Abstract
Background: Standard diagnostic methods for lower respiratory tract infections are currently too slow and insensitive to guide early clinical decisions concerning treatment and isolation. Syndrome-specific, diagnostic panels have potential to provide information about aetiology quickly. Available panels have been of limited use in lower respiratory tract infections due to slow turn-around-time, lack of quantification of important pathogens and lack of detection of resistance genes.
Materials/methods: We evaluated the newly developed Biofire® Filmarray® Pneumonia Panel plus (Biomérieux). Eighty-eight consecutive lower respiratory tract samples were analyzed by both standard microbiological methods, as requested by the referring clinician, and by the panel. The agreement with standard methods, empirical treatment coverage and possible impact on isolation practices were assessed by comparing the results from standard diagnostic methods with the panel results in relation to clinical data and information of antimicrobial therapy.
Results: Both qualitative and semi-quantitative results from the panel generally displayed good agreement with standard methods and by combining methods, a possible aetiology was detected in 73% of patients. Due to the panel approach, the panel detected viruses more frequently. In 25% of the 60 patients assessed for empirical treatment coverage, a pathogen not covered by current therapy was detected and in 30% of in-house patients the panel results were found to potentially influence clinical decisions related to isolation care.
Conclusions: The new diagnostic panel shows promise in improving aetiological diagnostics of lower respiratory tract infections. Correctly applied it has potential to offer support in clinical decision-making within hours of sampling.
Introduction
Lower respiratory tract infection (LRTI) is an important cause of morbidity and mortality worldwide [Citation1]. Traditional diagnostic methods for LRTI are currently too slow and insensitive to guide early clinical decisions concerning choice of antimicrobial treatment and the need of isolation/cohort care. Even though the proportion of patients who are subjected to comprehensive diagnostic testing has increased, a possible aetiology was identified in less than half of pneumonia patients treated at infectious disease clinics in Sweden 2017, according to the Swedish registry for community-acquired pneumonia (CAP) [Citation2]. Molecular diagnostics of LRTI is difficult, due to the non-sterile environment of the respiratory tract, and the overlap between colonizing and disease-causing pathogens. Recent years’ development in molecular diagnostic methods has led to a markedly increased availability of both commercial and in-house developed diagnostic panels targeting viruses and atypical bacterial pathogens [Citation3]. However, examples of panels targeting typical respiratory bacteria are few [Citation4–6], and molecular panels intended for LRTI diagnostics are even more scarce [Citation7–10]. Methods combining targets for typical bacterial pathogens, atypical bacteria and viruses have so far been limited to qPCR-based panels, requiring a separate sample extraction step [Citation8–10], which limit their use to larger clinical microbiology laboratories during working hours. In addition, these combined panels lack semi-quantification of bacterial targets, which might enable differentiation of infection from colonization, and/or analysis of important antimicrobial resistance genes. LRTIs, such as pneumonia, are commonly treated empirically with broad-spectrum antimicrobial therapies, although general use of such therapies have not proven to improve outcomes for patients [Citation11]. On the contrary, broad-spectrum antimicrobial treatment have been identified as an independent risk factor for both increased mortality and complications, such as Clostridium difficile infection, in hospital-treated patients [Citation12]. Since they are also ecologically unfavourable, it is both in the interest of the patient and the society to decrease the use of these drugs [Citation13].
Rapid molecular testing has potential to reduce the use of broad-spectrum empirical treatment in LRTI [Citation14], but the results are so far conflicting [Citation3]. The clinical value of a rapid diagnostic test is likely dependent on multiple factors, such as local antimicrobial prescription practices, severity of the infection, antimicrobial resistance (AMR) patterns as well as clinical routines concerning isolation and cohort care. The Biofire® Filmarray® Pneumonia panel plus (Biomérieux) is a newly developed, commercially available diagnostic panel for LRTI, targeting 18 bacterial pathogens, 9 viruses and 7 AMR genes, approved by the United States Food and Drug Administration. It has an integrated sample preparation step, which limits the hands-on time to less than 5 min, and a run-time of about 1 h.
