Abstract
Background
Currently, there is scant information regarding the features associated to the persistence of post-COVID-19 syndrome, which is the main aim of the present study.
Methods
A cohort study of 102 COVID-19 patients was conducted. The post-COVID-19 symptoms were assessed by a standardised questionnaire. Lymphocyte immunophenotyping was performed by flow cytometry and chemokines/cytokines, neutrophil extracellular traps, the tripartite motif 63, anti-cellular, and anti-SARS-CoV-2 IgG antibodies were addressed in serum. The primary outcome was the persistence of post-COVID-19 syndrome after six months follow-up.
Results
Thirteen patients (12.7%) developed the primary outcome and had a more frequent history of post-COVID-19 syndrome 3 months after infection onset (p = .044), increased levels of IL-1α (p = .011) and IP-10 (p = .037) and increased CD57 expression in CD8+ T cells (p = .003). There was a trend towards higher levels of IFN-γ (p = .051), IL-1β (p = .062) and IL-6 (p = .087). The history of post COVID-19 in the previous 3 months, obesity, baseline serum MIP-1α and IP-10, and CD57 expression in CD8+ T cells were independently associated with the persistence of post-COVID-19 syndrome.
Conclusion
Our data suggest an important relationship between a pro-inflammatory state mediated through metabolic pathways related to obesity and increased cellular senescence as a key element in the persistence of post-COVID-19 syndrome at six months of follow-up.
Introduction
Similarly to post-acute viral syndromes described in survivors of other virulent coronavirus epidemics, there are increasing reports of persistent and prolonged symptoms after acute coronavirus disease 2019 (COVID-19) [Citation1]. According to a recent systematic review of the literature, COVID-19 leads to multi-organ sequelae beyond the acute phase of infection [Citation1]. Although the nomenclature is still controversial, distinct clinical entities have been described to encompass the set of symptoms that initiate or persist after the acute SARS-CoV-2 infection. Post-COVID-19 syndrome/condition/long-COVID and long haulers’ syndrome are terms used to define a clinical entity in which the symptoms persist 12 weeks after the acute infection. This entity overlaps with the term post-acute sequelae of COVID-19 (PASC), which is used for symptoms lasting over four weeks after the resolution of the infection [Citation2]. Furthermore, to fulfil the mentioned definitions, the symptoms should be present for at least two months and should not be explained by an alternative diagnosis [Citation3].
Currently, there is not a consensus regarding which symptoms or outcomes should be assessed in these patients [Citation4,Citation5], and there is not a gold standard for the evaluation of the symptoms of PASC [Citation5]. Besides, the symptoms may fluctuate or relapse throughout time [Citation4]. These factors, along with the lack of a standard case definition, have limited the research about the epidemiology and risk factors for PASC [Citation4]. As a consequence, some studies have reported a wide range of prevalence of this entity (4.7–80%) at 3–24 weeks of follow-up [Citation6]. The PASC affect all kind of COVID-19 patients including those asymptomatic, but are more frequent in patients requiring hospitalisation during the acute infection (80%) [Citation7], in comparison to 11% of ambulatory subjects [Citation8]. PASC include pulmonary/cardiovascular (dyspnoea/persistent oxygen requirement), neuropsychiatric, musculoskeletal, renal, and thromboembolic features that have an impact on everyday functioning [Citation3,Citation9].
Although the pathogenesis of PASC is unknown, immunologic aberrations, inflammatory damage, and the expected sequelae of critical illness are potential factors involved in the development of this entity [Citation9]. Certain markers of COVID-19 severity such as neutrophil extracellular traps (NETs) [Citation10] and autoimmune phenomena like the development of anti-cellular antibodies [Citation11,Citation12] have been proposed as risk factors for the development of PASC. Also, the tripartite motif (TRIM) 63 ubiquitin ligase, which is a marker of muscle atrophy and has been associated with severe COVID-19 [Citation13] might be related to the presence of muscle symptoms in this entity.
A distinctive immunological profile in patients with post-COVID-19 syndrome has been described. It includes T cell compartment alterations involving low percentage of naïve, central memory, effector memory and terminally differentiated CD4+ T cells, an increased expression of CD57 in CD8+ T cells, and a high proportion of CD27+CD38lo/- B cells [Citation14,Citation15]. Also, CXCL10/IP-10, interferon β (IFN-β), IFN-λ1, IFN-γ, CXCL9, and interleukin-8 (IL-8) have been observed to remain elevated 4 months after the COVID-19 acute phase, while only IFN-β, PTX3, IFN-γ, IFN-λ2/3 and IL-6 remain elevated 8 months after the acute infection [Citation16]. These data highlight the potential role of the interaction between metabolic diseases and an abnormal immune response in the development of PASC.
At present, a rising amount of COVID-19 survivors keep reporting the presence of PASC, and it is not clear whether these symptoms will resolve over time or we will be facing a novel chronic disease [Citation5]. With this unanswered question, many recent studies have focussed on the evaluation of the persistence of PASC beyond 6 months after the acute infection, however, the distinctive immunological features that predict the persistence of PASC after this time have not been studied. In fact, the larger study addressing biomarkers of PASC was limited to 3 months of follow-up [Citation17]. This work aims to assess the association between baseline clinical and immunological features with the persistence of PASC at six months of follow-up. This information not only will contribute to the understanding of the immunopathogenesis of PASC, but also will be useful for the identification of biomarkers of the disease, to develop new technologies for its prevention, diagnostic and therapeutic approach.
Material and methods
An observational cohort study of 103 mild to critical patients with SARS-CoV-2 infection confirmed by polymerase chain reaction (PCR) test from a nasopharyngeal swab was conducted between August 2020 and August 2021 at the Unidad Temporal, a reference care centre for patients with COVID-19 in Mexico.
