ABSTRACT
We investigated the genetic and transcriptional changes associated with Clustered Regularly Interspaced Short Palindromic Repeats (CRISPR) associated protein 9 (Cas9) expression in human cancer cell lines. For a subset of cell lines with a wild-type tumor protein TP53 (best known as p53), we detected p53 pathway activation, DNA damage accumulation and emerging p53-inactivating mutations following Cas9 introduction. We discuss the potential implications of our findings in basic and translational research.
Clustered regularly interspaced short palindromic repeats (CRISPR)-associated protein 9 (Cas9) has become the preferred method for genome-editing. Its straightforward mode of use, affordability, versatility and multiplexing capacity render CRISPR-Cas9 popular in basic science and has made it a top candidate technology for gene therapy. With an increasing number of successful CRISPR-Cas9 screens published, however, a link between prolonged Cas9 activity and genotoxic stress has also been observed.1,Citation2 In a recent study we investigated whether Cas9 expression in and of itself was sufficient to cause genetic and transcriptional alterations in cell line models prior to CRISPR-Cas9-directed gene editing.Citation3
We first conducted a transcriptional characterization of 165 pairs of wild-type and Cas9-expressing human cancer cell lines using the L1000 assay.Citation4 Expression profiling revealed that ~12% of the average cell line transcriptome had been altered by at least two-fold following Cas9 introduction, and that this transcriptional change was larger than that seen when expressing reporters or empty vector controls. Gene Set Enrichment AnalysisCitation5 of pairwise Cas9 vs. parental differential expression signatures revealed significant activation of the tumor protein TP53 (best known as p53) pathway in ~15% of the evaluated Cas9-expressing cell lines, occurring in a third (33%) of the TP53 wild-type lines. We functionally validated this finding by measuring p53 and CDKN1A (best known as p21) protein expression, as well as mRNA expression of seven p53 transcriptional targets, in a range of TP53 wild-type and mutant cell lines. Indeed, we were able to validate Cas9-induced p53 pathway activation in wild-type but not mutant lines.
We next set out to determine the genetic consequences of Cas9 expression. Deep targeted exon sequencing of 42 wild-type and Cas9 cell line pairs revealed that Cas9 lines tend to acquire p53-inactivating mutations relatively often: in ~10% of the cell lines, a p53-inactivating mutation emerged or expanded in the population when Cas9-expressing lines were compared to their parental lines. We therefore hypothesized that p53 inactivation might promote proliferation and escape from cell cycle arrest following Cas9 introduction. Cell competition assays with isogenic TP53 wild-type and mutant lines transduced either with Cas9 or with a matched empty vector, confirmed that Cas9 expression increased the ability of the mutant cells to outcompete their wild-type counterparts. We conducted similar experiments with two additional tumor suppressors, but did not observe Cas9-induced selection, suggesting that the effect was specific to TP53.
From a biological perspective, the mechanism by which Cas9 activates the p53 pathway remains unclear (). We identified elevated DNA damage levels (measured by quantifying γH2AX foci) in cells transduced with Cas9. Why do DNA double-strand breaks accumulate in Cas9-expressing cells despite the absence of a sgRNA that could direct the endonuclease to the DNA? Does DNA cutting occur with equal probability across the genome or are there hotspots for this nonspecific cutting? How do p53 pathway activation and DNA damage accumulation change over time? Would lower levels of Cas9 expression (e.g., by a weaker promoter), or transient expression of the enzyme, alleviate p53 pathway activation? Experiments with catalytically-dead Cas9, multiple forms of Cas9 introduction, as well as additional Cas species, may begin to address these open questions.
Figure 1. Implications of Cas9-induced p53 signaling. A summary of the biological, practical and clinical concerns associated with CRISPR associated protein 9 (Cas9)-induced tumor protein TP53 (p53) signaling. These concerns raise open questions that need to be tackled: from a basic science perspective, it will be important to understand the mechanisms that lead to nonspecific DNA cutting and to p53 signaling; from a routine lab work perspective, it will be helpful to determine best practices to monitor and alleviate Cas9-induced signaling; from a therapeutics perspective, it will be important to evaluate this issue in clinically-relevant settings. dCas9, catalytically-dead Cas9.