Our aim of this study was to evaluate the possible clinical value of the Biofire pneumonia panel plus, through assessment of agreement with standard methods and evaluation of empirical treatment coverage and possible impact on isolation practices, related to the findings of the panel.
Materials and methods
Clinical setting, patients and samples
The study was conducted at Umeå University hospital, (Umeå, Sweden) as a prospective, method-comparison study using anonymized, clinical data available from the laboratory referral. Respiratory tract specimens: Sputum, trach aspirates and broncho-alveolar lavage (BAL), sent for respiratory infection diagnostics to the Clinical Microbiology laboratory, Umeå University hospital, with a clinical suspicion of acute LRTI, were consecutively included between June and September 2018. No exclusion criteria were applied. From the laboratory referral, the following data was recorded: Age, sex, referring clinic, description of the patient’s medical condition, current or planned antimicrobial treatment (when available) and type of specimen. Due to the study design, no further assessment of the patients’ medical records, including treatment outcome and final diagnosis, was possible. The results from both the Biofire pneumonia panel plus and standard methods, as described below, were also recorded. The study was performed in accordance with the Declaration of Helsinki. An ethical permission was not required since the samples and collected clinical data were anonymized before being obtained by the authors.
Standard diagnostic methods
Sputum, trach aspirates and BAL specimens were cultured for isolation of typical bacteria causing respiratory infections, according to standard methods [Citation15]. Sputum Gram stain was used to assess the ratio of leukocytes and epithelia cells. Only samples with a leukocyte:epithelial cell ratio ≥1:1 were cultured. All sputum samples from patients with cystic fibrosis, immunocompromised patients and from patients with lobar pneumonia were cultured without assessing the leukocyte count. The samples were homogenized using an equal volume of N-acetylcysteine solution and 10 µl of the sample and of a 1:100 dilution were plated on blood and McLeod agar and incubated at 36 °C. For patients with cystic fibrosis, additional culturing on Cysteine Lactose Electrolyte Deficient agar, Peptone Yeast agar, Burkholderia Cepacia Selective agar, Sabouraud Dextrose agar and S. aureus CHROM-agar plates was performed. Bacteria were semi-quantified by calculating colony-forming units (CFU) on the dilution-plates. For this evaluation, a cut-off of 105 CFU/ml for significance was used for all samples.
BAL and trach aspirates: Viscous trach aspirates were homogenized similar to sputum samples, as described above. 10 µl BAL or homogenized trach aspirate were plated on blood-, Sabouraud Dextrose, McLeod- and anaerobe blood agar plates and incubated at 35 °C (Sabouraud Dextrose agar: 30 °C). For cystic fibrosis patients, additional culture on selective media was performed as described above. Bacteria from BAL-samples were semi-quantified by calculating the CFU on the plates. For this evaluation a cut-off of 104 CFU/ml for significance was used. For trach aspirates, no semi-quantification was performed.
Identification to species level was performed by matrix-assisted laser desorption/ionization time-of-flight mass spectrometry and the Biotyper 2.0 database (Bruker Daltronics, Bremen, Germany) [Citation16]. A score of ≥2 was accepted for identification. All isolates were tested for antimicrobial susceptibility according to the EUCAST disc diffusion method for relevant pathogens [Citation17]. Detection of extended spectrum beta-lactamases was done according to recommendations from Nordic Committee on Antimicrobial Susceptibility Testing [Citation18], and detection of methicillin resistant staphylococcus aureus (MRSA) by an in-house molecular method, described by Francois et al. [Citation19]
Viral- and atypical bacterial qPCR-assays were performed on request by the referring clinician, which explains why only a limited number of samples were analyzed by these methods. Nucleic acid from 400 µl of specimen was extracted by a QIAsymphony instrument (QIAGEN GmbH, Hilden, Germany) using the DSP viral/pathogen midi kit (QIAGEN), with an elution volume of 60 µl and 200 µl for atypical bacteria and viruses, respectively. Individual in-house qPCR assays were performed on a 7500 real-time PCR system (Applied Biosystems, Foster City, CA), reaction volume 25 µl, using 10 µl of nucleic acid; universal master mixes for either DNA or RNA (QIAGEN, Quantitect Probe PCR mix/Probe RT-PCR mix). In-house assays were performed as previously described for the detection of influenzavirus A and B [Citation20], adenovirus [Citation21], RS-virus [Citation22], metapneumovirus [Citation23], Mycoplasma pneumoniae and Chlamydophila pneumoniae [Citation24]. For the detection of enterovirus, parainfluenzavirus, coronavirus, rhinovirus and bocavirus the Allplex™ Respiratory panel 2 and 3 [Citation25] were used on a CFX96 instrument (BioRad, Hercules, CA) according to the manufacturer (Seegene, South Korea).