The baseline evaluation corresponded to the patient’s first visit to the Unidad Temporal. At this time-point, we performed a complete clinical evaluation, and we registered the laboratory and radiologic features. Disease severity was classified as mild/moderate if patients had fever, upper respiratory infection symptoms, with or without pneumonia; severe if they had respiratory failure, respiratory rate ≥30 breaths per minute, oxygen saturation at rest ≤93%, or PaO2/FiO2 ≤300 mmHg and critical if there was requirement of invasive mechanical ventilation, shock, or multiple organ failure [Citation18].
All included subjects were clinically assessed by a general physician to address the presence of long-lasting PASC symptoms using a standardised questionnaire that was validated in our previous cohort study [Citation14]. The signs and symptoms were evaluated one, three and six months after the acute infection. None of the patients had a previous diagnosis of myalgic encephalomyelitis, chronic fatigue syndrome or fibromyalgia. A blood sample was drawn at baseline to isolate peripheral blood mononuclear cells (PBMCs); serum samples were obtained at baseline and at the previously mentioned follow-up visits. All sera were stored at −80 °C until further analyses. Immunophenotyping of PBMCs was performed by multiparametric flow cytometry and serum samples were assessed for chemokines/cytokines, circulating NETs, TRIM63 levels, anti-cellular (AC) antibodies, and anti-SARS-CoV-2 IgG antibodies as described below. All patient samples used in this study were processed by investigators who were blinded to the presence or absence of PASC at 6 months. The primary outcome of the present study was the persistence of PASC at 6 months, defined as the presence of fatigue and at least two other symptoms that continued 6 months after the onset of SARS-CoV-2 infection in ambulatory patients or hospital discharge in hospitalised subjects [Citation9]. This a particularly important outcome since it is known that patients with 3 or more symptoms have a negative impact in quality of life [Citation19]. Full data from the 3 months follow-up were reported in a previous report by our group [Citation14]. Herein, we depict all the data from the 6 months follow-up.
The present study was conducted according to the ethical principles outlined in the Declaration of Helsinki. All participants granted written informed consent prior to their inclusion in the study, which received approval by the Institutional Ethics and Research committee from the Instituto Nacional de Ciencias Médicas y Nutrición Salvador Zubirán (reference: 3341).
Immunophenotyping of PBMCs
PBMCs were separated from peripheral blood by density gradient with Ficoll-Paque (GE Healthcare Life Sciences, Chicago, IL, USA) and centrifugation. Afterwards, cells were washed twice with phosphate buffered saline (PBS) and cell viability was addressed with Zombie aqua (Biolegend, San Diego, CA, USA). The cells were then washed with PBS and incubated at room temperature for 30 min with the FcX blocker (Biolegend, San Diego, CA, USA) and different combinations of fluorochrome-coupled antibodies: CD19-Pacific Blue, CD3-APC/Fire-750, CD4-Alexa Fluor 488, CD8-PE/Dazzle-594, CD25-BV421, CD45RA-PE/Cy7, CD45RO-PerCP, CD62L-PE, CD127-BV650, CCR7-Alexa Fluor 700, PD-1-APC, CD57-BV785, CD73-BV711, CD21-APC/Fire-750, CD24-PerCP, CD11c-PE/Dazzle-594, IgM-PE, CD27-APC, CD38-Alexa Fluor 488, IgD-Alexa Fluor 700, and CD138-BV605 (all from Biolegend, San Diego, CA, USA). The following lymphocyte subsets were characterised as follows: CD4+ T cells (CD3+CD4+), CD8+ T cells (CD3+CD8+), naïve T cells (CD3+CD4+ or CD8+CD45RA+CD45RO-), memory T cells (CD3+CD4+ or CD8+CD45RA-CD45RO+), central memory T cells (CD3+CD4+ or CD8+CD45RA-CD45RO+CD62L+ CCR7+), effector memory T cells (CD3+CD4+ or CD8+CD45RA-CD45RO+CD62L-CCR7-), exhausted T cells (CD3+CD4+CD8+PD-1+/hi), senescent T cells (CD3+CD4+ CD8+CD57+), anergic T cells (CD3+CD4+CD8+CD73+), Th1 (CD4+IFN-γ+), Th2 (CD4+IL-4+), Th17 (CD4+IL-17+), Tc1 (CD8+IFNγ+), Tc2 (CD8+IL-4+), Tc17 (CD8+IL-17+), regulatory T cells (CD4+CD25hiCD127lo/-) , total B cells (CD3-CD19+), CD21- transitional B cells (CD19+CD27- CD38hiCD24hiCD21-/lo), CD21+ transitional B cells (CD19+CD27-CD38hiCD24loCD21+), resting naïve B cells (CD19+CD27-IgD+CD38-CD24-CD11c-), activated naïve B cells (CD19+CD27-IgD+CD38-CD24-CD11c+), mature B cells (CD19+CD27-CD24-/lo), plasmablasts (CD19+CD27hi CD38hi), unswitched classical memory B cells (CD19+ CD27+IgD+), switched classical memory B cells (CD19+ CD27+IgD-), non-classical CD27-IgD- memory B cells (CD19+CD38-/loCD24+CD27-IgD-), and non-classical CD27-IgD+ memory B cells (CD19+CD38-/loCD24+CD27-IgD+).
