P., & Smith, F. (1951). Calorimetric determination of sugar and related substance. Analyt Chemistry, 26(3), 351–356. https://doi.org/10.1021/ac60111a017
- Elbeltagi, A., Aslam, M. R., Malik, A., Mehdinejadiani, B., Srivastava, A., Bhatia, A. S., & Deng, J. (2020). The impact of climate changes on the water footprint of wheat and maize production in the Nile Delta, Egypt. Science of the Total Environment, 743, 140770. https://doi.org/10.1016/j.scitotenv.2020.140770
- El-Hamdi, K. H., Khair, A., & Abu Ishwayrib, H. (2020). Simulation of water stress and climate change impacts on canola yield, seed quality and water productivity at North Delta, Egypt. Journal of Soil Sciences and Agricultural Engineering, 11(8), 377–383.
- Eroglu, N., Emekci, M., & Athanassiou, C. G. (2017). Applications of natural zeolites on agriculture and food production. Journal of the Science of Food & Agriculture, 97(11), 3487–3499.
- Geriesh, M. H., Abouelmagd, A., & Mansour, B. M. (2023). Major groundwater reservoirs of Egypt. In The phanerozoic geology and natural resources of Egypt (pp. 613–635). Springer.
- Hh Khalifa, T., Elsaka, M. S., Shabana, M. A., & Abo-Elsoud, H. M. (2019). Effect of zeolite and mineral fertilizers on some soil properties and growth of jew’s mallow in clayey and sandy soils. International Journal of Plant & Soil Science, 31(2), 1–12.
- Jackson, M. (1958). Inc Englewood Cliffs NJ, 498, 183–204.
- Johns, C. (2017). Fut Direct Intl, 1.
- Kalra, A., Grover, R., Rishi, N., & Khurana, S. P. (1989). Interaction between phytophthora infestans and potato viruses X and Y in potato. The Journal of Agricultural Science, 112(1), 33–37.
- Kamal, R., & Hashim, M. (2023). Evaluation of Water Resources Impact on Agricultural Economic Development in Egypt. Evaluation 19(01).
- Kesari, K. K., Soni, R., Jamal, Q. M. S., Tripathi, P., Lal, J. A., Jha, N. K., Siddiqui, M. H., Kumar, P., Tripathi, V., & Ruokolainen, J. (2021). Wastewater treatment and reuse: A review of its applications and health implications. Water, Air, and Soil Pollution, 232(5), 1–28. https://doi.org/10.1007/s11270-021-05154-8
- Khodeir, M. H., & Abdelsalam, H. M. (2016). Simulating corn supply, demand and consumption in Egypt: A system dynamics approach. Proceedings of the 10th International Conference on Informatics and Systems, Giza, Egypt.
- Klute, A. (1986). Methods of Soil Analysis.
- Kulikova, A., Zakharov, N., Yashin, E., & Khairtdinova, N. (2020). Efficiency of zeolite as maize fertilizer of middle volga chernozem. BIO Web of Conferences, EDP Sciences.
- Lee, J. J., Park, R. D., Kim, Y. W., Shim, J. H., Rim, D. H. C. S., Sohn, B. K., Kim, T. H., & Kim, Y. (2004). Effect of food waste compost on microbial population, soil enzyme activity and lettuce growth. Biores Technol, 93(21), 28.
- Lemanowicz, J., Bartkowiak, A., Zielińska, A., Jaskulska, I., Rydlewska, M., Klunek, K., & Polkowska, M. (2023). The effect of enzyme activity on carbon sequestration and the cycle of available macro-(p, k, mg) and microelements (zn, cu) in phaeozems. Agriculture, 13(1), 172.
- Lima, C., Bieseki, L., Melguizo, P. V., & Pergher, S. B. C. (2019). Environmentally friendly zeolites. Springer.
- López-Piñeiro, A., Peña, D., Albarrán, A., Becerra, D., & Sánchez-Llerena, J. (2013). Sorption, leaching and persistence of metribuzin in Mediterranean soils amended with olive mill waste of different degrees of organic matter maturity. Journal of Environmental Management, 122, 76–84. https://doi.org/10.1016/j.jenvman.2013.03.006
- Majchrzak, L., Niewiadomska, A., & Natywa, M. (2014). Evaluation of dehydrogenase activity of spring barley depending on the tillage system, previous crop and type of crop residue. Annales Universitatis Mariae Curie-Skłodowska Sectio E, Agricultura, 69(4), 103–111.