Prospective testing by the Biofire pneumonia panel plus
We evaluated the Biofire® Filmarray® Pneumonia panel plus (Biomérieux, Marcy l’Etoile, France), targeting 27 pathogens and 7 antimicrobial resistance genes, with an analysis time of about 67 min, including sample preparation and DNA/RNA extraction. The panel was performed according to the manufacturer´s instructions [Citation26]. Each test included two internal controls: (1) to verify nucleic acid extraction and (2) to verify PCR performance. If both controls passed, the run was considered valid. The panels were run on the FilmArray® Torch systems. Typical colonizing bacteria were reported semi-quantitatively at ≥107, 106, 105 and 104 copies/ml of specimen, where 104 copies/ml and 105 copies/ml were determined as significant for BAL/trach aspirates and sputum, respectively. Viral, fungal and atypical bacterial detections were reported as not detected or detected. AMR-genes were reported as positive, only in the case of simultaneous detection of a compatible pathogen, i.e. S. aureus in combination with mecA/mecC and MREJ. Targets of the panel are specified in . The samples were prospectively analyzed, but no results made available to the referring clinician, due to the investigation only use status of the panel at the time of the study.
Table 1. Targets of the Biofire pneumonia panel plus.
Retrospective assessment of empirical antimicrobial therapy coverage and supposed impact on isolation practices
The coverage of empirical antimicrobial therapy and the supposed impact on isolation practices was assessed in retrospect by a physician experienced in diagnostics of respiratory diseases, not involved in the patients’ care (AE), by analyzing the results from the panel in relation to clinical data and information of antimicrobial therapy, stated on the laboratory referral. Impact variables assessed were empirical antimicrobial therapy coverage or non-coverage and isolation (only for in-patients). The algorithm for the assessment is displayed in . For patients where information of current antimicrobial therapy was available on the referral, the diagnostic findings of the Biofire pneumonia panel plus were assessed in relation to the specified treatment. For patients where no information about treatment was available, but a clinical diagnosis (e.g. CAP or ventilator-associated pneumonia) was stated on the referral, the diagnostic results were evaluated in relation to the first-line treatment for that particular diagnosis, according to Swedish guidelines [Citation27,Citation28]. In patients where no information of antimicrobial treatment, nor a clear clinical diagnosis was available, no assessment of empirical treatment coverage was performed. The impact on decisions on isolation or cohort care was evaluated for in-patients only. Since isolation is not routine practice at our hospital in patients where a LRTI is suspected, we assumed that none of the patients were isolated before the diagnostic results were available. In the case of a positive finding by the Biofire pneumonia panel plus of a pathogen where isolation or cohort care is recommended by Swedish guidelines [Citation27], the result was stated as “isolation” and as “no change” in all other cases.
Data analysis
Descriptive data analysis was done in Microsoft Excel 2016 (Microsoft corp., Redmond, WA, USA). Two-by-two contingency tables of categorical variables were analyzed by Fisher’s exact test to calculate positive percent agreement and negative percent agreement, positive predictive value and negative predictive value. Positive percent agreement and negative percent agreement was used instead of sensitivity and specificity, respectively, as recommended by the US Food and Drug administration [Citation29], when a non-perfect gold standard method is used as reference test, as is the case in LRTI diagnostics. The increased detection rate of the panel was tested for significance by a 2-sample test for equality of proportions with continuity correction. Confidence intervals for diagnostic accuracy parameters were calculated using the Wilson–Brown method in Graphpad Prism version 8.1.1 (Graphpad, CA, USA).