For the assessment of the T helper and cytotoxic T cell subsets, PBMCs were stimulated with phorbol 12-myristate 13-acetate (PMA) (50 ng/mL), ionomycin (1 µg/mL), and monensin (4 µL/6 mL) for 5 h at 37 °C. The cells were fixed and permeabilized using the Cytofix/Cytoperm fixation/permeabilization kit (BD Biosciences, Franklin Lake, NJ, USA) according to the manufacturer instructions and the following intracytoplasmic cytokines were detected: IFN-γ-APC, IL-4-PE, and IL-17-BV421 (all from Biolegend, San Diego, CA, USA). All samples were acquired using a 4-laser LSR Fortessa flow cytometer (BD Biosciences).
Proportions of PBMC subsets were expressed as absolute numbers according to the number of lymphocytes (T and B cells) in the complete blood count drawn at the baseline visit. The samples were analysed using FlowJo v10.7 software (BD Biosciences).
Measurement of cytokine/chemokine profiles
The serum concentration of 29 cytokines and chemokines were measured using the MILLIPLEX Multi-Analyte Profiling Human Cytokine/Chemokine Magnetic Bead Panel 29-plex kit (EMD Millipore, Darmstadt, Germany) and a 2-laser Bio-Plex 200 suspension array system coupled to a Bio-Plex Pro Wash Station (Bio-Rad, Hercules, CA, USA) and according to the provider instructions. Bead-fluorescence intensity readings for all the samples and standards were converted to the corresponding analyte concentrations using the Bio-Plex Manager software v6.2 (Bio-Rad).
The following analytes were measured at baseline and at the time of the development of persistent post-COVID-19 syndrome: interleukin 1-α (IL-1α), IL-1β, IL-1RA, IL-2, IL-3, IL-4, IL-5, IL-6, IL-7, IL-8, IL-10, IL-12p40, IL-12p70, IL-13, IL-15, IL-17A, interferon α-2 (IFNα2), IFNγ, tumour necrosis factor-α (TNF-α), TNF-β, monocyte chemoattractant protein 1 (MCP-1)/CCL2, macrophage inflammatory protein 1-α (MIP-1α)/CCL3, macrophage inflammatory protein-1β (MIP-1β)/CCL4, IFNγ-induced protein (IP-10)/CXCL10, eotaxin-1/CCL11, epidermal growth factor (EGF), granulocyte colony-stimulating factor (G-CSF), granulocyte-macrophage colony-stimulating factor (GM-CSF), and vascular endothelial growth factor (VEGF).
Assessment of peripheral NETs
At baseline, the serum NETs were assessed using a neutrophil elastase ELISA as previously described [Citation20]. Briefly, high binding 96 well plates were coated with mouse anti-human neutrophil elastase (Calbiochem, Darmstadt, Germany) diluted 1:2000 in coating buffer from the cell death detection ELISA kit (Roche, Basil, Switzerland) and incubated at 4 °C, overnight. Plates were washed and then blocked with 1% bovine serum albumin (BSA) in PBS for 6 h at room temperature. Serum samples (1:10 in 1% BSA) were added to the plates and incubated at 4 °C, overnight. The following day, the plates were washed and then incubated with the anti-human DNA-POD antibody (1:10) from the cell death detection ELISA kit (Roche) in incubation buffer, for 1 h at room temperature. Afterwards, 5 washes, TMB substrate was added to each well (Thermofisher Scientific, Walthem, MA, USA), and the reaction was finished by adding stop solution. The plates were read at 450 nm and the optic density index was calculated as previously described [Citation20] in a Tecan Sunrise-RC/ST Evolyzer Plate Reader.
Serum levels of the muscle atrophy marker TRIM63
The serum levels of ubiquitin ligase TRIM63 were measured at baseline using a TRIM63 commercial ELISA kit according to the provider instructions (MyBioSource, San Diego, CA, USA).
Measurement of anti-SARS-CoV-2 IgG antibodies
Antibodies against the spike S1 domain of SARS-CoV-2 (anti-SARS-CoV-2 IgG) were measured using an anti-SARS-CoV-2 ELISA IgG Kit (EUROIMMUN; Lübeck, Germany) according to manufacturer instructions. The plates were processed using a DSX System (DYNEX Technologies, Chantilly, VA, USA). Cut-off values (<0.51 AU at the 99th percentile) were calculated using serum samples of 100 matched subjects that were collected between 2017 and 2018 from our healthy donor bank. Using this cut-off, we observed a sensitivity of 98.2% and a specificity of 98.9% (AUC 0.99) measured two weeks after COVID-19 symptoms onset.
Assessment of AC antibodies
Indirect immunofluorescence using the NOVA Lite Hep-2 ANA kit was performed at baseline to detect AC/antinuclear IgG antibodies according to the provider instructions (INOVA Diagnostics, San Diego, CA, USA) and with the HEp-2 cell line as substrate. Serum samples were tested at a 1:40 dilution using a Bee Line System (HTZ, East Grinstead, West Sussex, UK). Three experts assessed all samples and the results were discussed and registered accordingly (AutoCyte Image Titre software; Burlington, NC, USA).
Statistical analysis
To address differences between quantitative variables that were expressed as medians and interquartile ranges (IQR), we employed the Kruskal-Wallis and Mann-Whitney U tests, and for binomial variables we performed a G-test. Missing values were predicted using a random forest trained algorithm on the observed values of a data matrix. To address the potential explanatory variables for the persistent PASC, a logistic regression analysis was performed. After eliminating the highly correlated variables, we selected those with a p-value <.1 for the multivariate analysis and select the best supported multivariate model using the minimum Akaike information criteria (AIC).