- Martínez-Rodríguez, Y., Acosta-Muñiz, C., Olivas, G. I., Guerrero-Beltrán, J., Rodrigo-Aliaga, D., Mujica-Paz, H., Welti-Chanes, J., & Sepulveda, D. R. (2014). Effect of high hydrostatic pressure on mycelial development, spore viability and enzyme activity of penicillium roqueforti. International Journal of Food Microbiology, 168, 42–46. https://doi.org/10.1016/j.ijfoodmicro.2013.10.012
- Mkhinini, M., Boughattas, I., Alphonse, V., Livet, A., Gıustı-Mıller, S., Bannı, M., & Bousserrhıne, N. (2020). Heavy metal accumulation and changes in soil enzymes activities and bacterial functional diversity under long-term treated wastewater irrigation in East Central region of Tunisia (Monastir governorate). Agricultural Water Management, 235, 106150. https://doi.org/10.1016/j.agwat.2020.106150
- Narasimha, G., Sridevi, A., Reddy, A. V. S., & Reddy, B. R. (2011). Effects of cotton ginning mill effluents on soil enzymatic activities and nitrogen mineralization in soil. J Chem Pharm Res, 3(1), 128–137.
- Page, A. L. (1982). Methods of soil analysis: Chemical and microbiological properties. American Society of Agronomy.
- Saba, B., Christy, A. D., Yu, Z., & Co, A. C. (2017). Sustainable power generation from bacterio-algal microbial fuel cells (MFCs): An overview. Renewable and Sustainable Energy Reviews, 73, 75–84. https://doi.org/10.1016/j.rser.2017.01.115
- Senthilkumar, M., Amaresan, N., Sankaranarayanan, A., Senthilkumar, M., Amaresan, N., & Sankaranarayanan, A. (2021). Quantitative estimation of nitrogenase activity: Acetylene reduction assay. Springer.
- Shahi Khalaf Ansar, B., Kavusi, E., Dehghanian, Z., Pandey, J., Asgari Lajayer, B., Price, G. W., & Astatkie, T. (2023). Removal of organic and inorganic contaminants from the air, soil, and water by algae. Environmental Science and Pollution Research, 30(55), 116538–116566.
- Shivakumara, M. N., Krishna murthy, R., Subbarayappa, C. T., Chamegowda, T. C., Thimmegowda, M. N., & Muthuraju, R. (2019). Effect of zeolite and fertilizer application on soil microbial biomass and enzyme activity in finger millet. Journal of Current Microbiology and Applied Sciences, 8(11), 2319–7706.
- Solaiman, Z. (2007). Measurement of microbial biomass and activity in soil. In A. Varma & R. Oelmüller (Eds.), Advanced techniques in soil microbiology (pp. 201–211). Springer.
- Sosulski, F. W., & Imafidon, G. I. (1990). Amino acid composition and nitrogen-to-protein conversion factors for animal and plant foods. Journal of Agricultural & Food Chemistry, 38(6), 1351–1356.
- Stern, R. (1991). CoStat-statutical software. California: CoHort software (1989), pp. 302, $76.00. Experimental Agriculture, 27(1), 87–87.
- Szatanik-Kloc, A., Szerement, J., Adamczuk, A., & Józefaciuk, G. (2021). Effect of low zeolite doses on plants and soil physicochemical properties. Materials, 14(10), 2617.
- Tabatabai, M. A., & Bremner, J. M. (1969). Use of p-nitrophenyl phosphate for assay of soil phosphatase activity. Soil Biology and Biochemistry, 1(4), 301–307.
- Thimmegowda, M., & Muthuraju, R. (2019). Effect of zeolite and fertilizer application on soil microbial biomass and enzyme activity in finger millet. Int J Curr Microbiol App Sci, 8(11), 1939–1957.
- Valchev, D., & Ribarova, I. (2022). A review on the reliability and the readiness level of microalgae-based nutrient recovery technologies for secondary treated effluent in municipal wastewater treatment plants. Processes, 10(2), 399.
- Wang, L., & Zhang, B. (2022). Integrated Wastewater Management and Valorization Using Algal Cultures, Elsevier, 235–264. https://doi.org/10.1016/B978-0-323-85859-5.00006-3
- Wolińska, A., & Stępniewska, Z. (2012). Dehydrogenase activity in the soil environment. Dehydrogenases, 10, 183–210. https://doi.org/10.5772/48294
- Zhang, Z., Xu, M., Fan, Y., Zhang, L., & Wang, H. (2024). Using microalgae to reduce the use of conventional fertilizers in hydroponics and soil-based cultivation. Science of the Total Environment, 912, 169424. https://doi.org/10.1016/j.scitotenv.2023.169424
- Zorpas, A. A., Pedreño, J. N., & Candel, M. B. A. (2021). Heavy metal treatment and removal using natural zeolites from sewage sludge, compost, and agricultural soils: A review. Arabian Journal of Geosciences, 14(12), 1098.