Results
Patient characteristics
Out of 88 initially analyzed samples, 4 were excluded from further data analysis due to lack of a clear clinical suspicion of an acute lower respiratory infection, upon secondary assessment of the referral data, since they did not fulfil the inclusion criteria. In these four cases, either clinical data were missing entirely or sampling was conducted as part of a non-acute clinical investigation, i.e. malignancy. Descriptive patient data of enrolled samples is specified in . Of note, 19 out of 21 patients enrolled from the intensive care unit were men. In 34 patients (41%) the preliminary diagnosis, as indicated on the referral, was pneumonia, including 5 cases of ventilator-associated and hospital-acquired pneumonia. Fourteen patients (17%) had symptoms of an acute exacerbation of a chronic lung disease such as bronchiectasis, chronic obstructive pulmonary disease or cystic fibrosis. The remaining 36 patients 43% had an unspecified LRTI, according to the referral. Sixty-one samples (73%) were positive for any organism, when results from both standard methods and the Biofire pneumonia panel plus were included, as compared to 50 samples (60%) by standard methods only. The difference was not statistically significant (p = .0516). Thirty-seven percent of the samples were positive for more than one pathogen.
Table 2. Descriptive data of enrolled patients.
Comparison of the Biofire pneumonia panel plus to standard diagnostic methods
Findings by standard methods and the Biofire pneumonia panel plus, specified by pathogen, is displayed in . The most common pathogen detected, by both standard methods and the panel, was H. influenzae, followed by rhino/enterovirus, S. aureus and S. pneumoniae. For S. aureus, adenovirus and rhino/enterovirus, the Biofire pneumonia panel plus accounted for a majority of the total number of positives. Since relatively few of the samples were analyzed by standard methods for viruses and atypical bacteria, a higher proportion of these findings were unique to the Biofire pneumonia panel plus. A total of 24 bacterial findings were detected below cut-off by both methods (these were excluded from further analysis). These included: S. aureus (N = 8), H. influenza (N = 8), S. pneumoniae (N = 5) and M. catarrhalis (N = 1). Additional positive findings of pathogens not included in the panel by standard methods included Candida spp (N = 9), α-hemolytic streptococci (N = 8), Stenotrophomonas maltophila (N = 2) and Bordetella pertussis (N = 2). There were no positive results of influenza A/B, K. aerogenes, Proetus spp, Acinetobacter, metapneumovirus, MERS-CoV or C. pneumoniae by either method. A summary of the diagnostic accuracy parameters can be found in . When comparing agreement in identification of an aetiology between methods for all samples, irrespectively of whether or not the identified pathogen was included in the panel, positive percent agreement was 86.0% while negative percent agreement was 67.7%. Upon exclusion of pathogens not included in the panel from the analysis, positive percent agreement as well as the negative predictive value was as high as 100%. The lower negative percent agreement of 73.2% was mainly explained by increased detection of H. influenzae, S. aureus and rhino/enterovirus. Three samples positive for MRSA were identified by both the panel and by standard methods, no other antimicrobial resistance genes were detected.
Table 3. Summary of findings from the Biofire Pneumonia panel plus (BP) and standard diagnostic methods.
Table 4. Comparison of the Biofire pneumonia panel plus (BP) to standard methods.
In 25 out of 33 samples where semi-quantitative result were available for both the panel and for culture, the difference between the two methods was 1 log10 or less. As anticipated, the measured concentration of bacterial DNA was higher or equal to the culture-determined CFU/ml in all samples, .
Figure 2. Comparison between the concentrations reported from culture diagnostics (CFU/ml) and the semi-quantitative results from the Biofire Pneumonia panel plus (BP), (DNA copies/ml). The figure shows all 33 results where a semi-quantitative result, above cut-off, was available from both methods for the same target.