The cut-off point for each explanatory variable was calculated using the Criterion based on maximising the Diagnostic Odds Ratio (OR) method [Citation21] and the AUC and likelihood ratio were calculated using receiving operator curves (ROC). As previously described, to construct a preliminary explanatory model to assess the features associated with persistence of PASC at 6 months, a score was assigned according to the OR that was proportional to the OR magnitude (i.e. highest score for the highest OR). The internal calibration and performance of the model were assessed using the following parameters: area under the curve (AUC, C-statistic), Spearman’s ρ, Somers’ D, R2, and optimism-corrected overfitting. The accuracy parameters (sensitivity, specificity, positive predictive value, negative predictive value, positive likelihood ratio, and negative predictive value) were evaluated by cross-validation and the goodness of fit of the model was assessed using the Lipsitz test [Citation22]. The calibration plot and discrimination indexes were created by bootstrapping 1000 samples of the original data. All statistical analyses were performed using R project software (version 4.0.3). This model was developed and reported according to the TRIPOD statement [Citation23].
Results
One patient did not have the complete clinical data 6 months after recruitment and was not included in the final analysis. There were no other missing data in the variables used for the evaluation of the main outcome and all patients completed the follow-up. The 102 subjects included in this cohort had a median (IQR) of age of 50.5 (41–58.2) years, and 56 (54.9%) were women. The most frequent comorbidities were obesity 45 (44.1%), hypertension 28 (24.4%), and type 2 diabetes 24 (23.5%). At recruitment, 45 (44.1%) had mild/moderate COVID-19, 30 (29.4%) were severe, and 27 (26.4%) were critical. During follow-up, 13 (12.7%) patients fulfilled the definition of persistent PASC at 6 months. There were not statistically significant differences in the baseline clinical and laboratory features in patients with and without the diagnosis of persistent PASC, other than a higher prevalence of cardiovascular disease in patients who did not develop persistent PASC at 6 months in comparison to those who did. The clinical features encompassing the PASC spectrum of symptoms at different time-points are described in .
Table 1. Clinical features of included patients during follow-up.
In comparison to patients without the primary outcome, there was not a statistically significant difference in the disease severity (p = .256), vaccination status (p = .628), the completion of the vaccination scheme (p = .968) or the type of vaccine (p = .596). Most of the patients with persistent PASC had the history of post-COVID-19 syndrome at 3 months after SARS-CoV-2 infection (10 (76–9%) vs 38 (42.6%), p = .044). Also, there was a trend towards a higher frequency of obesity (9 (69.2%) vs 36 (40.4%), p = .098) in these patients. Obesity was not related to the presence of fatigue in this cohort (p > 0.05). The baseline differential profile of serum cytokines and chemokines in patients with and without persistent post-COVID-19 syndrome is depicted in Table S1. Patients who developed the primary outcome had increased serum levels of IL-1α (6.21 pg/mL (4.96–16.34) vs 2.21 pg/mL (0.55–8.17), p = .011), G-CSF (55.08 pg/mL (30.73–84.01) vs 14.68 (14.68–44.38), p = .006) and IP-10 (2309.40 pg/mL (640.00–3447.90) vs 780.2 pg/mL (402.9–1493.0), p = .037). There was a trend towards an increased serum concentration of IL-1β (1.64 pg/mL (0.98–1.92) vs 2.21 (0.98 pg/mL (0.52–1.45), p = .062), IL-6 (15.96 pg/mL (2.48–26.28) vs 4.25 pg/mL (1.43–17.84), p = .087), and IFN-γ (12.28 pg/mL (4.49–33.67) vs 3.82 pg/mL (1.99–11.09), p = .051)
As shown in Table S2 and S3, patients with persistent PASC did not show a baseline differential amount of B and T cell subsets in peripheral blood; nonetheless, patients who fulfilled the definition of persistent PASC had an increased expression of CD57 in CD8+ T cells (42714 arbitrary units (AU) (36009–51936) vs 28506 (20196–36334), p = .003) six months after the acute infection.
There was not an association between the persistence of PASC and the baseline amount of NETs (p = .460), TRIM63 (p = .729), and anti-cellular antibodies (p = .204) nor with the titres of anti-SARS-CoV-2 antibodies at baseline and one month after COVID-19 diagnosis. Nonetheless, patients with persistent PASC had higher titres of anti-SARS-CoV-2 IgGs three months after the COVID-19 onset (Table S4).
Based on the previous data, we aimed to construct a preliminary explanatory model to address the clinical and immunological features associated with persistent PASC at 6 months after SARS-CoV-2 infection. In the univariate analysis, the variables associated with the diagnosis of persistent PASC were the serum levels of G-CSF (OR 1.01, 95% CI 1.00–1.02), p = .033), MIP-1α (OR 1.13, 95% CI 1.01–1.27), p = .020), IP-10 (OR 1.00, 95% CI 1.00–1.00), p = .009), IL-6 (OR 1.03, 95% CI 0.99–1.03), p = .045), the expression of CD57 in CD8+ T cells (OR 1.00, 95% CI 1.00–1.00), p = .006), the titres of anti-SARS-CoV-2 IgG one month (OR 1.44, 95% CI 1.03–1.44), p = .078) and three months after the COVID-19 onset (OR 1.45, 95% CI 1.06–2.19), p = .040). The results of the multivariate analysis are shown on and were used to construct a model to address the main features of persistent post-COVID-19 syndrome assigning a score according to the OR of each feature as shown in Scheme 1.
Scheme 1. Variables that predispose to the development of persistent post-COVID-19 syndrome. (A) The expression of CD57 in CD8+ T cells. (B) Obesity. (C) History of acute-Post-COVID-19 syndrome. (D,E) Increased serum levels of IP-10 and MIP-1α.