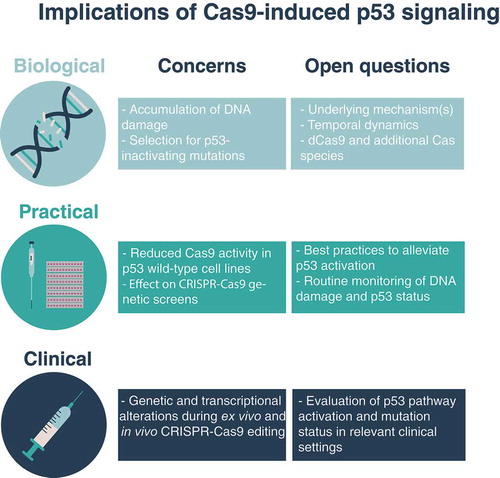
From a practical perspective, our findings call for an assessment of p53 status and pathway activity for the correct interpretation of CRISPR-Cas9-related analyses (). Previous studies have suggested a p53-dependent efficiency rate of CRISPR-Cas9 screens, as well as the accumulation of DNA damage with constant Cas9 expression in the presence of DNA-cutting sgRNAs.Citation1,Citation2,Citation6 We compared Cas9 activity levels across hundreds of TP53 wild-type and mutant cell lines and observed that Cas9 activity was indeed lower in the wild-type lines, confirming that p53 was a barrier for Cas9 activity. Comparison of genetic dependency scores between CRISPR-Cas9 and RNA interference genetic perturbation screens additionally showed that the agreement between the screening modalities was lower on average for TP53 wild-type cell lines.Citation7,Citation8 By functional annotation enrichment analysis, we identified the discordant genetic dependencies to be enriched for genes involved in DNA replication, DNA damage repair, RNA processing and viral transcription. These findings suggest that TP53 wild-type cell lines may be more dependent on functional DNA repair machinery in the presence of active Cas9. Importantly, careful experimental design and data analysis can overcome this hurdle for improved CRISPR-Cas9 screening in TP53 wild-type cells.Citation9,Citation10
Finally, from a clinical perspective, CRISPR-Cas9 technology is predicted to transform the field of gene therapy and enable a new avenue for therapeutics of genetic diseases. Clinical trials that make use of this technology have been initiated in the United States for the treatment of cancer, blood and eye disorders (ClinicalTrials.gov identifiers NCT03399448, NCT03745287 and NCT03872479, respectively). These trials will provide essential information on wanted and unwanted effects associated with specific and nonspecific DNA cutting, delivery of the CRISPR-cas9 machinery into human cells, and immune response. Our findings highlight the need to examine p53 pathway activation and acquisition of p53-inactivating mutations in the clinical setting (). Potential approaches could include the monitoring of genetic and transcriptional effects associated with in vivo CRISPR-Cas9-mediated editing in preclinical experiments in mice, as well as in human cells that are edited ex vivo prior to their re-introduction into a human recipient. Ultimately, the improved understanding of the desired and undesired effects of CRISPR-Cas9 gene editing will facilitate optimal use of this groundbreaking technology, both in basic science and for effective therapeutics.
Acknowledgments
Research in the laboratory of U.B.-D. is supported by the Azrieli Foundation, the Richard Eimert Research Fund on Solid Tumors, the Tel-Aviv University Cancer Biology Research Center, and the Israel Cancer Association.
Additional information
Funding
References
- Haapaniemi E, Botla S, Persson J, Schmierer B, Taipale J. CRISPR-Cas9 genome editing induces a p53-mediated DNA damage response. Nat Med. 2018;24(7):1–3. doi:10.1038/s41591-018-0049-z.
- Ihry RJ, Worringer KA, Salick MR, Frias E, Ho D, Theriault K, Kommineni S, Chen J, Sondey M, Ye C, et al. p53 inhibits CRISPR-Cas9 engineering in human pluripotent stem cells. Nat Med. 2018;24(7):939–946. doi:10.1038/s41591-018-0050-6.
- Enache OM, Rendo V, Abdusamad M, Lam D, Davison D, Pal S, Currimjee N, Hess J, Pantel S, Nag A, et al. Cas9 activates the p53 pathway and selects for p53-inactivating mutations. Nat Genet. 2020 May;18:1–7. doi:10.1038/s41588-020-0623-4.
- Subramanian A, Narayan R, Corsello SM, Peck DD, Natoli TE, Lu X, Gould J, Davis JF, Tubelli AA, Asiedu JK, et al. A next generation connectivity map: L1000 platform and the first 1,000,000 profiles. Cell. 2017;171(6):1437–1452.e17. doi:10.1016/j.cell.2017.10.049.
- Subramanian A, Tamayo P, Mootha VK, Mukherjee S, Ebert BL, Gillette MA, Paulovich A, Pomeroy SL, Golub TR, Lander ES, et al. Gene set enrichment analysis: a knowledge-based approach for interpreting genome-wide expression profiles. Proc Natl Acad Sci. 2005;102(43):15545–15550. doi:10.1073/pnas.0506580102.
- Schiroli G, Conti A, Ferrari S, Della Volpe L, Jacob A, Albano L, Beretta S, Calabria A, Vavassori V, Gasparini P, et al. Precise gene editing preserves hematopoietic stem cell function following transient p53-mediated DNA damage response. Cell Stem Cell. 2019;24(4):551–565.e8. doi:10.1016/j.stem.2019.02.019.
- Dempster JM, Rossen J, Kazachkova M, Pan J, Kugener G, Root DE, Tsherniak A. Extracting biological insights from the Project Achilles genome-scale CRISPR screens in cancer cell lines. bioRxiv. 2019 Jul;31:720243. doi:10.1101/720243.
- McFarland JM, Ho ZV, Kugener G, Dempster JM, Montgomery PG, Bryan JG, Krill-Burger JM, Green TM, Vazquez F, Boehm JS, et al. Improved estimation of cancer dependencies from large-scale RNAi screens using model-based normalization and data integration. Nat Commun. 2018;9(1):4610. doi:10.1038/s41467-018-06916-5.
- Bowden AR, Morales-Juarez DA, Sczaniecka-Clift M, Agudo MM, Lukashchuk N, Thomas JC, Jackson SP. Parallel CRISPR-Cas9 screens clarify impacts of p53 on screen performance. Marine J-C, Cheah KSE, Ventura A, Fernandez-Capetillo O, editors. eLife. 2020;9:e55325. doi:10.7554/eLife.55325.
- Sinha S, Guerra KB, Cheng K, Leiserson MD, Wilson DM, Ryan BM, Ronai ZA, Lee JS, Deshpande AJ, Ruppin E. Integrated computational and experimental identification of p53, KRAS and VHL mutant selection associated with CRISPR-Cas9 editing. Cancer Biol. 2018. accessed 2020 Jun 18 http://biorxiv.org/lookup/doi/10.1101/407767