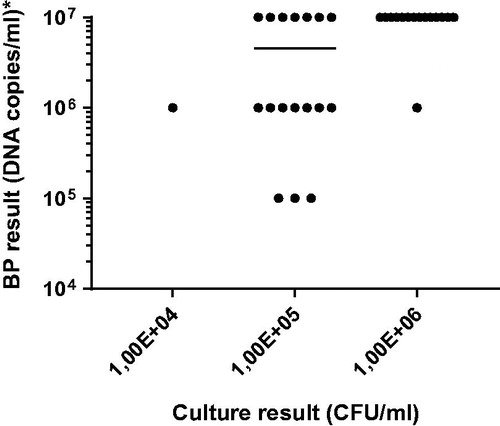
Assessment of empirical treatment coverage and supposed impact on isolation practices
Sixty samples, where either a specified treatment and/or information about clinical diagnosis was available, were assessed for empirical antimicrobial therapy coverage according to the algorithm outlined in . Twenty-eight patients were excluded from further analysis, since no bacterial pathogen was detected by the panel. In 17 cases (28%) the pathogen(s) detected was judged to be covered by the empirical therapy, in 7 of these patients, a therapy with a broader antimicrobial spectrum than motivated by the findings of the panel was used. In 15 patients (25%) a pathogen was detected by the panel which was not covered by the empirical antimicrobial treatment: H. influenzae (N = 4), S. aureus (N = 4, including MRSA) S. marcescens (N = 1), M. pneumoniae (N = 1), P. aeruginosa (N = 2) and coinfections with multiple bacteria (N = 3). Seventy-nine samples, all in-house patients, were assessed for impact on decision of isolation or cohort care of the patient. In 24 cases (30%), the Biofire pneumonia panel plus identified a pathogen were isolation is recommended, according to Swedish guidelines [Citation27]. Most of these cases were samples positive for rhino/enterovirus (N = 13). When rhino/enterovirus as a cause for isolation was excluded, 9 patients (11%) were judged to require isolation/cohort care.
Discussion
Aetiological diagnosis of LRTI is a challenging area of clinical microbiology, due to the wide range of potential pathogens and the non-sterile environment of the respiratory tract. In this study, we evaluated a newly developed, syndrome-specific, diagnostic panel for LRTI, for agreement with standard methods and retrospectively analyzed the empirical antimicrobial treatment coverage and potential impact on decisions concerning isolation care. We found the panel generally to provide diagnostic results of good agreement with standard methods, with high negative predictive values of in-panel targets and a markedly shorter analysis time, a few hours as compared to 3–7 days for culture-based methods.
Gadsby et al., among others, have shown that rapid aetiological diagnostics might favourably impact treatment decisions in severe LRTI [Citation14]. For optimal access to test results, however, the usage should not be limited to large clinical microbiology laboratories, which requires minimal sample handling. Up until now, no such test have existed, to the best of our knowledge. The Biofire pneumonia panel plus evaluated in this study, might have potential to fulfil these criteria. In our evaluation, the positive percent agreement of the panel for in-panel targets was high, 100%, which was reflected by the fact that there were only 2 unique findings by standard methods, i.e. false negatives of the panel. The majority of findings by standard methods of pathogens not included in the panel were of unclear clinical significance, e.g. candida spp and α-hemolytic streptococci. The combination of high n percent agreement, i.e. a very low number of false negatives, and a broad coverage of relevant pathogens within the panel is most probably important for the method’s implementation in clinical practice. A problem of past diagnostic panels, including our previously developed qPCR-based panel for CAP pathogens [Citation9], has been an increased detection rate of common colonizers of the upper respiratory tract, in particular H. influenzae and S. aureus, which might be of unclear clinical significance. Although we applied the same concentration cut-off as for the corresponding culture method, to enable differentiation of colonization from infection, the same pattern was observed here. Six out of 22 findings for H. influenzae and 9 out of 13 findings for S. aureus were unique to the panel. These pathogens were also the most common pathogens not covered by the empirical antimicrobial therapy. Treatment failure in, for example CAP, is reported to be between 2.4–31% [Citation30]. Given the relatively low detection rate of plausible aetiologies in LRTI patients, with new and more sensitive diagnostic methods evolving, we might need to reconsider the clinical significance of these findings in the future. However, the design of this study excludes further analysis of the empirical treatment outcome and treatment decisions related to the panel findings, which is needed in order to speculate further on the clinical significance of the increased detection of H. influenzae and S. aureus.