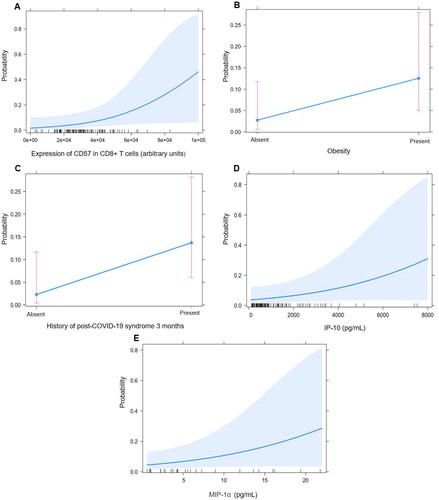
Table 2. Variables associated with persistent PASC at 6 months.
With a cut-off point of 12, the model showed a sensitivity of 97.7%, specificity of 53.8%, positive predictive value of 93.5% and a negative predictive value of 77.7%. After correcting for optimism, the model showed a good predictive capacity as shown in and Scheme 2.
Scheme 2. Statistical Model of persistent post-COVID-19 syndrome. (A) Area under the curve (AUC) with 95% CI using a cut-off point >_12. (B) Calibration plot showing a good capacity of the model for persistent post-COVID-19 syndrome features.
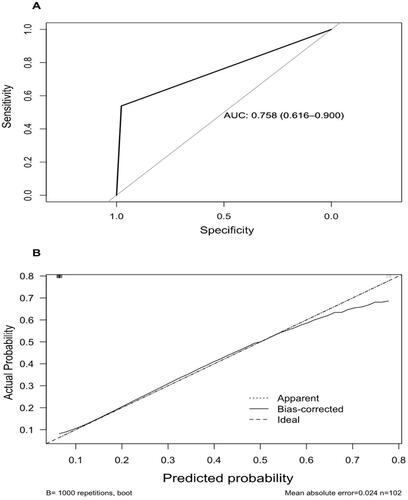
Table 3. Optimism-corrected accuracy parameters of the model.
Finally, we also decided to address the serum cytokine and chemokine 6 months after SARS-CoV-2 infection. There was not a statistically significant difference in these parameters among patients with or without PASC diagnosis at this time-point (Table S5).
Discussion
The main findings of our study are that the history of post COVID-19 syndrome, obesity, and higher baseline serum concentration of MIP-1α/CCL3, IP-10/CXCL10 and CD57 expression in CD8+ T cells in peripheral blood are important risk factors for the persistence of PASC at 6 months. To our knowledge, this is the first study to address the clinical and immunological features associated to the persistence of PASC at six months of follow-up. This is especially relevant for the identification of patients at risk of this outcome, who will not spontaneously recover from the COVID-19 sequelae and may benefit from early treatment.
Due to its high prevalence, the PASC will become an important public health problem in the short term. A meta-analysis of 40 studies with 10945 patients reported that 63.87% (95% CI 53.67–74.09%) of patients still report features of PASC after 6 months [Citation6]. The most frequent symptoms of this entity are dyspnoea with exertion, fatigue, muscle weakness, anxiety, sleep disorders, impaired concentration, chest tightness, depression [Citation6], post exertional malaise and cognitive dysfunction [Citation24]. Indeed, the symptoms of PASC overlap with those of myalgic encephalomyelitis/chronic fatigue syndrome [Citation24] however, patients with PASC usually report a shorter disease duration, and have a higher amount of symptoms [Citation25]. In a meta-analysis of 72 studies, it was shown that the PASC persist between 5–8 months after COVID-19 and most symptoms decrease after 9 months, except for the fatigue, arthralgia, anosmia, ageusia, and dizziness [Citation26]. The dyspnoea can persist in 13.1% of patients after 1 year [Citation26]. We found that 12.7% of patients report 3 or more symptoms of PASC at 6 months of follow-up. Nonetheless, due to the lack of diagnostic criteria and a standard way to assess the symptoms of PASC, a comparison of the prevalence among the different cohorts cannot be done. Indeed, previous studies have shown that 6 months after the acute SARS-CoV-2 infection, 22.9%, 10.8% and 6.5% of patients report at least 1, 2, and 3 symptoms respectively [Citation27], which is similar to our results.
Different risk factors for PASC have been described, including the female sex [Citation7,Citation24,Citation28], older age, the presence of comorbidities like diabetes, overweight and obesity, the length of hospitalisation, the diagnosis of severe COVID-19 (7), the number of symptoms during the acute infection, and a robust and persistent immune humoral response [Citation24]. According to previous studies, we did not find a difference in the vaccination status among patients who developed persistent PASC [Citation29]. Specifically at 6 months, the features associated with PASC were the female sex, a higher amount of acute symptoms, admission to the intensive care unit, and higher titres of anti-SARS-CoV-2 antibodies [Citation27].
Most of the current literature shows that the intensity of the inflammatory response during the acute infection may be a more important predictor of adverse outcomes in hospitalised patients with COVID-19. Also, an hyperinflammatory anti-viral response at baseline may be a key driver of the persistence of PASC [Citation30]. Although the pathogenesis of PASC is unknown, the direct viral damage, autoimmunity with molecular mimicry, the baseline inflammatory response and the sequelae of the stay in the intensive care unit are proposed mechanisms for the development of this entity [Citation2]. Indeed, prospective studies have demonstrated that patients who will develop PASC have a higher serum concentration of TNF-α and IP-10 in their early recovery, which is according to our results. Also, higher levels of IL-1β and IL-6 have been found to be predictors of PASC [Citation29]. In our study, we found a trend towards higher levels of IL-1β and IL-6 in patients with PASC at 6 months of follow-up but the difference was not statistically significant, probably due to our limited sample size. Furthermore, the role of IL-1α which we found elevated in patients who developed persistent PASC, is unclear and not explored. We found that the baseline serum concentration of MIP-1α and IP-10 was related to the persistence of PASC after 6 months of follow-up. IP-10 is a predictor of severe disease in COVID-19 [Citation31]. In addition to its chemoattractant role, IP-10 has been considered as an inducer of neuronal apoptosis in HIV encephalitis models [Citation32] and has been linked to the subsequent onset of neurological symptoms in this disease, which may contribute to the neurocognitive symptoms of PASC. Similar to our results, serum IP-10 levels have been related to fatigue in post viral chronic fatigue syndrome [Citation33]. PASC may be part of the spectrum of post-viral syndromes, in which after the acute infection a small reservoir of the virus has been observed, which activates the immune response and metabolic signalling cascades in mast and glial cells, maintaining an amplified production of TNF-α and IL-6, which plays an important part in the neurological symptomatology observed in the PASC, particularly in patients with obesity [Citation24], which we identified as another risk factor for this entity.
According to our results, one of the main sources of increased chemokines in persistent post-COVID-19 syndrome seems to be the adipose tissue, which is in agreement with Zickler et al. who found the persistence of SARS CoV-2 in human adipose tissue in overweight and obese patients [Citation34]. Indeed, obesity has been reported as a key metabolic dysfunction in the development of PASC [Citation35]. Obesity is a risk factor for long term morbidity and mortality for other viral infections that share similar traits with COVID-19 (35). Our data supports the hypothesis that the IL-6, IP-10 and MIP-1α mediated inflammation in obesity may not only promote the organ dysfunction in COVID-19, but also may be implicated in the development of PASC.
Another recent theory sustains that the PASC are the result of a defective clearance of SARS-CoV-2 [Citation36], leading to a prolonged and persistent antiviral immune response [Citation4]. According to this hypothesis, it is known that after an infection with intense systemic inflammation, there is a prolonged counterregulatory anti-inflammatory response leading to immunosuppression and a higher risk of viral reactivation [Citation37]. The persistent inflammatory response may promote an exhausted or senescent phenotype in the adaptive immune response. In fact, one previous study showed that patients with post-COVID-19 conditions have a decreased amount of CD8+ effector memory T cells and higher proportions of CD4+ T lymphocytes, CD8+ central memory T cells and increased amount of PD-1 in peripheral blood many months after the acute infection [Citation24]. We also found senescent CD8+ T cells as key predictors of persistent post-COVID-19 syndrome. The CD8+ CD57+ phenotype has been previously described in chronic viral infections as a consequence of a continuous cell proliferation, with premature immune-senescence [Citation38]. Furthermore, viral infections have been reported as drivers of premature immunological ageing in young adults, with the consequent increased risk of morbidity, disability and frailty [Citation39]. To address that a persistent cytotoxic activity driven by CD8+ CD57+ correlates with the PASC presentation, deeper metabolic characterisation of this cellular subgroup is needed, as an immunomodulatory CD8+ CD57+ phenotype has also been described [Citation38].
The SARS-CoV-2 persistence in CNS and adipose tissue [Citation34] has been proposed as a potential mechanism responsible for the development of PASC [Citation40], highlighting the importance of fat tissue in obesity as a unique SARS-CoV-2 reservoir that induces CD8+ T cells senescence as reflected in our model. Changes in the adaptive immune response due to the presence of the antigenic reservoir, mainly in obese patients, also has consequences in the humoral response that could be represented by the persistence of anti-SARS-CoV-2 IgG for more than 12 months, which is related to the presence of post-COVID-19 syndrome in ours and previous reports [Citation41]. In patients with severe COVID-19, there is potent inflammatory response mediated by anti-SARS-CoV2 IgG, which activate Fc receptors, fix complement, and promote the production of MCP-1 and IL-8 with the subsequent recruitment and accumulation of proinflammatory monocytes and macrophages [Citation42]. In the humoral response triggered by SARS-CoV-2, a lower amount of neutralising and specific antibodies has been reported in obese versus lean patients (p = .002) [Citation43]. Besides, a molecular mimicry between SARS-CoV-2 and gut, kidney, lung and heart self-antigens has been described, which may be a pathogenic mechanism of post-COVID-19 syndrome [Citation44].
This study has several limitations. Our cohort was composed of a limited number of Latin American patients and the possible occurrence of reinfection with the new variants during follow up, which could modify the course of the disease was not addressed. Moreover, the prolonged time between the measurement of most immunological features and the primary outcome along with the small number of patients with persistent PASC after 6 months of follow-up may have precluded us to find statistically significant differences and associations. The main strength of our study is the identification of the proinflammatory, and senescent immune response related to obesity as a potential pathogenic factor in the persistence of PASC at 6 months of follow-up. Also, the identification of the mentioned parameters at baseline may allow the prompt identification of patients at risk. Our data provides preliminary findings that lack a predictive or diagnostic capacity due to the limited amount of patients who developed the primary outcome. Nonetheless, these data could be useful to to address PASC prevalence at 6 months and for sample size calculations and to guide on the inclusion of key immunological features in order to improve methodological approaches for future studies to reduce the PASC disease burden. Finally, we acknowledge that the interpretation of our findings is based on the current literature regarding PASC and other post-viral syndromes. Therefore, our study provides only preliminary findings regarding the potential immunopathogenic role of this immunologic features on PASC, which should be confirmed and validated in larger cohorts and using experimental approaches.
In summary, we provide a clinical and immunological characterisation of patients with persistent PASC at 6 months follow-up, which suggest an important relationship between a pro-inflammatory state mediated through metabolic pathways related to obesity and increased cellular senescence. We acknowledge our results reinforce and complement previous studies, however, much more information is needed to be able to confirm the hypotheses about the pathogenic scheme of persistent post-COVID-19 syndrome.