The impact of a diagnostic test on antimicrobial therapy is dependent on the local treatment guidelines. In settings where a narrow-spectrum drug is used as first-line treatment in many cases of non-severe LRTI, such as Sweden [Citation27], or the Netherlands [Citation31], a higher detection of for example S. aureus or atypical bacterial pathogens will likely result in escalation of treatment, as compared to settings where these pathogens are routinely covered empirically, as is the case in the Unites States [Citation32]. In 15 of 60 patients assessed in this study, a pathogen was found which was not covered by the empirical antimicrobial treatment. To maximize the benefit from rapid diagnostic tests it is important that they are applied to the right group of patients, a concept known as diagnostic stewardship [Citation33]. In the case of syndromic testing for LRTI in our clinical setting, a panel such as the Biofire Pneumonia plus, would likely provide most benefit for patients with severe LRTI, such as severe CAP or ventilator-associated pneumonia, who are routinely prescribed broad-spectrum empirical treatment. If applied to a heterogeneous group of patients with LRTI, such as this study population, there is instead a risk of increased use of broad-spectrum therapies of unclear clinical benefit (or even harm), which is an important conclusion of our results.
A panel approach to diagnostics also leads to increased analysis, and detection, of respiratory viruses, as have been shown by for example Jain et al. 2015 [Citation34]. This was also the case in our evaluation, were 12 out of 18 findings of rhino virus and 3 out of 4 positives for adeno virus were unique to the Biofire pneumonia panel plus. Larger, prospective studies will be required to assess the clinical significance of the presence of these findings, since the role of viral and bacterial co-infection in LRTI is not clear. When it comes to clinical decisions concerning isolation or cohort care of patients with viral infections, however, this increased detection will be of immediate importance. In this study we chose to evaluate the clinical impact on isolation according to current Swedish guidelines which state that isolation or cohort care is recommended for patients with influenzavirus, RS-virus, metapneumovirus, coronavirus, rhinovirus, adenovirus, M. pneumoniae and C. psittaci [Citation27]. Considering that 19 out of 24 findings of these pathogens were unique to the panel, it will cause an substantial increase in isolation resources in a clinical setting were this is not routine practice for patients with LRTI.
Our study have a number of limitations. Firstly, it was not powered for method comparison of specific pathogens, were at least 20 positive and negative samples of each target pathogen of the panel is recommended for the validation of a new method [Citation35]. As an example, we had no positive findings of influenza virus of either method, which is of great importance to assess in LRTI diagnostics. Also, reflecting the low rate of AMR in our area, only three cases of MRSA were detected, and we had no positive findings of the other AMR-genes of the panel. Secondly, due to the data collection design, it was a strictly observational study, only allowing assessment of the clinical data provided with the referral to our laboratory, which limits the evaluation of rapid aetiological diagnostics as an intervention, as well as assessment on patient outcome variables and final diagnosis. The wide inclusion criteria also resulted in a heterogeneous study population, which complicate the interpretation of the findings. To further assess impact on patient outcomes, concerning treatment success or failure related to findings of different pathogens, and treatment related adverse events, as a consequence of changes in the clinical management, prospective and preferably randomized studies are needed.
In conclusion, our evaluation suggests that correctly applied, the Biofire pneumonia panel plus looks promising for improving aetiological diagnostics of LRTI, by offering good agreement of both qualitative and quantitative results with standard methods. It has potential to offer support in clinical decision-making with a markedly faster turn-around-time than standard methods, which is perhaps the most important advantage, as well as increased detection of viruses and atypical bacterial pathogens. The clinical relevance of the relatively high detection rate of H. influenzae, S. aureus and respiratory viruses, such as rhino/enterovirus and adeno virus, remains to be clarified.
Acknowledgements
We thank the staff at Clinical Microbiology, Umeå University Hospital for their contribution to the study, Patrik Rydén for statistical consultation and Biomérieux for providing equipment and technical support.
Disclosure statement
Biomérieux provided the test cartridges used in this study. No other economical support or other benefits were received by the authors from the company. Biomérieux designed the data collection strategy, but was not involved in the data analysis or the data interpretation process. The authors have no other conflict of interest to declare.