Author contributions
JT-R, JL-G, ASV-C, JL-L, AP-F, RT-C, MN-A, BA-C, AA-A, JLM-M, NRM-D, CN-A, MR-G, LL, SR-R, VAS-H, RC-D, GJ-V, DM-S, LAM-J, RRV-V, JAF-L, and DG-M participated in the conceptualisation of the study. JT-R, JL-G, ASV-C, AP-F, JLM-M, NRM-D, CN-A, MR-G, LL, LAM-J, LM-J, LNL-L, JAN-T, JAF-L, RRV-V, HG-R, and DG-M collaborated with the study design. Data acquisition and analysis were done by JT-R, JL-G, ASV-C, DG-M, JL-L, AP-F, RT-C, MN-A, BA-C, AA-A, JLM-M, NRM-D, CN-A, GJ-V, DM-S, LNL-L, and JAN-T. Data interpretation was done by JT-R, JL-G, ASV-C, DG-M, JL-L, AP-F, RT-C, MN-A, BA-C, AA-A, JLM-M, CN-A, MR-G, LL, SR-R, VAS-H, RC-D, GJ-V, DM-S, LAM-J, LM-J, JAF-L, RRV-V, and DG-M. JT-R, JL-G, ASV-C, DG-M, JL-L, AP-F, RT-C, MN-A, BA-C, AA-A, JLM-M, NRM-D, CN-A, MR-G, LL, SR-R, VAS-H, RC-D, GJ-V, DM-S, LAM-J, LM-J, and RRV-V wrote the original draft. All authors read and approved the final manuscript.
Supplemental Material
Download MS Word (33.9 KB)Acknowledgments
The authors acknowledge the technical support provided by the Red de Apoyo a la Investigación (RAI).
Disclosure statement
No potential conflict of interest was reported by the author(s).
Data availablity statement
All data generated or analysed during this study are included in this published article.
Additional information
Funding
References
- Nalbandian A, Sehgal K, Gupta A, et al. Post-acute COVID-19 syndrome. Nat Med. 2021;27(4):601–615.
- Munipalli B, Seim L, Dawson NL, et al. Post-acute sequelae of COVID-19 (PASC): a meta-narrative review of pathophysiology, prevalence, and management. SN Compr Clin Med. 2022;4(1):90.
- Soriano JB, Murthy S, Marshall JC, WHO Clinical Case Definition Working Group on Post-COVID-19 Condition, et al. A clinical case definition of post-COVID-19 condition by a delphi consensus. Lancet Infect Dis. 2022;22(4):e102–e7.
- Munblit D, Nicholson TR, Needham DM, et al. Studying the post-COVID-19 condition: research challenges, strategies, and importance of core outcome set development. BMC Med. 2022;20(1):50.
- Pinto MD, Downs CA, Huang Y, et al. A distinct symptom pattern emerges for COVID-19 long-haul: a nationwide study. Sci Rep. 2022;12(1):15905.
- Ma Y, Deng J, Liu Q, et al. Long-Term consequences of COVID-19 at 6 months and above: a systematic review and Meta-Analysis. Int J Environ Res Public Health. 2022;19(11):6865.
- Ceban F, Ling S, Lui LMW, et al. Fatigue and cognitive impairment in Post-COVID-19 syndrome: a systematic review and meta-analysis. Brain Behav Immun. 2022;101:93–135.
- Huang Y, Pinto MD, Borelli JL, et al. COVID symptoms, symptom clusters, and predictors for becoming a Long-Hauler looking for clarity in the haze of the pandemic. Clin Nurs Res. 2022;31(8):1390–1398.
- Amenta EM, Spallone A, Rodriguez-Barradas MC, et al. Postacute COVID-19: an overview and approach to classification. Open Forum Infect Dis. 2020;7(12):ofaa509.
- Zhu Y, Chen X, Liu X. NETosis and neutrophil extracellular traps in COVID-19: immunothrombosis and Beyond. Front Immunol. 2022;13:838011.
- Seeßle J, Waterboer T, Hippchen T, et al. Persistent symptoms in adult patients 1 year after coronavirus disease 2019 (COVID-19): a prospective cohort study. Clin Infect Dis. 2022;74(7):1191–1198.
- Son K, Jamil R, Chowdhury A, et al. Circulating anti-nuclear autoantibodies in COVID-19 survivors predict long-COVID symptoms. Eur Respir J. 2022;2200970. DOI:10.1183/13993003.00970-2022
- Torres-Ruiz J, Pérez-Fragoso A, Maravillas-Montero JL, et al. Redefining COVID-19 severity and prognosis: the role of clinical and immunobiotypes. Front Immunol. 2021;12:689966.
- Torres‐Ruiz J, Lomelín‐Gascón J, Lira‐Luna J, et al. FANSY POSTCOV: a composite clinical immunological predictive index for post‐COVID‐19 syndrome unveils distinctive features in a cohort study of mild to critical patients. Clinical and Translational Med. 2021;11(11):e623.
- De Biasi S, Meschiari M, Gibellini L, et al. Marked T cell activation, senescence, exhaustion and skewing towards TH17 in patients with COVID-19 pneumonia. Nat Commun. 2020;11(1):3434.
- Phetsouphanh C, Darley DR, Wilson DB, et al. Immunological dysfunction persists for 8 months following initial mild-to-moderate SARS-CoV-2 infection. Nat Immunol. 2022;23(2):210–216.
- Su Y, Yuan D, Chen DG, ISB-Swedish COVID-19 Biobanking Unit, et al. Multiple early factors anticipate post-acute COVID-19 sequelae. Cell. 2022;185(5):881–95 e20.