References
- Troeger C, Forouzanfar M, Rao PC, et al. Estimates of the global, regional, and national morbidity, mortality, and aetiologies of lower respiratory tract infections in 195 countries: a systematic analysis for the Global Burden of Disease Study 2015. Lancet Infect Dis. 2017;17(11):1133–1161.
- Nauclér P. Rapport Från Pneumoniregistret 2017. 2018. [cited 2020 Apr 13]. Available from: http://infektion.net/wp-content/uploads/2018/11/kvalreg_inf_2017_pneumoni.pdf.
- Vos LM, Bruning AHL, Reitsma JB, et al. Rapid molecular tests for influenza, respiratory syncytial virus, and other respiratory viruses: a systematic review of diagnostic accuracy and clinical impact studies. Clin Infect Dis. 2019;69(7):1243–1253.
- Pulido MR, Moreno-Martínez P, González-Galán V, et al. Application of BioFire FilmArray Blood Culture Identification panel for rapid identification of the causative agents of ventilator-associated pneumonia. Clin Microbiol Infect. 2018;24(11):1213.e1–1213.e4.
- Baudel J-L, Tankovic J, Dahoumane R, et al. Multiplex PCR performed of bronchoalveolar lavage fluid increases pathogen identification rate in critically ill patients with pneumonia: a pilot study. Ann Intensive Care. 2014;4(1):35.
- Ullberg M, Lüthje P, Mölling P, et al. Broad-range detection of microorganisms directly from bronchoalveolar lavage specimens by PCR/electrospray ionization-mass spectrometry. PLoS One. 2017;12(1):e0170033.
- Gadsby NJ, McHugh MP, Forbes C, et al. Comparison of Unyvero P55 Pneumonia Cartridge, in-house PCR and culture for the identification of respiratory pathogens and antibiotic resistance in bronchoalveolar lavage fluids in the critical care setting. Eur J Clin Microbiol Infect Dis. 2019;38(6):1171–1178.
- Gadsby NJ, McHugh MP, Russell CD, et al. Development of two real-time multiplex PCR assays for the detection and quantification of eight key bacterial pathogens in lower respiratory tract infections. Clin Microbiol Infect. 2015;21(8):e788.e1–788.e13.
- Edin A, Granholm S, Koskiniemi S, et al. Development and laboratory evaluation of a quantitative real-time PCR assay for detecting viruses and bacteria of relevance for community-acquired pneumonia. J Mol Diagnostics. 2015:17(3):315–324.
- Simusika P, Bateman AC, Theo A, et al. Identification of viral and bacterial pathogens from hospitalized children with severe acute respiratory illness in Lusaka, Zambia, 2011-2012. BMC Infect Dis. 2015;15(1):2011–2012.
- Haessler S, Lagu T, Lindenauer PK, et al. Treatment trends and outcomes in healthcare-associated pneumonia. J Hosp Med. 2017;12(11):886–891.
- Webb BJ, Sorensen J, Jephson A, et al. Broad-spectrum antibiotic use and poor outcomes in community-onset pneumonia: a cohort study. Eur Respir J. 2019;54(1):1900057.
- Llor C, Bjerrum L. Antimicrobial resistance: risk associated with antibiotic overuse and initiatives to reduce the problem. Ther Adv Drug Saf. 2014;5(6):229–241.
- Gadsby NJ, Russell CD, Mchugh MP, et al. Comprehensive molecular testing for respiratory pathogens in community-acquired pneumonia. Clin Infect Dis. 2016;62(7):817–823.
- Hallander H, Thore M, Olsson-Liljequist B, et al. Referensmetodik I 2: Nedre Luftvägsinfektioner. 2nd ed. Swedish Institute for Infectious disease Control. Stockholm: SBL; 2005. Available from: http://referensmetodik.folkhalsomyndigheten.se/w/Referensmetodik:_Nedre_luftvägsinfektioner,_2:a_upplagan_2005.
- Richter C, Hollstein S, Woloszyn J, et al. Evaluation of species-specific score cut-off values for various Staphylococcus species using a MALDI Biotyper-based identification. J Med Microbiol. 2012;61(Pt 10):1409–1416.
- Matuschek E, Brown DFJ, Kahlmeter G. Development of the EUCAST disk diffusion antimicrobial susceptibility testing method and its implementation in routine microbiology laboratories. Clin Microbiol Infect. 2014;20(4):O255–O266.