- Liu W, Tao ZW, Wang L, et al. Analysis of factors associated with disease outcomes in hospitalized patients with 2019 novel coronavirus disease. Chin Med J (Engl). 2020;133(9):1032–1038.
- Demko ZO, Yu T, Mullapudi SK, et al. Post-acute sequelae of SARS-CoV-2 (PASC) impact quality of life at 6, 12 and 18 months post-infection. medRxiv. 2022.
- Lood C, Blanco LP, Purmalek MM, et al. Neutrophil extracellular traps enriched in oxidized mitochondrial DNA are interferogenic and contribute to lupus-like disease. Nat Med. 2016;22(2):146–153.
- Böhning D, Holling H, Patilea V. A limitation of the diagnostic-odds ratio in determining an optimal cut-off value for a continuous diagnostic test. Stat Methods Med Res. 2011;20(5):541–550.
- Lipsitz SR, Buoncristiani JF. A robust goodness-of-fit test statistic with application to ordinal regression models. Stat Med. 1994;13(2):143–152.
- Zamanipoor Najafabadi AH, Ramspek CL, Dekker FW, et al. TRIPOD statement: a preliminary pre-post analysis of reporting and methods of prediction models. BMJ Open. 2020;10(9):e041537.
- Castanares-Zapatero D, Chalon P, Kohn L, et al. Pathophysiology and mechanism of long COVID: a comprehensive review. Ann Med. 2022;54(1):1473–1487.
- Jason LA, Islam M, Conroy K, et al. COVID-19 symptoms over time: comparing Long-Haulers to ME/CFS. Fatigue. 2021;9(2):59–68.
- Yang T, Yan MZ, Li X, et al. Sequelae of COVID-19 among previously hospitalized patients up to 1 year after discharge: a systematic review and meta-analysis. Infection. 2022;50(5):1067–1109.
- Peghin M, Palese A, Venturini M, et al. Post-COVID-19 symptoms 6 months after acute infection among hospitalized and non-hospitalized patients. Clin Microbiol Infect. 2021;27(10):1507–1513.
- Maglietta G, Diodati F, Puntoni M, et al. Prognostic factors for Post-COVID-19 syndrome: a systematic review and Meta-Analysis. J Clin Med. 2022;(6):11.
- Schultheiß C, Willscher E, Paschold L, et al. The IL-1beta, IL-6, and TNF cytokine triad is associated with post-acute sequelae of COVID-19. Cell Rep Med. 2022;3(6):100663.
- Peluso MJ, Lu S, Tang AF, et al. Markers of immune activation and inflammation in individuals with postacute sequelae of severe acute respiratory syndrome coronavirus 2 infection. J Infect Dis. 2021;224(11):1839–1848.
- Schulte-Schrepping J, Reusch N, Paclik D, Deutsche COVID-19 OMICS Initiative (DeCOI), et al. Severe COVID-19 is marked by a dysregulated myeloid cell compartment. Cell. 2020;182(6):1419–1440.e23.
- Sui Y, Potula R, Dhillon N, et al. Neuronal apoptosis is mediated by CXCL10 overexpression in simian human immunodeficiency virus encephalitis. Am J Pathol. 2004;164(5):1557–1566.
- Montoya JG, Holmes TH, Anderson JN, et al. Cytokine signature associated with disease severity in chronic fatigue syndrome patients. Proc Natl Acad Sci U S A. 2017;114(34):E7150–E7158.
- Zickler M, Stanelle-Bertram S, Ehret S, et al. Replication of SARS-CoV-2 in adipose tissue determines organ and systemic lipid metabolism in hamsters and humans. Cell Metab. 2022;34(1):1–2.
- Smith M, Honce R, Schultz-Cherry S. Metabolic syndrome and viral pathogenesis: lessons from influenza and coronaviruses. J Virol. 2020;94(18):e00665–20.
- Mehandru S, Merad M. Pathological sequelae of long-haul COVID. Nat Immunol. 2022;23(2):194–202.
- Oronsky B, Larson C, Hammond TC, et al. A review of persistent Post-COVID syndrome (PPCS). Clin Rev Allergy Immunol. 2021. DOI:10.1007/s12016-021-08848-3
- Strioga M, Pasukoniene V, Characiejus D. CD8+ CD28− and CD8+ CD57+ T cells and their role in health and disease. Immunology. 2011;134(1):17–32.
- Le Priol Y, Puthier D, Lécureuil C, et al. High cytotoxic and specific migratory potencies of senescent CD8+CD57+cells in HIV-Infected and uninfected individuals. J Immunol. 2006;177(8):5145–5154.
- Scordo KA, Richmond MM, Munro N. Post–COVID-19 syndrome: theoretical basis, identification, and management. AACN Adv Crit Care. 2021;32(2):188–194.
- Kučinskaitė-Kodzė I, Simanavičius M, Šimaitis A, et al. Persistence of SARS-CoV-2-Specific antibodies for 13 months after infection. Viruses. 2021;13(11):2313.
- Folgueira MD, Luczkowiak J, Lasala F, et al. Prolonged SARS-CoV-2 cell culture replication in respiratory samples from patients with severe COVID-19. Clin Microbiol Infect. 2021;27(6):886–891.
- Frasca D, Reidy L, Romero M, et al. The majority of SARS-CoV-2-specific antibodies in COVID-19 patients with obesity are autoimmune and not neutralizing. Int J Obes. 2022;46(2):427–432.
- Wang EY, Mao T, Klein J, Yale IMPACT Team, et al. Diverse functional autoantibodies in patients with COVID-19. Nature. 2021;595(7866):283–288.