- Giske C. Nordic Committee on Antimicrobial Susceptibility Testing, Enterobacteriaceae: Detection of ESBL A/ESBLM/ESBLCARBA. [cited 2020 Apr 2]. Available from: http://nordicast.intranet2.se/d/2612?store_referer=true.
- Francois P, Pittet D, Bento M, et al. Rapid detection of methicillin-resistant Staphylococcus aureus directly from sterile or nonsterile clinical samples by a new molecular assay. J Clin Microbiol. 2003;41(1):254–260.
- Public Health Agency of Sweden. 2018. [cited 2020 Apr 13]. Available from: www.folkhalsomyndigheten.se.
- Hernroth BE, Conden-Hansson A-C, Rehnstam-Holm A-S, et al. Environmental Factors influencing human viral pathogens and their potential indicator organisms in the Blue Mussel, Mytilus edulis: the first Scandinavian report. Appl Environ Microbiol. 2002;68(9):4523–4533.
- Brittain-Long R, Nord S, Olofsson S, et al. Multiplex real-time PCR for detection of respiratory tract infections. J Clin Virol. 2008;41(1):53–56.
- Tiveljung-Lindell A, Rotzén-Östlund M, Gupta S, et al. Development and implementation of a molecular diagnostic platform for daily rapid detection of 15 respiratory viruses. J Med Virol. 2009;81(1):167–175.
- Gullsby K, Storm M, Bondeson K. Simultaneous detection of Chlamydophila pneumoniae and Mycoplasma pneumoniae by use of molecular beacons in a duplex real-time PCR. J Clin Microbiol. 2008;46(2):727–731.
- Lee J, Lee J. Performance evaluation of Allplex Respiratory Panels 1, 2, and 3 for the detection of respiratory viral infection. Clin Lab. 2019;65(1). DOI:10.7754/Clin.Lab.2018.180730
- Babady NE. The FilmArray® respiratory panel: an automated, broadly multiplexed molecular test for the rapid and accurate detection of respiratory pathogens. Expert Rev Mol Diagn. 2013;13(8):779–788.
- Athlin S, Lidman C, Lundqvist A, et al. Management of community-acquired pneumonia in immunocompetent adults: updated Swedish guidelines 2017. Infect Dis (Auckl). 2018;50(4):247–272.
- Sjukhusförvärvad pneumoni – behandlingsrekommendation. Information från Läkemedelsverket. Swedish Medical Products Agency. 2016;1(1):17–30. https://lakemedelsverket.se/pneumoni.
- FDA. Statistical guidance on reporting results from studies evaluating diagnostic tests. 2007 [cited 2020 Mar 30]. Available from: https://www.fda.gov/regulatory-information/search-fda-guidance-documents/statistical-guidance-reporting-results-studies-evaluating-diagnostic-tests-guidance-industry-and-fda
- Garcia-Vidal C, Carratalà J. Early and late treatment failure in community-acquired pneumonia. Semin Respir Crit Care Med. 2009;30(02):154–160.
- The Dutch Working Party on Antibiotic Policy (SWAB)/Dutch Association of Chest Physicians (NVALT). Dutch Guidelines on the Management of Community-Acquired Pneumonia in Adults. 2011. (November):1–95.
- Mandell L. a, Wunderink RG, Anzueto A, et al. Infectious Diseases Society of America/American Thoracic Society consensus guidelines on the management of community-acquired pneumonia in adults. Clin Infect Dis. 2007;44(Supplement_2):S27–S72.
- Messacar K, Parker SK, Todd JK, et al. Implementation of rapid molecular infectious disease diagnostics: the role of diagnostic and antimicrobial stewardship. J Clin Microbiol. 2017;55(3):715–723.
- Jain S, Self WH, Wunderink RG, et al. Community-acquired pneumonia requiring hospitalization among U.S. adults. N Engl J Med. 2015;373(5):415–427.
- Rabenau HF, Kessler HH, Kortenbusch M, et al. Verification and validation of diagnostic laboratory tests in clinical virology. J Clin Virol. 2007;40(2):93–98.